Abstract
Hyperbaric oxygen (HBO) therapy is used to treat or prevent tissue necrosis in patients undergoing irradiation. Many such patients require reconstructive surgery, but little is known of the effects of HBO on bone vascularization and regeneration. In this study, copolymer poly( l -lactide-co-1,5-dioxepan-2-one) (poly(LLA-co-DXO)) scaffolds were implanted into critical-sized calvarial defects in Wistar rats. The animals were randomly allotted to hyperbaric or normobaric oxygen groups. The treatment group received five sessions weekly for 90 min at increased atmospheric pressure, for up to 4 weeks. Samples were retrieved at weeks 2 and 8, i.e. after a total of 10 and 20 sessions, respectively. The samples were analyzed by real-time reverse transcriptase polymerase chain reaction (RT-PCR) and histology at week 2, and radiographically and histologically at week 8. At week 2, defects treated with HBO exhibited greater numbers of cells positive for the endothelial marker CD31, up-regulated gene expression of osteogenic markers, and down-regulated expression of pro-inflammatory cytokines. At week 8, radiographic examination revealed that calvarial defects subjected to HBO exhibited a higher percentage of radiopacities than normobaric controls, and histological examination disclosed enhanced bone healing. These results confirmed that HBO treatment was effective in stimulating vascularization and bone formation in rat calvarial defects.
Reconstruction of bone defects through autologous grafting is associated with donor site morbidity and is not feasible in all patients. As a basis for translational research in oral and maxillofacial bone reconstruction, a series of studies using stem cells in combination with biodegradable synthetic scaffolding materials has been undertaken. Two major challenges in this field of research are inadequate vascularization and the dependence of tissue regeneration on adequate recruitment of endogenous cells.
Hyperbaric oxygen (HBO) therapy, where the patient breathes 100% oxygen at increased atmospheric pressure, leads to both arterial and tissue oxygen tension. The production of reactive oxygen species activates various signalling molecules and has been attributed a key role in the therapeutic effects of HBO. Stimulated homing of vasculogenic stem cells has been found in skin wounds, and a review by Goldman concluded that there is good evidence for using HBO to promote wound healing. Nilsson et al. found an effect on bone regeneration in a rabbit bone harvest chamber, and more recently the healing of critical-sized calvarial defects in rabbits were shown to increase after HBO treatment. Sirin et al. investigated healing of rat tibia defects filled with β-tricalcium phosphate (β-TCP) and calcium phosphate-coated bovine bone, where only the former showed improved bone healing compared to control defects. Following HBO, bone defects filled with biphasic calcium phosphate have also shown a more favourable bone healing response than defects filled with demineralized bone matrix.
Many patients needing major bone reconstruction have impaired local vascularization due to tumour surgery and radiation therapy, and HBO has been proposed as a post-grafting treatment modality. Sawai et al. grafted corticocancellous bone from the iliac crest to the mandible in rabbits, followed by post-operative HBO. The results suggested that the union of autogenous bone grafts was accelerated in the treatment group. Furthermore, the reduction of bone mineral content in autografts following HBO has also been found, possibly attributable to increased angiogenesis within the graft. Recent studies have shown that copolymer poly( l -lactide-co-1,5-dioxepan-2-one) (poly(LLA-co-DXO)) scaffolds are able to serve as a framework for cellular proliferation and differentiation, and the chemical versatility of the material enables degradation and mechanical properties to be tailored to meet the intended application.
HBO might be a part of the treatment regime in the rehabilitation of patients recovering from malignant disease, especially if reconstructive surgery is required. However, limited knowledge exists about the biological effects of HBO in the presence of biodegradable scaffolding materials aimed at bone tissue engineering. In the present work, cell- and material-derived osteoinductive factors were not included in order to study the isolated effects of treatment. The aim of the present study was to test the hypothesis that HBO stimulates vascular ingrowth in calvarial bone defects filled with poly(LLA-co-DXO) scaffolds, leading to mobilization of endogenous progenitor cells and improved bone healing.
Materials and methods
Scaffolds
Production of the copolymer poly(LLA-co-DXO) scaffolds has been described previously. Briefly, polymerization was performed in bulk at 110 °C for 72 h and precipitated three times in cold hexane and methanol. Scaffolds were made through the salt-leaching method whereby dissolved poly(LLA-co-DXO) was casted in a mould with NaCl at a ratio of 10:1. After repeated soaking in water, the salt-free scaffolds were vacuum-dried and sterilized with electron beam radiation.
Animal procedures
Skeletally mature 16 week-old female Wistar rats were anaesthetized with isoflurane (IsobaVet ® ; Schering-Plough, Kenilworth, NJ, USA) combined with O 2 using a custom-made mask. The scalp was shaved and disinfected. A midline incision was made through skin, temporalis muscle and periosteum and flaps were reflected laterally. A trephine burr was used under saline irrigation to create bilateral 6-mm bone defects in the parietal calvaria. The dura mater was exposed and inspected for bleeding. Supradural placement of scaffolds of the same size as the defects was carefully performed, leaving the material in contact with the bony margins of the defects. The wound was closed in two layers with resorbable sutures (vicryl rapid 4-0; Ethicon, Somerville, NJ, USA).
A topical antibiotic was applied on the sutured skin wounds to prevent post-operative infections. Postoperatively, the animals were placed in separate cages for recovery, after which they were returned to their original cages as before surgery. One rat had a partially open wound on the first postoperative day, which was re-sutured. At weeks 2 and 8, animals were euthanized with an overdose of CO 2 . At week 2, scaffolds were dissected out and halved, one for reverse transcriptase polymerase chain reaction (RT-PCR) analysis and the other for histological analysis. Samples intended for paraffin embedding were fixed in 4% paraformaldehyde (PFA) (Merck, Darmstadt, Germany), while those intended for RT-PCR and O.C.T.™ compound (Sakura Finetek, Tokyo, Japan) embedding were kept in RNALater (Invitrogen, Carlsbad, CA, USA) and frozen within 3 h. Samples retrieved at week 8 were fixed in 4% PFA.
Hyperbaric oxygen chamber
An Oxycom 250 Arc pressure chamber was used (Hypcom Oy, Tampere, Finland), with a volume of 27 l, an inner diameter of 25 cm, and inner length of 55 cm. A standard prophylactic protocol for osteoradionecrosis was applied. The chamber was filled with 100% O 2 for 15 min, and pressure was raised to 1.5 bar (2.5 atm) over a period of 10–15 min. Pressure was maintained for 90 min, and no more than four rats were treated simultaneously. To maintain >97% O 2 at all times, the chamber was flushed with pure oxygen for 5 min every 10 min. The rats were decompressed for 10–15 min. As shown in Table 1 , HBO was administered to the experimental group from the first day post-operatively and every weekday for 2 or 4 weeks: the 4 weeks of treatment were followed by an interval of 4 weeks without treatment before the animals were euthanized. Thus the animals in the experimental group that were euthanized at week 2 received a total of 10 HBO treatments, and those euthanized at week 8 received 20 treatments over the first 4 weeks.
NBO | HBO | |||
---|---|---|---|---|
Time-point (weeks) | 2 | 8 | 2 a | 8 b |
Number of exposures | 0 | 0 | 10 a | 20 b |
Exposure time (min) | 0 | 0 | 90 | 90 |
Ambient pressure (atm) | 1 | 1 | 2.5 | 2.5 |
Gas | Air | Air | O 2 | O 2 |
a HBO was administered five times weekly for 2 weeks; the animals were then euthanized.
b HBO was administered five times weekly for 4 weeks, followed by an interval of 4 weeks without treatment before the animals were euthanized at week 8.
Real-time RT-PCR
An E.Z.N.A. ® Total RNA Kit (Omega Bio-Tek, Norcross, GA, USA) was used to extract RNA from the retrieved scaffolds. A NanoDrop Spectrophotometer (ThermoScientific NanoDrop Technologies, Wilmington, DE, USA) was used to quantify RNA and determine the purity of RNA. A high capacity cDNA Archive Kit (Applied Biosystems, Carlsbad, CA, USA) was used for the reverse transcription reaction. Total RNA (1000 ng) was mixed with nuclease-free water, reverse transcriptase buffer, random primers, dNTP, and MultiScribe reverse transcriptase. The real-time RT-PCR was performed on a StepOne™ Real-Time PCR System (Applied Biosystems) under standard enzyme and cycling conditions. cDNA corresponding to 10 ng mRNA was used in each reaction, prepared in duplicate for each target gene. Taqman ® gene expression assays were used: alkaline phosphatase (ALP), bone morphogenetic protein 2 (BMP-2), bone sialoprotein (BSP), osteocalcin (OC), interleukin 1 (IL-1), interleukin 6 (IL-6), and interleukin 10 (IL-10). A comparative cycle threshold (Ct) method was used for data analysis, and β-actin served as endogenous control.
Histological evaluation
Among the samples retrieved for histology at week 2, half were fixed in 4% PFA and embedded in paraffin and the remainder were embedded in O.C.T. compound (Sakura Finetek) and maintained at −80 °C. The paraffin-embedded samples were sectioned to 8-μm thickness with a microtome (Leica Microsystems, Wetzlar, Germany), while frozen samples were cryosectioned with a Leica CM 3050S at −24 °C. Samples retrieved at week 8 were decalcified for 3 weeks with 10% ethylenediaminetetraacetic acid (EDTA) in 0.1 M Tris buffer and 7.5% polyvinylpyrrolidone (PVP) (Merck) before embedding in paraffin, sectioning, and staining with haematoxylin–eosin (HE) or Masson’s trichrome. The slides were passed through a graded alcohol and xylol series before mounting with Eukitt (O. Kindler, Freiburg, Germany). ALP staining was performed with a 2-h incubation time at room temperature with freshly made substrate solution (Sigma–Aldrich, St. Louis, MO, USA) containing 100 mM Tris–maleate buffer, 8 mg/ml naphthol AS-TR, and 2 mg/ml diazonium salt fast red violet LB. The slides were washed with distilled water and counterstained with 0.1% fast green. CD31 staining was performed with a polyclonal CD31 antibody (Santa Cruz Biotechnology, Santa Cruz, CA, USA) diluted 1:200 in phosphate-buffered saline (PBS) with 5% rabbit serum for 4 h at room temperature. The secondary antibody was a tetramethylrhodamine isothiocyanate (TRITC)-conjugated rabbit anti-goat antibody diluted 1:1000, incubated for 2 h at room temperature. The slides were mounted with Prolong ® Gold Antifade Reagent (Invitrogen) before imaging.
Radiographic evaluation
A dental X-ray machine (Gendex, Hatfield, PA, USA) was used to obtain radiographs from the retrieved calvaria at week 8, before decalcification. Exposure conditions were 10 mA, 0.08 ms, and 60 kV. NIS-Elements BR 3.07 software (Nikon, Tokyo, Japan) was used for image analysis. A common threshold was applied to all defects and the sum percentage of radiopacity was determined for each defect. Sectional images were obtained with a BrightField CT Scanner (GE Healthcare, Waukesha, WI, USA), and OsiriX DICOM Viewer version 5.0.2 was applied for image processing.
Statistical analysis
The sample size for radiographic evaluation was eight. For histology and real-time RT-PCR, the results presented are from a minimum of six parallels of treated (HBO) and non-treated (NBO) samples. For statistical evaluation, the Shapiro–Wilk test was first applied to test for normality, in which H 0 of normally distributed values could not be rejected. Next, a two-sample F -test with n − 1 degrees of freedom was performed, which also failed to reject H 0 , in this case <SPAN role=presentation tabIndex=0 id=MathJax-Element-1-Frame class=MathJax style="POSITION: relative" data-mathml='σ12=σ22′>σ21=σ22σ12=σ22
σ 1 2 = σ 2 2
. Based on this, the independent sample t -test was selected. SPSS Statistics 19.0 (IBM, Armonk, NY, USA) was applied for statistical processing and analysis, and the significance level was set to P < 0.05. The results are presented as mean ± standard deviation. A post hoc power analysis was performed specifically for the radiographic measurements using the non-central t -distribution. The effect size d for this parameter was calculated to be 1.957, and the non-centrality parameter δ was 3.916, with the critical t being 2.145 with 14 degrees of freedom (( n 1 + n 2 ) − 2). With α set to 0.05, the statistical power defined as the probability of 1 − β error was calculated to be 0.953. The respective formulas for calculating effect size and the non-centrality parameter were:
d = x ¯ 1 − x ¯ 2 σ and δ = d × n 1 n 2 n 1 + n 2 ⋅
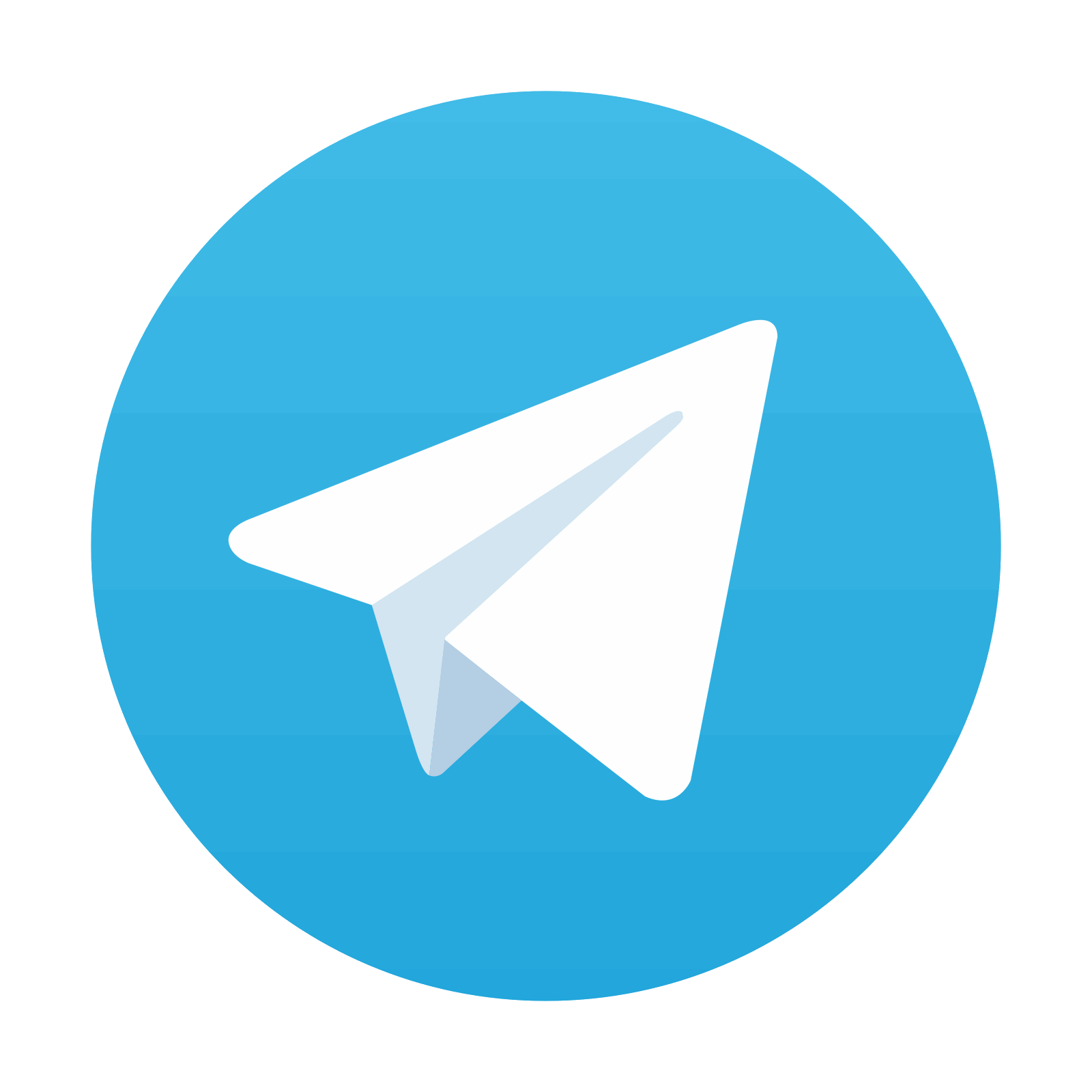
Stay updated, free dental videos. Join our Telegram channel

VIDEdental - Online dental courses
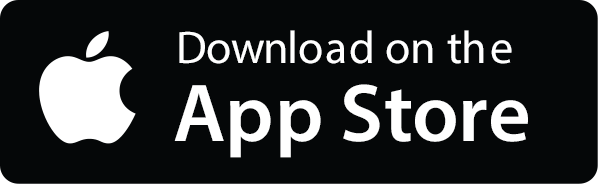
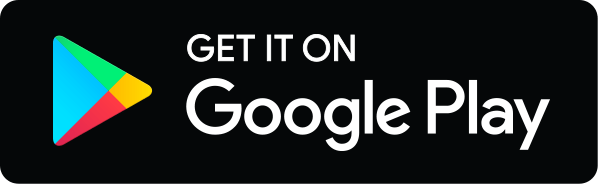