Abstract
The motivation for orthognathic surgery is to improve facial appearance and quality of life. This study aimed to validate a three-dimensional (3D) orthognathic planning programme (Maxilim) for predicting soft tissue changes following Le Fort I advancements. Cone beam computed tomography (CBCT) scans were taken before surgery (T 1 ) and at 6–12 months after surgery (T 2 ) for 13 patients. For each patient the 3D hard tissue changes between T 1 and T 2 were determined by CBCT superimposition on the cranial vault. Using Maxilim, each patient’s skeletal movements were used to generate a 3D soft tissue prediction. The actual soft tissue mesh at T 2 was compared to the predicted mesh. The face was divided into areas: nose, right and left nares, right and left paranasal regions, upper and lower lip, and chin. The absolute distance between meshes for each region was calculated. A one-sample t -test showed the distances between the meshes for all of the areas were within 3 mm ( P < 0.05), except for the upper lip which was greater than 3 mm ( P = 0.577). Using Maxilim, 3D soft tissue predictions for Le Fort I advancements were clinically satisfactory in the regions assessed, but associated with marked errors around the region of the upper lip.
It has been shown that significant facial and dental anomalies may have negative social implications for the affected individual. The main aim of orthognathic surgery is to improve facial and dental aesthetics, often with a secondary aim of improving function.
Accurate computerised prediction planning for the surgical correction of dento-facial deformities is important for several reasons: guiding the surgical procedure regarding the magnitude and direction, providing the patient with the expected outcome, and to serve as a communication tool between orthodontist, surgeon, and patient. Therefore the accuracy of prediction planning is crucial to the success of patient management and the satisfactory correction of dento-facial deformities.
At present many units rely on conventional two-dimensional (2D) prediction planning, which has several shortcomings. Firstly, the 2D prediction of the changes following orthognathic surgery is of limited accuracy due to the limited set of ratios for the soft tissue changes in response to the surgical movements of the underlying bone segments. These ratios are based on historical, varied, and questionable evidence. Secondly, prediction is limited to 2D and does not take into consideration the changes in the third dimension. Patients have also found 2D prediction planning to be of limited value due to the fact that they are unaccustomed to seeing themselves in profile, which is usually the case with most of the existing commercial packages for prediction planning of orthognathic surgery. Moreover, the method is unsuitable for the prediction of the surgical correction of facial asymmetry.
To overcome the present deficiencies, three-dimensional (3D) surgical planning programs have been developed and used to analyze and predict the outcome following orthognathic surgery. These programs are designed to allow surgical manipulation of the jaw bones and predict the overlying soft tissue response. The drawback with using these software programs is that they are time-consuming and require comprehensive training, and the novice user would find this challenging. Data on the validity of the predictions produced by these packages are also very limited.
Computer-assisted 3D virtual reality soft tissue prediction planning following orthognathic surgery has developed over the last decade. In an effort to commercialise this type of software, Mollemans et al. assessed computational strategies to model the deformation behaviour of facial soft tissues and developed Maxilim (MEDICIM, Mechelen, Belgium).
A recent study assessed the 3D prediction programe SurgiCase CMF Pro on 10 consecutive patients using standardised images from a NewTom 3G cone beam computed tomography (CBCT) machine, prior to and 6 months after surgery. The 10 patients were a mixed cohort of single jaw and double jaw surgery and genioplasties. Using 3-matic ® (Materialise), the actual postoperative facial soft tissue surface was compared with the predicted image by superimposing both of them on the forehead and eye region. This produced qualitative colour maps that showed graphically the differences between the actual postoperative soft tissue surfaces and the prediction. The study reported a median prediction error of 0.47 mm, but the range of values was very large, ranging from 0 mm to over 3 mm for two out of the 10 patients.
Therefore the aim of this study was to determine the validity of Maxilim in predicting the 3D soft tissue morphology of patients undergoing a maxillary Le Fort I advancement procedure for correction of class III skeletal patterns, taking into consideration the drawbacks of previous studies. The null hypothesis was that the mean differences between the 3D soft tissue surface mesh generated by Maxilim and the final 3D soft tissue mesh produced by surgery at predefined anatomical regions were not clinically significant. A soft tissue difference of greater than 3 mm was taken as clinically significant. This threshold value is based on a previous study that showed that a 3-mm change was defined as clinically significant in facial soft tissue measurements.
Materials and methods
Ethical approval was obtained from the local area ethics committee. Routine data were collected at two time points: (1) pre-surgery, a CBCT taken immediately prior to surgery (T 1 ), and (2) post-surgery, a CBCT taken between 6 and 12 months after surgery (T 2 ), to minimise the effect of post-operative facial swelling on the analysis.
This retrospective study used the records of 13 non-syndromic patients selected from a multidisciplinary dento-facial planning clinic. All included patients were diagnosed with a maxillary deficiency based on a comprehensive clinical and radiographic assessment and treated with a standard Le Fort I advancement procedure with minimal vertical movements and no associated mandibular surgery. For all the patients, the prominence of ANS (Anterior Nasal Spine) was reduced during surgery. A cinch stitch was used via an extra-oral approach to maintain the alar base width to the recorded pre-operative measurement. This was carried out using a 3(0) non-resorbable suture, which was threaded through a hole drilled in the remaining part of the ANS. A V–Y closure was carried out for all the cases to allow some eversion of the vermilion border. Any patients who had undergone bimaxillary surgery, previous osseous or soft tissue surgery to the face, additional segmental procedures, or required multi-part maxillary surgery were excluded from the study.
The average age of the patients was 23 years ± 8 years; five males and eight females were included in the study. The time interval between the pre- and post-operative CBCT scans ranged from 6 to 12 months. The mean anterior–posterior maxillary change following surgery was 5.5 mm ± 2.2 mm, with negligible vertical movement.
Routine CBCT scans were taken at the extended field of view option (EFOV 22 cm) with 0.4 mm voxel size resolution, which produced an overall scan time of 40 s at 120 kV. Each patient was positioned by the same experienced clinician, with each CBCT image taken by the same operator using the same machine, i-CAT ® (Imaging Sciences International, Hatfield, PA, USA).
During image capture the patients were stabilised with a high head band and their teeth kept in centric occlusion – if there was evidence of mandibular over-closure and soft tissue distortion, a wax wafer in rest position was used; lips were always kept in repose.
Data files from the CBCT machine were stored in DICOM format (Digital Imaging and Communications in Medicine). The initial 3D models for both hard and soft tissues were constructed using Maxilim 2.2.0 (MEDICIM) installed on a personal computer (Dell Optiplex 745). The models were analysed using VRMesh CAD/CAM mesh editing software (VirtualGrid, Seattle, WA, USA) installed on a personal computer (Dell XPS).
Data processing
Both pre- and post-surgical DICOM files for each patient were imported into Maxilim and a conventional 3D virtual volumetric image produced of the hard and soft tissue. The hard and soft tissue surfaces for each patient were then saved as STL surface models.
For each patient, the pre- and post-surgical hard tissue surface models were imported into VRMesh, and the post-surgical image was translated, rotated, and superimposed on the anterior cranial base and frontal bone of the cranium of the pre-surgical image. The maxilla and mandible of the post-surgical model were isolated and exported from VRMesh as an STL file into the Maxilim software. This produced the actual post-surgical position of the maxilla and mandible realigned to the frame of reference of the pre-surgical image ( Fig. 1 ).
The pre-surgical DICOM image of each patient was then imported into Maxilim. A virtual Le Fort I osteotomy was carried out on the pre-surgical model with the maxilla and mandible translated, rotated, and re-aligned to the final post-surgical position ( Fig. 2 ). Once that was achieved, a new overlying soft tissue prediction model was produced which was dependent on the magnitude of the actual skeletal surgical movements ( Fig. 3 ).
Data processing
Both pre- and post-surgical DICOM files for each patient were imported into Maxilim and a conventional 3D virtual volumetric image produced of the hard and soft tissue. The hard and soft tissue surfaces for each patient were then saved as STL surface models.
For each patient, the pre- and post-surgical hard tissue surface models were imported into VRMesh, and the post-surgical image was translated, rotated, and superimposed on the anterior cranial base and frontal bone of the cranium of the pre-surgical image. The maxilla and mandible of the post-surgical model were isolated and exported from VRMesh as an STL file into the Maxilim software. This produced the actual post-surgical position of the maxilla and mandible realigned to the frame of reference of the pre-surgical image ( Fig. 1 ).
The pre-surgical DICOM image of each patient was then imported into Maxilim. A virtual Le Fort I osteotomy was carried out on the pre-surgical model with the maxilla and mandible translated, rotated, and re-aligned to the final post-surgical position ( Fig. 2 ). Once that was achieved, a new overlying soft tissue prediction model was produced which was dependent on the magnitude of the actual skeletal surgical movements ( Fig. 3 ).
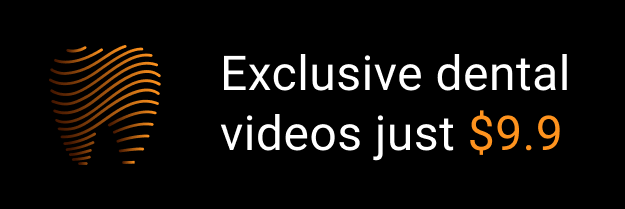