Abstract
Objectives
This study evaluates the hypothesis that the incorporation of fibrous hydroxyapatite nanoparticles with high crystallinity and high aspect ratio, synthesized by hydrothermal method, into an experimental ethanol-based one-bottle dentin adhesive, improves the mechanical properties of the adhesive layer, and accordingly increases the bond strength to dentin.
Methods
Hydroxyapatite nanorods were synthesized using a simple hydrothermal procedure. First, the HPO 4 2 -containing solution was added drop-wise into the Ca 2+ -containing solution while the molar ratio of Ca/P was adjusted at 1.67. The HAp precursor was then treated hydrothermally at 200 °C for 60 h. The resulting powder was characterized using XRD, FTIR, SEM, TEM, and EDXA. The synthesized HAp nanorods were added to an experimental one-bottle dentin adhesive followed by the characterization of the filled adhesive. The diametral tensile strength, flexural strength, flexural modulus, and the microshear bond strength to the dentin of human premolars of seven adhesive systems containing different nanorod contents were evaluated. The distribution of the filler was determined using EDX-mapping. The depth of cure was also evaluated using scraping technique. Moreover, after microshear testing, the fracture cross-section was observed using SEM to determine the mode of failure involved. The colloidal stability was studied using a separation analyzer and also zeta potential measurement. Data were analyzed using one-way analysis of variance followed by the Tukey test.
Results
The results confirmed the high purity, high crystallinity, and high aspect ratio of synthesized HAp nanorods. The diametral tensile strength of nanorod containing adhesive system appeared to increase when 0.2–0.5 wt.% HAp nanorods were incorporated ( p < 0.05). A similar trend was observed in the flexural test providing higher flexural strength at filler contents of 0.2–0.5 wt.% while flexural modulus remained unchanged. The highest microshear bond strength was also obtained at 0.2 wt.% filler content ( p < 0.05). The improved properties of the new adhesive system might be due to the high crystallinity and high aspect ratio of the nanorods. SEM observation of debonded surfaces revealed that most specimens showed an adhesive failure from the adhesive–dentin interface. Energy dispersive X-ray (EDX) mapping confirmed the uniform distribution of nanorods in the adhesive matrix. The colloidal stability studies indicated that synthesized hydroxyapatite nanorods have high colloidal stability in the dental adhesive solution. Indeed, the nanorods are well dispersed and protected from aggregation by their high surface charge confirmed by zeta potential measurement.
Significance
Hydroxyapatite-based composites have shown promising bioactivity. However, the knowledge about the influence of the nano-sized HAp on the properties of the dental materials, especially dentin bonding adhesives, is yet insufficient. The nanorod containing adhesive system presented here might be considered to have practical applications in dental clinics.
1
Introduction
Dental adhesives, especially one step systems, are now widely used in restorative and artistic dentistry due to their conservative and generally aesthetic roles. In fact, the clinical performance of composite restorations depends in part upon complete adhesion of the restorative composites to enamel and dentin. For enamel, the acid-etch procedure is usually successfully used . Dentin, however, is a hydrated biological composite consists of inorganic materials (mostly hydroxyapatite), organic materials (collagen), and water with properties that vary significantly with location. Moreover, dentin contains fluid-containing dentinal tubules which make a dynamic and wet substrate for bonding agents and a more complicated condition in comparison to the enamel .
Recently nanoparticles have been used in the formulation of restorative composite systems . With the aim of improving physical and mechanical properties, nanoparticles have also been incorporated into the dental adhesives . The filler particles are used in dental adhesives to fortify the bond strength of adhesive to dentin by penetrating into the dentin tubules, decreasing polymerization shrinkage, and increasing the elastic modulus of adhesive layer. Because of their very small sizes, nanoparticles are able to penetrate into the dentinal tubules along with the adhesive matrix resin providing a composite adhesive layer with improved properties. Although some researches report the application of nanofillers in dental adhesives , it is still a challenging subject in the field of dental material. The type and concentration of these fillers and their colloidal stability in the dilute adhesives remain a matter of concern.
As the major component of inorganic material of tooth is hydroxyapatite, it might be a promising material for the preparation of new dental adhesives with improved mechanical and biological properties. Although HAp nanoparticles are currently used as coatings in orthopedic and dental implants , no information is available on the effect of hydroxyapatite on dentin bonding systems. Hydroxyapatite (Ca 10 (PO 4 ) 6 (OH) 2 , HAp), which is a form of calcium phosphate, is one of the most biocompatible materials, but the low mechanical characteristics have limited its application for load-bearing materials . It has also been reported that an experimental dental composite material containing surface modified nanoparticles of hydroxyapatite is unsuitable for clinical performance .
One of the procedures to improve the mechanical characteristics of HAp nanoparticles is the induction of one-dimensional growth of crystals to form nanorods . However, the preparation of nano-sized rods with high aspect ratio, appropriate stoichiometry, and high crystallinity still remains as an interesting challenge . Several methods have been reported for the synthesis of hydroxyapatite nanorods such as solid-state synthesis , hydrothermal method , and solvothermal procedure .
In the present study, fibrous HAp nanoparticles were synthesized using hydrothermal method and their morphology, stoichiometry, and crystallinity were characterized. The synthesized HAp nanorods were then incorporated into an experimental one-bottle dentin bonding system and the mechanical properties of the adhesive and microshear bond strength to dentin were studied. The results were compared with those of a commercially available dentin bonding system. Different methods were utilized to characterize the bulk and adhesive properties of the bonding system. The colloidal stability of HAp-filled adhesive was also assessed.
2
Experimental
2.1
Materials
Calcium nitrate tetrahydrate, di-ammonium hydrogen phosphate, 2-hydroxyethyl methacrylate (HEMA), camphorquinone (CQ), 2-ethyl-2-(hydroxymethyl)-1,3-propandiol trimethacrylate (TMPTMA), formaldehyde solution, and ethanol were purchased from Merck (Germany). N , N ′-Dimethyl aminoethyl methacrylate (DMAEMA) was obtained from Fluka (Germany). 2,2-Bis[ p -(2-hydroxy-3-methacryloxypropoxy)phenyl] propane (Bis-GMA) and 1,6-bis-[2-methacryloyloxyethoxycarbonylamino]-2,4,4-trimethylhexane (UDMA) were kindly supplied by Röhm (Degussa Group, Germany). All reagents were analytical grade and used as received. The dental composite (Universal Restorative A3.5 Body Shade) and the one-bottle dentin bonding (Adper™ Single Bond 2, a commercially available nanoparticle containing dentin bonding used as the gold standard) were obtained from 3M ESPE (USA). The 37.5% phosphoric acid gel (Kerr Gel Etchant) was obtained from SDS Kerr (USA). Deionized water was used throughout the experiments.
2.2
Methods
2.2.1
Synthesis of HAp nanorods
Solutions of Ca 2+ and HPO 4 2− with concentration of 0.1 M were prepared by dissolving appropriate amounts of Ca(NO 3 ) 2 ·4H 2 O and (NH 4 ) 2 HPO 4 in deionized water, respectively. The phosphate ion solution was then added into the calcium ion solution at the rate of 0.1 ml/min under continuous and gentle stirring, while molar ratio of Ca/P was kept at stoichiometric amount according to its ratio in hydroxyapatite (1.67). The HAp precursor was transferred into a 100 ml autoclave and treated hydrothermally at 200 °C for 60 h, then followed by cooling naturally. Finally the synthesized powder was separated by centrifuging the suspension at 10,000 rpm for 20 min. The precipitate was washed thoroughly four times by a mixture of deionized water and ethanol (volume ratio of 1:1), dried at 60 °C, and then crushed into the powder form.
2.2.2
Powder characterization
The HAp nanorods were characterized using X-ray diffractometer (XRD, Model: D5000, SIEMENS, Germany) with CuKα ( λ = 1.5418 Å) radiation over the 2 range of 10–80° at room temperature with a step size of 0.02° and Fourier transform infrared spectroscopy (FTIR, Model: EQUINOX55, BRUKER, Germany) using KBr pellet technique. The fraction of the crystalline phase in HAp nanoparticles was determined according to the following equation :
X c = 1 − V 112 / 3 0 0 I 3 0 0
where I 3 0 0 represents the intensity of (3 0 0) diffraction peak, and V 1 1 2/3 0 0 represents the intensity of the valley between the peaks of (1 1 2) and (3 0 0).
The morphology and size of the nanoparticles were studied using scanning electron microscopy (TESCAN, VEGAII, XMU, Czech Republic). The elemental compositions of the products were quantitatively identified by energy dispersive X-ray analysis (EDXA, Model: QX2, RONTEC Co.) which was coupled by SEM.
Transmission electron microscopy (TEM) observations of the particles were conducted on a CEM-902A, Zeiss Co. (Germany) at an accelerating voltage of 80 kV.
2.2.3
Adhesive preparation
An experimental ethanol-based one-bottle dentin bonding was prepared. The details of the formulated adhesive mixture are provided in Table 1 . The as-synthesized HAp nanorods were incorporated, in weight ratios ranging from 0% to 5%, into the adhesive solution and then homogenized by ultra-sonication using a probe sonicator apparatus (Sonoplus UW2200, Bandelin, Germany) for 1 min. To prepare a one-component light-cure formulation, CQ/DMADME photoinitiating system at a 0.5:0.5 weight ratio (with respect to the total adhesive resin) were mixed therein. Finally, prepared adhesive mixtures containing 0%, 0.2%, 0.5%, 1%, 2%, and 5% (by weight) HAp nanorods were divided into two groups. The first group was used in microshear bond strength test. In the second group, the solvent of the adhesives was vacuum-evaporated for 2 days in subambient light to prepare mechanical test specimens of the bulk resins.
Materials | % Weight |
---|---|
Ethanol | 40 |
1,6-Bis-[2-methacryloyloxyethoxycarbonylamino]-2,4,4-trimethylhexane (UDMA) | 12 |
2,2-Bis[ p -(2-hydroxy-3-methacryloxypropoxy)phenyl] propane (Bis-GMA) | 14 |
2-Hydroxyethyl methacrylate (HEMA) | 26 |
2-Ethyl-2-(hydroxymethyl)-1,3-propandiol trimethacrylate (TMPTMA) | 8 |
2.2.4
Evaluation of colloidal stability
The colloidal stability measurement was carried out using a separation analyzer (LUMiReader ® 416.1, LUM, Germany). The specimen was prepared by dispersing 0.2 wt.% of HAp nanorods in the adhesive solution and sonication for 2 min. Monitoring of colloidal stability was performed for 60 h using visible light with intensity of 100%. Moreover, the colloidal stability was also investigated using an indirect procedure, based on the knowledge of the zeta potential, by means of the Helmholtz-Smoluchowski model . In fact, the zeta potential ( ζ ) is the electrical charge of particle surface which provides information about the stability of the colloidal systems . However, a threshold value for determining whether a suspension is stable or not is not equal for dissimilar particles and the relative zeta potential is only used to compare the colloidal stability of homologous particles in the same media. In our previous study the hydroxyapatite nanoparticles, synthesized by solvo-treatment method, showed a low colloidal stability so that sedimentation was completed after about 2 h . These nanoparticles were used to compare the zeta potential of the suspensions containing the particles. Zeta potential measurements were performed using an electrophoretic technique. Samples were placed in a clear disposable zeta cell that acted as the measurement chamber of the electrophoresis instrument (Zetasizer Nanoseries ZS, Malvern Instruments, UK) and then the external DC voltage at the constant value of 100 V was applied. The colloidal stability is determined by measuring electrophoretic mobility of the dispersed particles in the applied electric field. The electrophoretic mobility measurements were then converted into ζ -potential values using Helmholtz-Smoluchowski. The reported values for ζ -potential are the average of at least six measurements.
2.2.5
Mechanical testing
Tensile and flexural strength are of the important mechanical test for assessing the performance of dental resins . Diametral tensile strength (DTS) test provides a simple method for the measurement of the tensile strength of brittle materials such as dental adhesives. DTS test was performed by adopting the procedure of ANSI/ADA specification no. 27 for light cure resins. The solvent-free resins were inserted in a cylindrical stainless-steel mold with the internal diameter of 6 mm and height of 3 mm, which was placed on a glass slide. Then, the mold was covered with another glass slide and specimens were cured from both sides using a light-curing unit (Demetron LC. SDS Kerr, USA; light intensity, 600 mW/cm 2 ) for 40 s. The specimens were removed from the mold and stored in deionized water for 1 day at room temperature prior to the test. At least 7 specimens were tested for each formulation and the diameter and height of each specimen was measured using a digital micrometer before the test. The universal testing machine (SMT 20, Santam, Iran) was used for the test utilizing a load cell of 5 kN at the cross-head speed of 10 mm/min. The DTS (MPa) was then calculated according to the following equation:
DTS = 2 p π D L
where p is the load at fracture (N), D (mm) and L (mm) are the diameter and height of specimens, respectively.
The flexural strength of the unfilled and HAp-filled adhesives was measured according to the ISO 4049(2000) standard method. The specimens were prepared in stainless-steel rectangular mold with dimensions of 2 mm × 2 mm × 25 mm utilizing the same light-curing unit. After 1 day of storage in deionized water, a three-point bending test was performed using the universal testing machine at a cross-head speed of 0.5 mm/min. The flexural strength (FS) in MPa was calculated as:
FS = 3 p L 2 b d 2
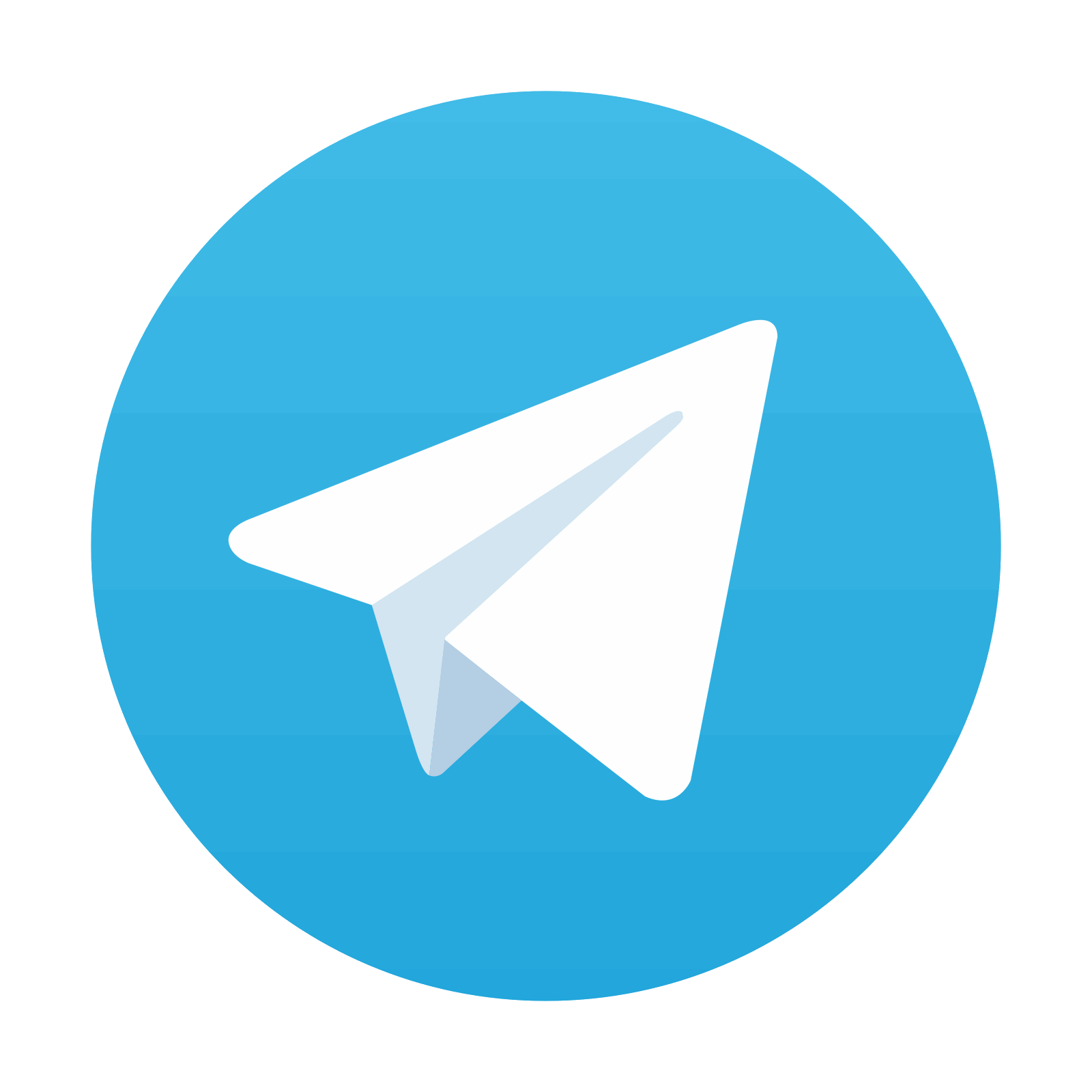
Stay updated, free dental videos. Join our Telegram channel

VIDEdental - Online dental courses
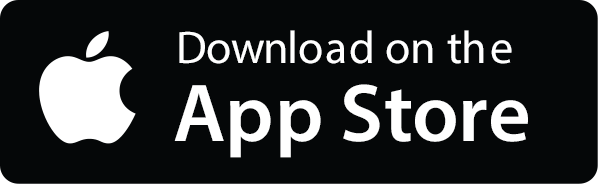
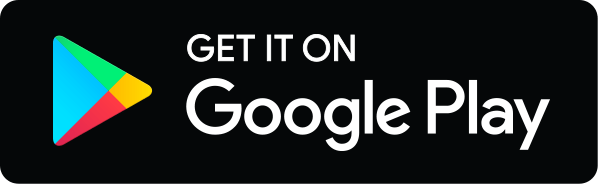