Abstract
Objective
To evaluate in vitro Streptococcus mutans ( S. mutans ) biofilm formation on the surface of five light-curing experimental dental bonding systems (DBS) with increasing hydrophilicity. The null hypothesis tested was that resin chemical composition and hydrophilicity does not affect S. mutans biofilm formation.
Methods
Five light-curing versions of experimental resin blends with increasing hydrophilicity were investigated (R 1 , R 2 , R 3 , R 4 and R 5 ). R 1 and R 2 contained ethoxylated BisGMA/TEGDMA or BisGMA/TEGDMA, respectively, and were very hydrophobic, were representative of pit-and-fissure bonding agents. R 3 was representative of a typical two-step etch-and-rinse adhesive, while R 4 and R 5 were very hydrophilic resins analogous to self-etching adhesives. Twenty-eight disks were prepared for each resin blend. After a 24 h-incubation at 37 °C, a multilayer monospecific biofilm of S. mutans was obtained on the surface of each disk. The adherent biomass was determined using the MTT assay and evaluated morphologically with confocal laser scanning microscopy (CLSM) and scanning electron microscopy (SEM).
Results
R 2 and R 3 surfaces showed the highest biofilm formation while R 1 and R 4 showed a similar intermediate biofilm formation. R 5 was more hydrophilic and acidic and was significantly less colonized than all the other resins. A significant quadratic relationship between biofilm formation and hydrophilicity of the resin blends was found. CLSM and SEM evaluation confirmed MTT assay results.
Conclusions
The null hypothesis was rejected since S. mutans biofilm formation was influenced by hydrophilicity, surface acidity and chemical composition of the experimental resins. Further studies using a bioreactor are needed to confirm the results and clarify the role of the single factors.
1
Introduction
Resin-based composites are increasingly used because of their excellent esthetic properties and improved mechanical characteristics . The evolution of polymer matrices and filler particles composition enhanced the performances of these materials along with more reliable bonds to dental hard tissues. Nevertheless, the main reason for failure of resin composite restorations is still secondary caries occurring at the interface between the composite material/dentin bonding systems (DBS) and enamel and dentin .
As the development of such lesions is mainly related to the effect of acids and enzymes produced by bacteria colonizing the interface, the interactions between the surface of resin composites, DBS and the overlying biofilm greatly influence the lifespan of the adhesive restorations. A relevant problem is that resin composite and DBS surfaces are more heavily colonized by oral biofilms than surfaces of other restorative materials (such as amalgam, glass-ionomer cements and ceramics), as well as sound enamel surfaces .
Dentin bonding systems are blends of hydrophilic and hydrophobic monomers with the ability to couple the hydrophobic resin composite materials to hydrophilic surfaces such as dentin or enamel. They may be classified as etch-and-rinse or self-etching adhesives. These adhesives may be further classified as multi-step ( i.e. three-step etch-and-rinse and two-step self-etching) or simplified by combining the number of steps required for the clinical application ( i.e. two-step etch-and rinse and one-step self-etching) . Since simplified formulations involve mixing of nonsolvated adhesives with solvated primers ( i.e. two-step etch-and-rinse) or with self-etching primers ( i.e. one-step self-etch), DBS simplification strongly increases the hydrophilicity of the mixture and of the bond . It has been previously shown that the chemical composition of DBS influences bacterial colonization. The results suggest that variations in the chemical structure of the monomers, solvents or application techniques can extensively influence biofilm formation even when a DBS does not contain any specific antibacterial formulation .
Previous studies investigated the effect of commercially available DBS on cariogenic bacteria colonization. Pinheiro et al. studied Streptococcus mutans biofilm formation on the surface of different DBS. The authors concluded that different materials produce diverse colonization levels in relation to their variable chemical composition, solvent and application technique. Comparison between etch-and-rinse and self-etching adhesives showed lower S. mutans colonization for the latter, when compared to the etch-and-rinse approach . Additionally, discrepancies in bacterial growth induced by different one-step self-etching adhesives and self-etching primers have been reported .
Experimental resins that cover a wide range of hydrophilicity and other properties of contemporary DBS have been formulated (R 1 –R 5 ); these resins rank from very hydrophobic to very hydrophilic, as manifested by their solubility parameters . Previous studies have evaluated their tensile strength, modulus of elasticity, degree of conversion, influence of solvent content, water sorption and solubility . However, no data is available regarding the influence of the hydrophilicity of these experimental resin blends on surface biofilm formation. Since S. mutans is considered to be one of the most important microorganisms responsible for primary and secondary caries , the aim of the present study was to investigate the influence of experimental DBS chemical composition and hydrophilicity on S. mutans colonization in vitro . The null hypothesis tested was that hydrophilicity of DBS does not affect S. mutans biofilm formation.
2
Materials and methods
2.1
Specimen preparation
All reagents and multi-well plates used in the present study were purchased from Sigma–Aldrich (St. Louis, MO) unless otherwise specified.
Five light-curing versions of neat experimental resin blends with increasing hydrophilicity were investigated (R 1 , R 2 , R 3 , R 4 and R 5 ). Their compositions are listed in Table 1 . All blends included 0.25% camphorquinone and 1% 2-ethyl-dimethyl-4-aminobenzoate as the photoinitiator and accelerator, respectively. Resin blend R 1 and R 2 are similar to nonsolvated hydrophobic resins used in the formulation of the bonding agent of three-step etch-and-rinse and two-step self-etching adhesives. Resin blend R 3 represents a typical two-step etch-and-rinse adhesive. Resin blends R 4 and R 5 contain methacrylate derivatives of carboxylic and phosphoric acids, respectively, and they have the highest hydrophilicity, similarly to one-step self-etching adhesives. All resin blends were formulated in an increasing order of hydrophilicity based on their Hoy’s solubility parameters ( Table 1 ).
Resin # | Neat resin composition | Hoy’s solubility parameters (J/cm 3 ) ½ | |||
---|---|---|---|---|---|
δ d | δ p | δ h | δ t | ||
R 1 | 70 wt% E-BisADM | 15.0 | 10.3 | 6.6 | 19.4 |
28.75% TEGDMA | |||||
R 2 | 70% BisGMA | 15.9 | 12.4 | 6.9 | 21.2 |
28.75% TEGDMA | |||||
R 3 | 70% BisGMA | 15.6 | 13.0 | 8.5 | 22.1 |
28.75% HEMA | |||||
R 4 | 40% BisGMA | 16.5 | 12.9 | 7.0 | 22.1 |
30% TCDM | |||||
28.75% TEGDMA | |||||
R 5 | 40% BisGMA | 15.1 | 13.5 | 11.1 | 23.1 |
30% BisMP | |||||
28.75% HEMA |
Twenty-eight disks of each experimental resin blend (6.4 ± 0.1 mm diameter; 1.00 ± 0.02 mm thick) were prepared by placing 45 μL of resin on the bottom of modified 96-well polystyrene plates. The plates were sealed with impression material (Express™ 2 Light Body Standard, 3M ESPE, Seefeld, Germany), leaving a 2 mm void space between the lid and the wells. Then, two holes (3 mm diameter) were prepared on top of lid. The holes were sealed by plastic joints and connected by tubing to a nitrogen source. A constant flow of nitrogen was maintained inside the plates for 20 min to create an oxygen-free environment. The resin blend in each well was cured through the bottom of the plate for 80 s, using a quartz-tungsten halogen light-curing unit (Spectrum 800, Dentsply International Inc., York, PA, USA). The light-curing unit was set at a power level of 800 mW/cm 2 . This procedure was used to obtain a smooth resin surface and a perfect fit between the resin disk and the well, overcoming the formation of an oxygen-inhibited layer and the need for surface finishing.
Each plate was stored under light-proof conditions for 24 h at 37 °C to allow complete polymerization of the resins. Then 200 μL of sterile phosphate-buffered saline (PBS) were added to each well. Plates were stored at room temperature for an additional 7 days, allowing unreacted monomers to leach out. To remove those compounds, each well was rinsed twice a day with 200 μL of sterile PBS. The plates were sterilized with a chemical peroxide-ion plasma sterilizer (STERRAD, ASP, Irvine, CA, USA) for 60 min at a maximum temperature of 45 °C to prevent heat-induced modification of the resin surface.
2.2
Bacteria
All the culture media were obtained from Becton–Dickinson (BD Diagnostics-Difco, Franklin Lakes, NJ, USA). S. mutans ATCC 25175 was cultured on Mitis Salivarius Bacitracin agar. The plates were incubated at 37 °C for 48 h in a 5% supplemented CO 2 environment and a pure suspension of the microorganism in Brain Heart Infusion (BHI) was obtained from these plates after an incubation time of 12 h at 37 °C in a 5% supplemented CO 2 environment. S. mutans cells were harvested by centrifugation (2200 rpm for 5 min at 19 °C), rinsed twice with PBS and resuspended in the same buffer. The suspension was then sonicated to disperse bacterial chains (Sonifier model B-15, Branson, Danbury, CT, USA, operating at 40 W energy output). The optical density was adjusted to 0.3 OD units at 550 nm using a spectrophotometer (Genesys 10-S, Thermo Spectronic, Rochester, NY, USA), which corresponds to a microbial concentration of 3.65 × 10 8 cells/mL.
2.3
MTT assay reagents
A 3-(4,5-dimethylthiazol-2-yl)-2,5-diphenyltetrazolium bromide (MTT) stock solution was prepared by dissolving 5 mg/mL of MTT in sterile PBS. A N-methylphenazonium methyl sulfate (PMS) stock solution was prepared by dissolving 0.3 mg/mL of PMS in sterile PBS. The solutions were stored at 2 °C in light-proof conditions until the day of the experiment. A fresh measurement solution (FMS) was prepared by mixing 1 mL of MTT stock solution, 1 mL of PMS stock solution, and 8 mL of sterile PBS. A lysing solution (LS) was prepared by dissolving 10% (v/v) of sodium dodecyl sulfate and 50% (v/v) of dimethylformamide in distilled water.
2.4
Biofilm development and MTT assay
Sixteen disks prepared from each experimental resin blend were used for this test. Twenty microliter of the S. mutans cell suspension and 180 μL of sterile BHI supplemented with 5% sucrose were placed in each well of the plates. The mixture was incubated for 24 h at 37 °C in a 5% supplemented CO 2 environment, as required for the development of a multilayer biofilm . In brief, the wells were carefully washed three times with sterile PBS to remove non-adherent cells. One hundred microliter of FMS was then placed in each well and the plates were incubated for 3 h at 37 °C in light-proof conditions. During incubation, microbial redox systems converted the yellow MTT salt to insoluble purple formazan. The unreacted FMS was carefully removed and formazan crystals were dissolved by adding 100 μL of LS to each well and by incubating for 1 h at room temperature under light-proof conditions. Ninety microliter of suspension were obtained from each well and the optical density at 550 nm was measured using a microplate reader (Genesys 10-S, Thermo Spectronic, Rochester, USA).
2.5
Laser confocal microscopy (CLSM) and scanning electron microscopy (SEM)
Six disks from each resin blend were analysed using CLSM and six disks examined using SEM. After the 24-h incubation time, the biofilm growing on the disks was gently washed three times with PBS to remove non-adherent cells and stained using the FilmTracer™ LIVE/DEAD ® Biofilm Viability Kit for microscopy (Invitrogen Ltd., Paisley, United Kingdom). The fluorescence from live stained cells adherent to the test samples was observed using a CLSM (Leica TCS SP5, Leica Microsystems, Wetzlar, Germany). Ten randomly selected image stack sections were recorded for each biofilm specimen. Confocal images were obtained using a 40× (NA 1.25) oil immersion objective and digitalized by using the Leica Application Suite Advanced Fluorescence Software (LAS AF, Leica microsystems, Wetzlar, Germany), at a resolution of 1024 × 1024 pixels, with a zoom factor of 3.0. For each image stack, a maximum-intensity projection (MIP) reconstruction was obtained.
Using the same incubation time, the 6 disks selected for SEM observation were gently washed three times with PBS to remove non-adherent cells and placed in a 2% glutaraldehyde fixative solution for 1 week. The specimens were then transferred to 70, 80, 85, 90, and 95% (v/v) ethanol solutions for 24 h each, and finally in a 100% ethanol solution for 48 h. Finally, the specimens were critical point dried (Critical-point Dryer, EMS 850, Hatfield, PA, USA), mounted on stubs with conductive glue, sputter-coated (JEOL FFC-1100, Japan), and observed with a scanning electron microscope (JEOL JSM-5300, Japan) at a magnification of 2000×.
2.6
pH analysis
Resin disks were obtained at the bottom of 96 well plates by placing 45 μL of resin on the bottom of modified 96-well polystyrene plates and by polymerizing in a nitrogen atmosphere as specified in paragraph 2.1. Then, resin specimens underwent rinsing (as previously described in Section 2.1 ) and pH measurements were conducted on 4 specimens for each resin blend using a ROSS micro-pH electrode (Thermo Scientific, Waltham, MA, USA) which fitted inside the wells of 96-well plates. Two-hundred microliters of distilled water or PBS or BHI solution were inserted inside the specimen-containing wells and measurements were performed immediately (time = 0 h) or after 3 h, 6 h, 12 h and 24 h. After that, solutions were discarded and plates were rinsed three times with distilled water and 200 μL of new solutions were inserted again inside the wells; measurements were performed immediately (time = 24 h, after rinsing). Every 20 measurements the electrode was checked against standard solutions and, if necessary, recalibrated.
2.7
Statistical analysis
Statistical analysis was performed with Stata v.10 software (Stata v.10, StataCorp., College Station, TX, USA). Normal distribution of data was checked using the Shapiro–Wilk test and homogeneity of variances was verified using Bartlett’s test. One-way ANOVA ( p < 0.05) was performed and Student–Neumann–Keuls post hoc test was used to assess differences among the resin groups. The level of significance ( α ) was set to p < 0.05. The existence of a relationship between Hoy’s parameters and biofilm formation on the surfaces of the different resin blends was evaluated by fitting the data series with a quadratic function.
2
Materials and methods
2.1
Specimen preparation
All reagents and multi-well plates used in the present study were purchased from Sigma–Aldrich (St. Louis, MO) unless otherwise specified.
Five light-curing versions of neat experimental resin blends with increasing hydrophilicity were investigated (R 1 , R 2 , R 3 , R 4 and R 5 ). Their compositions are listed in Table 1 . All blends included 0.25% camphorquinone and 1% 2-ethyl-dimethyl-4-aminobenzoate as the photoinitiator and accelerator, respectively. Resin blend R 1 and R 2 are similar to nonsolvated hydrophobic resins used in the formulation of the bonding agent of three-step etch-and-rinse and two-step self-etching adhesives. Resin blend R 3 represents a typical two-step etch-and-rinse adhesive. Resin blends R 4 and R 5 contain methacrylate derivatives of carboxylic and phosphoric acids, respectively, and they have the highest hydrophilicity, similarly to one-step self-etching adhesives. All resin blends were formulated in an increasing order of hydrophilicity based on their Hoy’s solubility parameters ( Table 1 ).
Resin # | Neat resin composition | Hoy’s solubility parameters (J/cm 3 ) ½ | |||
---|---|---|---|---|---|
δ d | δ p | δ h | δ t | ||
R 1 | 70 wt% E-BisADM | 15.0 | 10.3 | 6.6 | 19.4 |
28.75% TEGDMA | |||||
R 2 | 70% BisGMA | 15.9 | 12.4 | 6.9 | 21.2 |
28.75% TEGDMA | |||||
R 3 | 70% BisGMA | 15.6 | 13.0 | 8.5 | 22.1 |
28.75% HEMA | |||||
R 4 | 40% BisGMA | 16.5 | 12.9 | 7.0 | 22.1 |
30% TCDM | |||||
28.75% TEGDMA | |||||
R 5 | 40% BisGMA | 15.1 | 13.5 | 11.1 | 23.1 |
30% BisMP | |||||
28.75% HEMA |
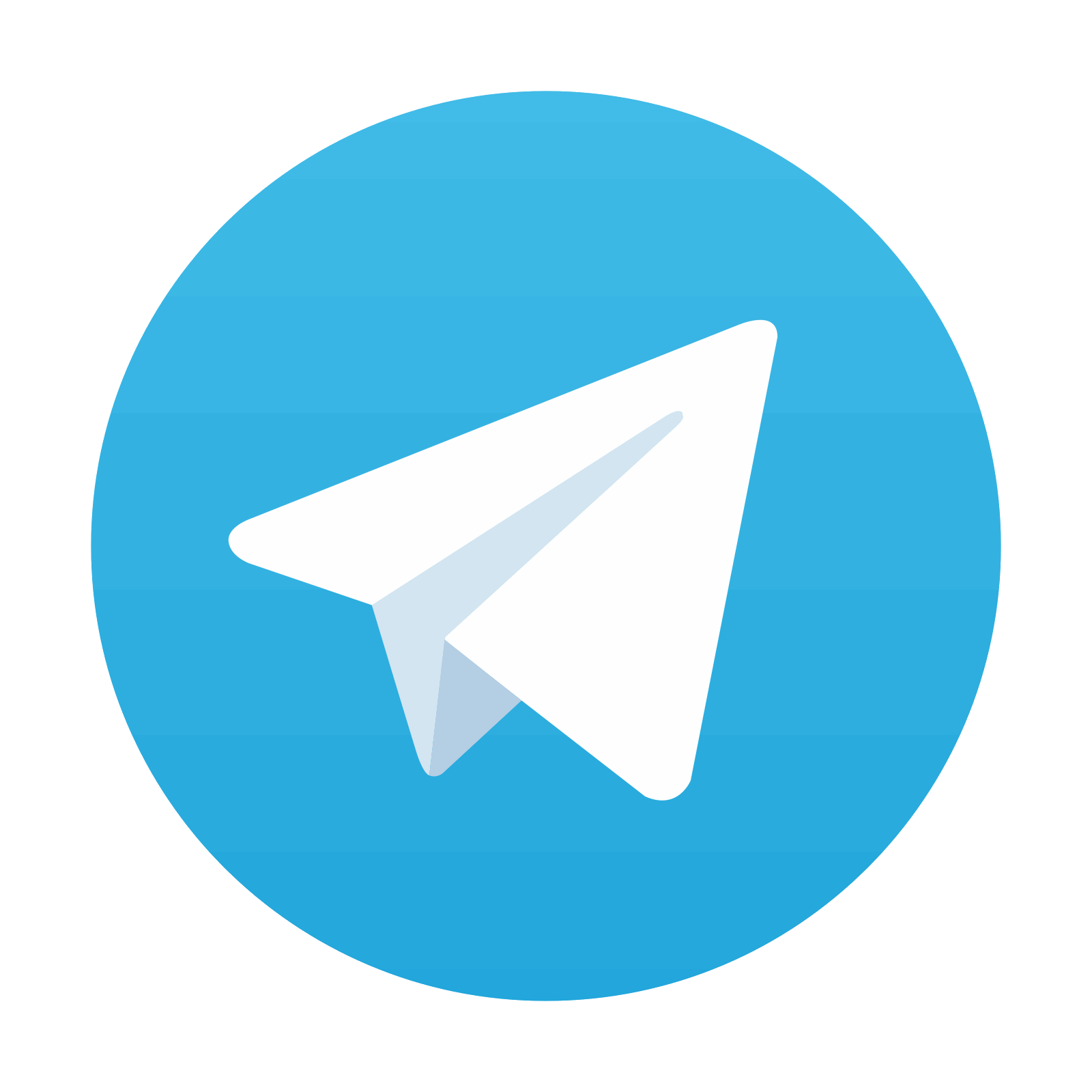
Stay updated, free dental videos. Join our Telegram channel

VIDEdental - Online dental courses
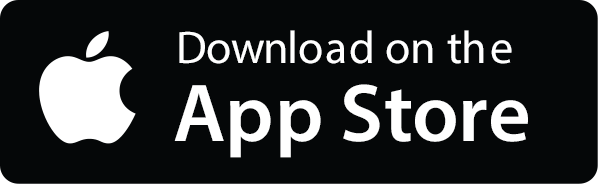
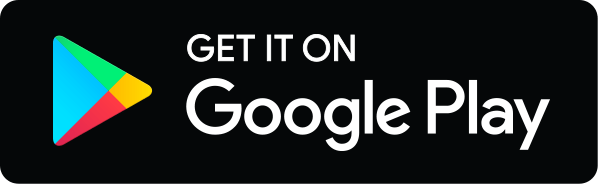
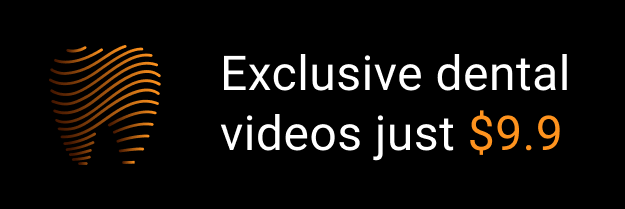