Abstract
The aim of this systematic review and meta-analysis was to investigate how parameters related to geometry influence the clinical performance of orthodontic mini-implants (MIs). Systematic searches were performed in electronic databases including MEDLINE, Scopus, Web of Science, Virtual Health Library, and Cochrane Library and reference lists up to March 2016. Eligibility criteria comprised clinical studies involving patients who received MIs for orthodontic anchorage, with data for categories of MI dimension, shape, and thread design and insertion site, and evaluated by assessment of primary and secondary stability. Study selection, data extraction, quality assessment, and a meta-analysis were carried out. Twenty-seven studies were included in the qualitative synthesis: five randomized, eight prospective, and 14 retrospective clinical studies. One study with a serious risk of bias was later excluded. Medium and short MIs (1.4–1.9 mm diameter and 5–8 mm length) presented the highest success rates (0.87, 95% CI 0.80–0.92). A maximum insertion torque of 13.28 N cm (standard error 0.34) was observed for tapered self-drilling MIs in the mandible, whereas cylindrical MIs in the maxilla presented a maximum removal torque of 10.01 N cm (standard error 0.17). Moderate evidence indicates that the clinical performance of MIs is influenced by implant geometry parameters and is also related to properties of the insertion site. However, further research is necessary to support these associations.
Since their first clinical use almost two decades ago, orthodontic mini-implants (MIs) have been investigated extensively and adopted worldwide. This is mainly due to their potential to expand the biomechanical modalities for orthodontic treatment, based on the skeletal anchorage principle . These devices represent an effective alternative, especially in non-compliant patients and those with insufficient dental structures for conventional anchorage reinforcement .
Scientific and clinical studies on MIs have reported mechanical and biological factors , as well as clinical applications, including the intrusion, extrusion, and uprighting of impacted teeth, distalization of the maxillary and/or mandibular teeth, en-masse retraction of the anterior teeth, and the management of sagittal and vertical discrepancies . The correction of maxillary transverse discrepancies supported by MIs in adult patients who have been treated conventionally with a surgical approach has also been reported recently . Despite the great progress made so far, improvements in the clinical performance of MIs is still a major topic of interest.
In order to be considered clinically effective, MIs must provide resistance to orthodontic forces for the entire period in which anchorage reinforcement is required during treatment . Nonetheless, MIs may present episodes of loosening, mobility, or displacement , and may be associated with injury to the adjacent structures and inflammation or infection of the surrounding tissues . Stability is one of the essential factors related to MI permanence at the site of insertion. This is initially represented by the mechanical interaction between the MI surface and surrounding bone, referred to as primary stability, and is followed by a biological bone healing process, which represents secondary stability .
Due to the proximity of MI insertion sites to surrounding nerves, vessels, and especially dental roots and tissues , it is important to consider the dimensions of MIs to prevent injuries. Furthermore, it is known from concepts of oral implantology that implant geometry may influence the stress distribution pattern over the bone interface . Previous evidence from orthodontic MI studies has indicated relationships between factors of MI geometry, such as diameter and length , shape, thread form, and pitch, and the MI stability achieved .
Previous systematic reviews that have investigated implant-, patient-, and insertion-related factors have provided evidence of several factors potentially related to MI performance . However, due to the wide range of MI designs used in these primary studies and the different outcome assessments performed, no objective consensus regarding the influence of MI geometry design on both primary and secondary stability has yet been achieved. The relevance of this topic for both clinicians and researchers highlights the need for a study design that considers parameters of MI geometry as the main object of analysis in order to obtain accurate and up-to-date information.
The aim of this systematic review and meta-analysis was to investigate whether parameters related to MI geometry influence the clinical performance of orthodontic MIs.
Materials and methods
Protocol and registration
The study protocol was registered in the PROSPERO database ( http://www.crd.york.ac.uk/PROSPERO; number CRD42015025286). It was performed in accordance with the PRISMA statement and following the guidelines in the Cochrane Handbook for Systematic Reviews of Interventions (version 5.1.0) .
Eligibility criteria
The selection criteria for this review were defined with consideration to the elements of the PICOS question, as outlined below.
Population (P): the population comprised patients of both sexes, without restriction on age, ethnic, or socioeconomic group, whose orthodontic treatment with fixed appliances required skeletal anchorage reinforcement.
Intervention (I): the intervention comprised the placement of orthodontic MIs of less than 2.5 mm in diameter for skeletal anchorage. Other skeletal anchorage systems, for example osseointegrated implants, palatal implants, onplants, and miniplates, were excluded.
Comparison (C): the different geometrical characteristics of orthodontic MIs in isolation or in relation to the insertion site (maxilla or mandible) were actively compared by subgroup analysis. Due to the wide variability in MI geometry characteristics, they were assigned to categories according to the following criteria: (1) dimensions (diameter and length): the MI diameter was classified as small (1.1–1.3 mm), medium (1.4–1.9 mm), or large (2.0–2.5 mm), and the length was classified as short (5–8 mm) or long (8.5–15 mm); (2) shape: MIs that presented a uniform body format were categorized as cylindrical, and those that narrowed from the head to the tip area were categorized as tapered; (3) thread design: predrilling MIs require a pilot hole to be made using a bone drill, while self-drilling MIs are those placed without any previous drilling.
Outcome (O): primary and secondary outcomes included the assessment of clinical performance, specifically through the evaluation of primary and secondary concepts of mini-implant stability, respectively. Primary stability is the stability measured immediately after the implantation procedure . Studies that recorded maximum insertion torque (MIT) and mobility were included. MIT can be recorded using a mechanical or electronic screwdriver during the MI insertion process , and is expressed in Newton centimetres (N cm). MI mobility values measured objectively, for example through resonance frequency analysis or electromechanical percussion, were considered; subjective assessments were not included. Secondary stability is expressed after the healing phase . Therefore, studies that measured the MI maximum removal torque (MRT) values, as well as those that evaluated the ability of the MI to remain in situ until the completion of its anchorage purpose, were considered. Studies that recorded the MRT after the removal process and quantified the permanence of MI through success rates with a minimum period of 6 months of follow-up were included. Mini-implants that became unusable, were lost, or fractured were defined as failures. In order to evaluate the influence of the follow-up period on secondary stability, two time frames were considered: 6–12 months and >12 months.
Study design (S): eligible studies included randomized controlled trials (RCTs) and non-randomized clinical studies, both prospective and retrospective. Animal and laboratory studies, technical and case reports, opinion and review articles were excluded.
Information sources, search strategy, and study selection
Systematic searches were performed of the following electronic databases through March 2016: PubMed (MEDLINE), Scopus, Web of Science, Virtual Health Library (VHL), and Cochrane Library. The Clinical Trials database was searched to identify unpublished or ongoing trials related to the review question. The grey literature was searched via the System for Information on Grey Literature in Europe (SIGLE) through OpenGrey. Abstracts from the annual conference of the International Association for Dental Research (IADR) and their regional divisions, and theses and dissertations were also consulted. The reference lists of all retrieved studies were screened manually and experts were also contacted to identify additional relevant data and other publications, unpublished or ongoing studies . No restrictions were placed on the publication date or language. A librarian (D.M.) who is expert in computerized searches of publications assisted with the conception and development of the search strategy.
Controlled vocabulary (MeSH terms) and free key words used in the search strategy were based on the population (P); intervention (I); and comparison (C) elements; irrespective of the outcomes measured or study design reported . The search strategies were designed in accordance with the criteria of each database; as described in Supplementary Table 1.
Two investigators (A.C.C. and A.M.A.V.) screened all titles and abstracts retrieved from the database searches and excluded irrelevant records. Duplicate studies were excluded using EndNote Web software (Thomson Reuters, New York, USA), followed by a manual revision. If insufficient information was provided in the abstract of a paper, the full text was assessed before a final decision was made. After selecting the potentially relevant studies, the same authors reviewed the full texts against the selection criteria. Eligibility was assessed independently, and in the case of any inconsistency, a third author (L.C.M) was consulted.
Data items and collection
The following data were extracted: (1) author, year of publication, and geographical location; (2) study design; (3) sample size (numbers of participants and MIs); (4) area of MI insertion; (5) MI characteristics (dimensions and shape), if reported; (6) thread design, if reported; (7) follow-up period, and (8) outcome evaluation criteria. The data extraction forms were pilot tested for their validity and consistency with the review question.
Risk of bias/quality assessment for individual studies
The assessment of quality and control of bias was conducted independently by two investigators (A.C.C. and A.M.A.V.). The Cochrane Collaboration tool was used to assess the risk of bias of RCTs, as recommended in the Cochrane Handbook for Systematic Reviews of Interventions (version 5.1.0) . The following key domains were considered: (1) adequate sequence generation, (2) allocation concealment, (3) blinding of participants, (4) blinding of outcome assessment, (5) incomplete outcome data, (6) selective reporting, and (7) other sources of bias. For the third domain, a minor change was made, with the removal of the evaluation of blinding of personnel. The blinding of personnel would not be possible, as the MI design characteristics are easily distinguishable, especially in the head portion, and the auxiliary tools differ recognizably. Each domain was judged as showing a low risk, high risk, or unclear risk. With regard to the overall risk of bias, the study was considered as ‘high risk’ when at least one key domain was assigned a high risk, as ‘low risk’ when all key domains were assigned a low risk, and as ‘unclear risk’ when there was insufficient information available to categorize the study as ‘high risk’ or ‘low risk’.
The risk of bias of non-randomized clinical studies was assessed using the ROBINS-I tool (Risk Of Bias In Non-randomised Studies − of Interventions) . The ROBINS-I version for cohort-type studies (version 19 September 2016) includes seven domains: bias due to confounding, selection of participants into the study, classification of interventions, deviations from intended interventions, missing data, measurements of outcomes, and selection of the reported result. The first two domains refer to the pre-intervention period, the third one addresses the time of the intervention, and the last four refer to the post-intervention period. The risk of bias within each domain is judged by answering the questions with ‘yes’, ‘probably yes’, ‘probably no’, or ‘no’; a response of ‘no information’ can also be recorded. According to the response to the question, the risk of bias is assigned as ‘low risk’, ‘moderate risk’, ‘serious risk’, or ‘critical risk’. The overall risk of bias is then based on the judgement for each domain. In the case of any disagreement in the risk of bias assessment, a third author (L.C.M) was consulted.
Summary measures and approach to synthesis
MIT, mobility, and MRT of the MIs were expressed as continuous variables with mean and standard deviation values. MI success rates constituted dichotomous event rates. Confidence intervals (CI) were set at 95%. Weighted means among the studies were calculated through the random-effects model, and heterogeneity was assessed using the Cochran Q test and I 2 statistic . All analyses were performed using Comprehensive Meta-Analysis software version 3.2 (Biostat, Inc., Englewood, NJ, USA).
Additional analyses
Structured comparisons among the different types of MI geometry characteristics and insertion sites were performed by subgroup analysis. In addition, due to the number of representative studies collected, a cumulative analysis of MI success rates over time was conducted.
Results
Study selection and characteristics
A flow diagram of the search and selection procedures, according to the PRISMA guidelines, is shown in Fig. 1 . Of the 7152 articles initially retrieved, 4352 remained after the removal of duplicates. A total 4060 records were excluded after title screening and a further 160 after abstract screening. Following this, 132 full-text articles were assessed for eligibility; 105 records were excluded following full-text reading (the respective reasons for exclusion are shown in Fig. 1 ). At this stage, the systematic reviews and meta-analyses retrieved were excluded for not addressing the comparison (C) element of the PICOS question . Finally, 27 studies were included in the qualitative synthesis: five RCTs , eight prospective clinical studies , and 14 retrospective clinical studies .

Characteristics of the included studies, including study design, numbers of participants and MIs, insertion area, MI characteristics, thread design, evaluation criteria, follow-up period, and primary/secondary outcomes are detailed in Supplementary Table 2. Studies were published between 2003 and 2015 and were performed in 11 different countries and states: Poland, Colombia, Taiwan, India, Japan, Italy, Korea, Thailand, Greece, Germany and China. MIs of many different dimensions were used in the studies; diameters ranged from 1.0 mm to 2.3 mm , and lengths from 4 mm to 15 mm . Information about mini-implant shape was found in only a few studies and the tapered type was most commonly used. Regarding the thread design, the predrilling type was the most common . A total of 3934 MIs were placed in 1769 patients, and the mean follow-up period was 11.5 months.
Two different papers with potential overlapping samples were identified . The corresponding author of both articles was contacted with no reply. Therefore, the data collected from these articles were included in the meta-analysis disregarding the group with presumed overlapping information in order to avoid duplication of data in the analysis .
Risk of bias within studies
The quality control and risk of bias assessment indicated that all five RCTs had an unclear risk of bias, mainly due to insufficient information about allocation concealment, blinding of participants and personnel, and blinding of the outcome assessment (see Supplementary Fig. S1) (Review Manager (RevMan) version 5.3; The Nordic Cochrane Centre, The Cochrane Collaboration, Copenhagen, 2014). With regard to the non-randomized studies, 21 were considered to have a moderate risk of bias and one a serious risk of bias (see Supplementary Fig. S2). This result was attributed mainly to bias due to confounding, in the measurement of outcomes, and in the selection of reported results. After exclusion of the low quality study, 26 articles remained for quantitative synthesis (meta-analysis). The authors were contacted by e-mail for additional data and the information obtained was also included .
Results of individual studies, meta-analysis, and subgroup analyses
A lack of inflammation and mobility, satisfactory anchorage, and an adequate period of permanence were the main criteria established by the primary studies to evaluate MI success, which was expressed quantitatively with the success rate. The results of the meta-analysis showed an overall success rate of 0.86 (95% CI 0.83–0.89) for the cumulative analysis ( Fig. 2 A). The success rate was 0.85 (95% CI 0.82–0.88) for studies with 6–12 months of follow-up and 0.88 (95% CI 0.81–0.92) for studies with more than 12 months of follow-up ( Fig. 2 B).

Subgroup analysis revealed success rates of 0.89 (95% CI 0.86–0.92) for maxillary insertion sites and 0.82 (95% CI 0.77–0.86) for mandibular insertion sites ( Fig. 3 ).

Regarding parameters related to geometry, success rates for the different MI dimensions ranged from 0.71 (95% CI 0.43–0.89) for small and short MIs to 0.87 (95% CI 0.80–0.92) for medium and short MIs; the overall success rate was 0.82 (95% CI 0.78–0.92) ( Fig. 4 A). Rates for MI shape according to insertion site groups ranged from 0.81 (95% CI 0.71–0.88) for cylindrical MIs in the mandible to 0.88 (95% CI 0.60–0.97) for cylindrical MIs in the maxilla ( Fig. 4 B). Rates for the different thread design groups ranged from 0.87 (95% CI 0.82–0.91) for self-drilling to 0.90 (95% CI 0.87–0.92) for predrilling MIs ( Fig. 4 C).
The insertion and removal torque subgroup analyses according to MI shape and thread design are show in Figs 5 and 6 , respectively. Maximum insertion and removal torques were mainly measured by torque wrench and sensors. MIT values for the MI shape analysis according to insertion site ranged from 7.05 N cm (standard error (SE) 0.14) for cylindrical MIs in the maxilla to 13.28 N cm (SE 0.34) for tapered MIs in the mandible ( Fig. 4 A). The thread design subgroups showed MIT of 7.14 N cm (SE 0.13) for predrilling MIs in the maxilla and 10.08 N cm (SE 0.24) for self-drilling MIs in the maxilla ( Fig. 4 B). For structured comparisons of shape and thread design, a higher MIT was observed for tapered self-drilling MIs inserted in the mandible (mean 13.28 N cm, SE 0.34) ( Fig. 4 C).
Removal torque values were similar between the subgroups of tapered and cylindrical MIs inserted in the mandible (mean 8.49 N cm (SE 0.24) and 7.84 N cm (SE 0.25), respectively), whereas in the maxilla, cylindrical MIs presented higher MRT than the tapered ones (mean 10.01 N cm (SE 0.17) and 7.35 N cm (SE 0.20), respectively) ( Fig. 6 ). Due to an insufficient number of studies on MI mobility, this outcome could not be assessed in the present study.
This meta-analysis was conducted with the random-effects model, and different heterogeneity levels were found according to subgroups, with I 2 values ranging from 33.99% (success rates for MI thread design subgroup; Fig. 6 C) to 99.85% (MRT of insertion site and MI shape subgroup; Fig. 6 ). The heterogeneity was considered high in most comparisons ( I 2 above 50% and Q test with a statistically significant P -value).
Results
Study selection and characteristics
A flow diagram of the search and selection procedures, according to the PRISMA guidelines, is shown in Fig. 1 . Of the 7152 articles initially retrieved, 4352 remained after the removal of duplicates. A total 4060 records were excluded after title screening and a further 160 after abstract screening. Following this, 132 full-text articles were assessed for eligibility; 105 records were excluded following full-text reading (the respective reasons for exclusion are shown in Fig. 1 ). At this stage, the systematic reviews and meta-analyses retrieved were excluded for not addressing the comparison (C) element of the PICOS question . Finally, 27 studies were included in the qualitative synthesis: five RCTs , eight prospective clinical studies , and 14 retrospective clinical studies .
Characteristics of the included studies, including study design, numbers of participants and MIs, insertion area, MI characteristics, thread design, evaluation criteria, follow-up period, and primary/secondary outcomes are detailed in Supplementary Table 2. Studies were published between 2003 and 2015 and were performed in 11 different countries and states: Poland, Colombia, Taiwan, India, Japan, Italy, Korea, Thailand, Greece, Germany and China. MIs of many different dimensions were used in the studies; diameters ranged from 1.0 mm to 2.3 mm , and lengths from 4 mm to 15 mm . Information about mini-implant shape was found in only a few studies and the tapered type was most commonly used. Regarding the thread design, the predrilling type was the most common . A total of 3934 MIs were placed in 1769 patients, and the mean follow-up period was 11.5 months.
Two different papers with potential overlapping samples were identified . The corresponding author of both articles was contacted with no reply. Therefore, the data collected from these articles were included in the meta-analysis disregarding the group with presumed overlapping information in order to avoid duplication of data in the analysis .
Risk of bias within studies
The quality control and risk of bias assessment indicated that all five RCTs had an unclear risk of bias, mainly due to insufficient information about allocation concealment, blinding of participants and personnel, and blinding of the outcome assessment (see Supplementary Fig. S1) (Review Manager (RevMan) version 5.3; The Nordic Cochrane Centre, The Cochrane Collaboration, Copenhagen, 2014). With regard to the non-randomized studies, 21 were considered to have a moderate risk of bias and one a serious risk of bias (see Supplementary Fig. S2). This result was attributed mainly to bias due to confounding, in the measurement of outcomes, and in the selection of reported results. After exclusion of the low quality study, 26 articles remained for quantitative synthesis (meta-analysis). The authors were contacted by e-mail for additional data and the information obtained was also included .
Results of individual studies, meta-analysis, and subgroup analyses
A lack of inflammation and mobility, satisfactory anchorage, and an adequate period of permanence were the main criteria established by the primary studies to evaluate MI success, which was expressed quantitatively with the success rate. The results of the meta-analysis showed an overall success rate of 0.86 (95% CI 0.83–0.89) for the cumulative analysis ( Fig. 2 A). The success rate was 0.85 (95% CI 0.82–0.88) for studies with 6–12 months of follow-up and 0.88 (95% CI 0.81–0.92) for studies with more than 12 months of follow-up ( Fig. 2 B).
Subgroup analysis revealed success rates of 0.89 (95% CI 0.86–0.92) for maxillary insertion sites and 0.82 (95% CI 0.77–0.86) for mandibular insertion sites ( Fig. 3 ).

VIDEdental - Online dental courses
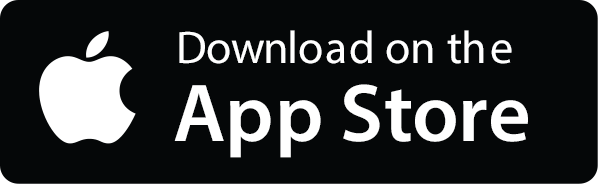
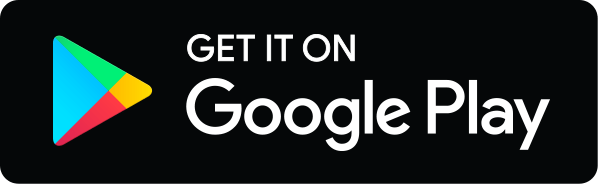