Highlights
- •
Refractive index changes via nanoparticle incorporation or organic functionalization.
- •
Refractive index adaption leads to highly translucent composites.
- •
Specimen thickness has great influence on translucency.
- •
ORMOCER®-based composites are biocompatible.
Abstract
Objectives
For dental resin composites, high translucency is important. Therefore, the aim of the study was to create a biocompatible and highly translucent resin-based composite, and to investigate the effect of material thickness on translucency.
Methods
A biocompatible ORMOCER® resin matrix was reinforced with dental glass powder as fillers. To reach a high translucency, refractive index matching of the matrix and fillers was done in the two ways: (1) Highly refractive ZrO 2 nanoparticles were incorporated into the resin. (2) The resin was modified via addition of 4-Methylthiophenol. The corresponding refractive indices were acquired on an Abbe refractometer (n = 5). In both cases, the dental glass powder was added and translucency of the resulting minifilled and nanohybrid composites were measured using spectral photometry (n = 5). Additionally, the translucency of the experimental composites was determined as a function of specimen thickness in the range 10 μm–2 mm (n = 5). One-way ANOVA was performed to determine the significant differences in various optical parameters among different amounts of modifications and thicknesses at α = 0.05. Furthermore, cytotoxicity tests (extract and direct contact tests) were conducted according to ISO 10993 to classify the biocompatibility of the composites (n = 6).
Results
The translucency values of the composites with 47 wt.-% dental glass powder and a specimen thickness of 2 mm, could be increased from 26% up to 71% by increasing the refractive index of the matrix through incorporating ZrO 2 nanoparticles. Moreover, it can also be increased to 67% via addition of 4-Methylthiophenol. Further results showed that the translucency significantly depended on the sample thickness following an exponential function. The effect of all tested parameters was significant among the materials (p < 0.001). The composites did not show any cytotoxic effect.
Significance
Highly translucent and biocompatible resin composites were developed. They show attractive properties for the use as dental enamel material in direct and indirect restorations.
1
Introduction
In the early 1960s, the first dental resin-based composites were developed by Bowen [ ] by mixing Bis-GMA (bisphenol A glycerolate dimethacrylate) with inorganic particles [ ]. In the last 50 years, dental resin composites have been optimized and have become increasingly popular for both direct and indirect dental restorations [ ]. They have replaced the conventional dental amalgams [ ] due to their advantages such as aesthetic and mechanical properties, biocompatibility, and user-friendliness [ , ]. In general, dental resin composites consist of polymeric matrices, inorganic fillers, and initiators [ , , ]. Organic matrix monomers like Bis-GMA, TEGDMA (triethylene glycol dimethacrylate), and UDMA (urethane dimethacrylate) are widely used [ , ]. Moreover, another group of promising resin matrices are inorganic-organic hybrid polymers, the so called ORMOCER®s, which were developed in the 1990s for dental application [ , ]. Their typical inorganic Si-O-Si-structure which is formed via a sol-gel process is covalently linked to a polymerizable organic group. Compared to pure organic matrix materials, ORMOCER®s show a reduced polymerization shrinkage [ , ], improved abrasion resistance [ , ], and high biocompatibility [ ], to name but a few advantages of these materials. The inorganic fillers reinforce the matrix by increasing the mechanical properties, improving aesthetic and radiopacity properties, and reducing polymerization shrinkage [ , , ]. Commonly, quartz, colloidal silica, and silica glass which contains barium, strontium, zirconium, aluminum, ytterbium, boron, zinc and fluoride are utilized as different types of fillers [ , , ]. Silane coupling agents on the fillers are very important for the covalent linking between the filler and matrix, and therefore the overall properties of the composite [ , ]. Composites containing milled glass fillers in the range of 0.1–1 μm can be classified as minifilled composites [ ], whereas when nanoparticles are added, the resulting products are classified as nanohybrid composites [ , ].
Due to the gradually increasing demand for aesthetics in dentistry, it has become necessary for the restorations to have a natural appearance to blend with the surrounding teeth [ , ]. Besides the color itself, the translucency of the restoration is a primary factor in determining the aesthetic outcome [ ]. Generally, natural tooth enamel has a higher translucency, compared to dentin which is less translucent [ ]. Therefore, depending on the type ( e.g. veneer, inlay, onlay, and crown) and location ( e.g. anterior/posterior, incisal/cervical) of the indirect restoration, the desired translucency varies considerably [ ]. Especially in the anterior region a translucency adapted to the surrounding natural teeth is of high importance. Hence, restorative material with a wide range of translucencies is needed. Typically, with a layered buildup technique, using opaque and translucent material, a natural appearance of the restoration can be obtained [ ].
Translucency can be described as an intermediate state of transparency and opacity. A translucent material allows the passage of light through its interior, but in contrast to transparent material, it disperses light, which prevents a clear observation of the objects behind it [ , ]. Generally, a change in the refractive index leads to a change in the light path. For specimens consisting of two or more components, as is the case with composites, the different materials such as the resin matrix and the inorganic fillers, need to have similar refractive indices to become highly translucent. The degree of translucency and opacity of the composite is defined based on how well the refractive indices of the matrix and the fillers match, respectively [ , ].
As the change in the direction of light adds up with increasing distance covered by it, the translucency decreases with increasing thickness of the material [ , ]. Research has shown an exponential correlation between the thickness ( x ) of a specimen and its corresponding translucency ( y ). The correlation can be expressed using the following equation [ , ]:
y=a∙e-b∙x
where a and b are constants. Since translucency is determined by the ratio of the intensity of the transmitted light to that of the incident light, it can be compared with the physical correlation of the Lambert-Beer law [ ]:
I(d)=I0∙e-μ∙d
where I(d) is the intensity of the transmitted light, I 0 is the intensity of the incident light, <SPAN role=presentation tabIndex=0 id=MathJax-Element-3-Frame class=MathJax style="POSITION: relative" data-mathml='μ’>?μ
μ
is the extinction coefficient, which is a measure of the light weakening ability of a material, and d is the specimen thickness [ ].
Besides the aesthetic aspect of the final restoration, the translucency of a material plays a significant role during the production of the restoration using additive manufacturing technologies based on photopolymerization of resin materials. Different processes, like stereolithography (SLA) or Digital Light Processing (DLP), work by curing thin layers of photosensitive material on top of each other using a laser or LED light source for SLA or DLP, respectively [ ]. For a better understanding of the polymerization process, the relationship between the translucency and thickness of a material must be known to avoid errors of overcuring in particular at overhanging parts since there is no fusing with a bottom layer [ ].
The purpose of the study was to develop biocompatible minifilled and nanohybrid composites based on different inorganic-organic hybrid polymers (ORMOCER®s). The focus was particularly on generating highly translucent materials by perfectly matching the refractive index of the resin matrix and inorganic fillers. The long-term objective is to process these materials via 3D-printing to indirect restorations. The null hypotheses tested were that
- 1)
the incorporation of highly refractive ZrO 2 nanoparticles or the addition of aromatic and sulfur-containing molecules would not change the refractive index of the resin matrix;
- 2)
an adaption of the refractive indices of the resin matrix and fillers would not affect translucency;
- 3)
material thickness would not affect translucency; and
- 4)
changes to the material would result in a non-biocompatible material.
2
Materials and methods
2.1
Materials
Three methacrylate(-acrylate)-based monomer-free ORMOCER® resin systems were used in the study (see Fig. 1 ). The synthesis processes of the resin systems are described in a patent application [ ] according to the examples “Grundharzsystem 2”, “Harzsystem 5”, and “Harzsystem 6” for resins A, B, and C, respectively. Resin B is a siloxane co-condensation product. The resins C are based on resin B, which was further functionalized with different amounts of 4-Methylthiophenol (0.35 to 0.55 mol per mol resin B). The double-bond contents of the three resins are 4.6 mmol/g, 5.7 mmol/g, and 3.6–4.3 mmol/g for resins A, B, and C, respectively. The varying double-bond content of resin C depends on the amount of 4-Methylthiophenol added.

The photoinitiator 2,4,6-trimethylbenzoyl-diphenyl-phosphine oxide (LTPO) was purchased from BASF (Germany). The ZrO 2 nanoparticles PixClear® PCPB-2-50-ETA (particle size of 7−10 nm, n D = 1.85) were purchased from Pixelligent (USA). The dental glass powder of type GM27884 (UltraFine-type with a grain size of 0.7 μm (d 50 ), n D = 1.528 ± 0.003) was purchased from Schott (Germany). Freeprint® temp (A3) was purchased from Detax (Germany). Signum composite dentine (DA3) and Signum composite enamel (EL) were purchased from Heraeus Kulzer (Germany). in:joy Dentin (A3) and in:joy Incisal (medium) were purchased from Dentsply (Germany). All materials were used as received.
2.2
Preparation of the composites
First, 1 wt.-% LTPO was dissolved in the respective resin. For the preparation of the nanocomposites, their respective resins A and B were put in a round flask. The ZrO 2 nanoparticle dispersion (50 wt.-% in ethyl acetate) was added, and the mixture was stirred at 22 °C for 1 h. The mixture was then placed under vacuum at 35 °C for the removal of the solvent. The mass and volume fractions of the nano-sized ZrO 2 in the nanocomposites based on resins A and B are listed in Table 1 .
Resin | ZrO 2 mass fraction [wt.-%] | ZrO 2 volume fraction [vol.-%] |
---|---|---|
Resin A | 10 | 2.2 |
15 | 3.5 | |
18 | 4.3 | |
20 | 4.8 | |
22 | 5.4 | |
25 | 6.3 | |
Resin B | 18 | 4.4 |
22 | 5.5 | |
25 | 6.5 | |
27 | 7.1 | |
30 | 8.2 | |
35 | 10.1 |
For the minifilled and nanohybrid composites, dental glass powder (DGP) was added to resins A, B and C, as well as to the nanocomposites based on resins A and B. The silanized dental glass powder was manually premixed with the resins and nanocomposites, using hand spatulation. After completely wetting the powder with the resin matrix, the composite pastes were thoroughly blended in a three roll mill (EXAKT 80E, Exakt Advanced Technologies, Germany) at 60 °C. The mass and volume fractions of the inorganic fillers of the produced minifilled and nanohybrid composites can be seen in Table 2 . Each material was degassed under vacuum before use.
Resin | 4-Methylthiophenol content | ZrO 2 content | DGP content | Total filler content | |||
---|---|---|---|---|---|---|---|
[mol/mol] | [wt.-%] | [vol.-%] | [wt.-%] | [vol.-%] | [wt.-%] | [vol.-%] | |
Resin A | – | 0.0 | 0.0 | 47.0 | 26.8 | 47.0 | 26.8 |
– | 5.3 | 1.6 | 47.0 | 28.4 | 52.3 | 30.0 | |
– | 8.0 | 2.4 | 47.0 | 29.3 | 55.0 | 31.7 | |
– | 9.5 | 3.0 | 47.0 | 29.9 | 56.5 | 32.9 | |
– | 10.3 | 3.3 | 47.0 | 30.2 | 57.3 | 33.4 | |
– | 11.7 | 3.8 | 47.0 | 30.7 | 58.7 | 34.5 | |
– | 13.0 | 4.3 | 47.0 | 31.2 | 60.0 | 35.6 | |
Resin B | – | 0.0 | 0.0 | 30.0 | 15.3 | 30.0 | 15.3 |
– | 12.6 | 3.6 | 30.0 | 17.4 | 42.6 | 21.0 | |
– | 15.4 | 4.5 | 30.0 | 17.9 | 45.4 | 22.5 | |
– | 17.5 | 5.3 | 30.0 | 18.3 | 47.5 | 23.6 | |
– | 18.9 | 5.7 | 30.0 | 18.7 | 48.9 | 24.4 | |
– | 21.0 | 6.6 | 30.0 | 19.3 | 51.0 | 25.9 | |
– | 24.5 | 8.2 | 30.0 | 20.3 | 54.5 | 28.5 | |
Resins C | 0 | – | – | 30.0 | 15.3 | 30.0 | 15.3 |
0.35 | – | – | 30.0 | 15.4 | 30.0 | 15.4 | |
0.40 | – | – | 30.0 | 15.4 | 30.0 | 15.4 | |
0.45 | – | – | 30.0 | 15.4 | 30.0 | 15.4 | |
0.50 | – | – | 30.0 | 15.4 | 30.0 | 15.4 | |
0.50 | – | – | 47.0 | 27.3 | 47.0 | 27.3 | |
0.55 | – | – | 30.0 | 15.4 | 30.0 | 15.4 |
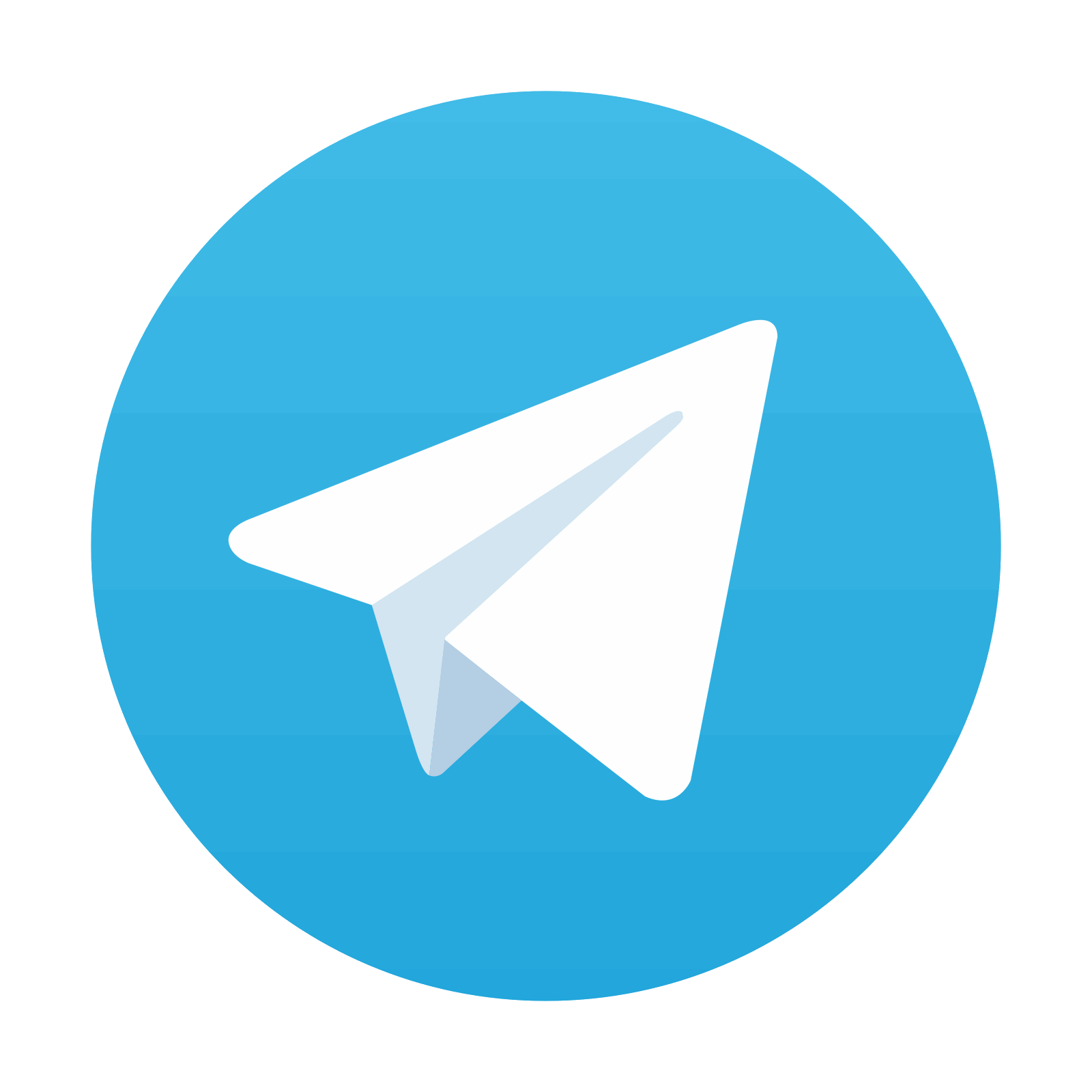
Stay updated, free dental videos. Join our Telegram channel

VIDEdental - Online dental courses
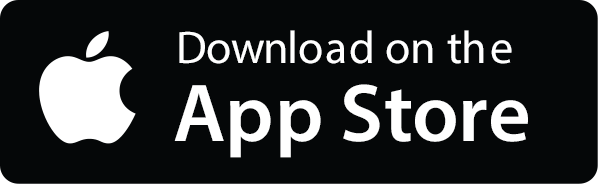
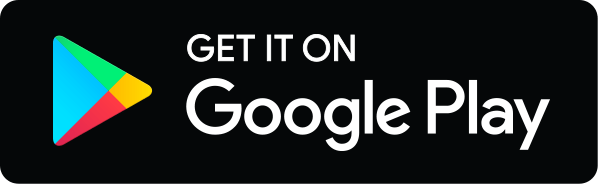
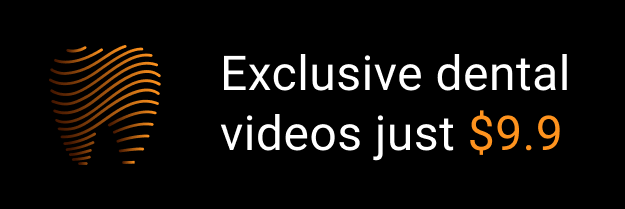