Highlights
- •
Proteoglycans are tightly bound to collagen acting as a barrier for infiltration of dental resin monomers.
- •
Trypsin digestion removed PGs resulting in altered water balance and surface wettability of the dentin matrix.
- •
Low hydrophilicity dental adhesives can effectively bond to dentin depleted of PGs.
Abstract
Objectives
To investigate the role of proteoglycans (PGs) on the physical properties of the dentin matrix and the bond strength of methacrylate resins with varying hydrophilicities.
Methods
Dentin were obtained from crowns of human molars. Enzymatic removal of PGs followed a standard protocol using 1 mg/mL trypsin (Try) for 24 h. Controls were incubated in ammonium bicarbonate buffer. Removal of PGs was assessed by visualization of glycosaminoglycan chains (GAGs) in dentin under transmission electron microscopy (TEM). The dentin matrix swelling ratio was estimated using fully demineralized dentin. Dentin wettability was assessed on wet, dry and re-wetted dentin surfaces through water contact angle measurements. Microtensile bond strength test (TBS) was performed with experimental adhesives containing 6% HEMA (H 6 ) and 18% HEMA (H 18 ) and a commercial dental adhesive. Data were statistically analyzed using ANOVA and post-hoc tests (α = 0.05).
Results
The enzymatic removal of PGs was confirmed by the absence and fragmentation of GAGs. There was statistically significant difference between the swelling ratio of Try-treated and control dentin (p < 0.001). Significantly lower contact angle was found for Try-treated on wet and dry dentin (p < 0.002). The contact angle on re-wet dentin was not recovered in Try-treated group (p = 0.9). Removal of PGs significantly improved the TBS of H 6 (109% higher, p < 0.001) and H 18 (29% higher, p = 0.002) when compared to control. The TBS of commercial adhesive was not affected by trypsin treatment (p = 0.9).
Significance
Changing the surface energy of dentin by PGs removal improved resin adhesion, likely due to more efficient water displacement, aiding to improved resin infiltration and polymerization.
1
Introduction
The dentin constitutes the bulk tissue of teeth providing biological shielding to the vascularized pulp and mechanical support to enamel [ ]. The extracellular matrix (ECM) comprises approximately 30% of the dentin and includes collagen and non-collagenous components [ ], serving as a scaffold for the controlled deposition of hydroxyapatite crystallites (50%) [ ]. Small leucine rich proteoglycans (SLRPs), the second most predominant non-collagenous component in dentin, are not only essential for dentin formation, but also the dentin’s ultra-structural and biomechanical features. These biomacromolecules are major constituents of mineralized and non-mineralized connective tissues [ ]. Proteoglycans (PGs) contain GAGs chains polysaccharides covalently attached to a core protein [ ]. Chondroitin-4-sulfate (C4S)/chondroitin-6-sulfate (C6S) are the most predominant glycosaminoglycans (GAGs) in dentin [ ]. The high water affinity of GAGs and their tight relationship with the collagen fibrils network [ , ] assures to the PGs the fundamental structural and biomechanical regulatory functions in the dentin matrix.
The dentin ECM is often exposed for the effective usage of man-made reparative/restorative biomaterials [ ], such as resin composites. Dental resin-based restorations rely on the infiltration of a hydrophilic/hydrophobic blend of resin monomers into partially demineralized dentin creating a micromechanical interlocking with the exposed collagen network [ ]. Hydrophilicity and residual water at the adhesive interface interfere with both the resin adhesion and interfacial stability over time [ , ]. Pitfalls leading to proteolytic and hydrolytic degradation of the dentin-resin interface could be minimized if successful infiltration and polymerization of the adhesive resin is attained [ ]. Hydrophilic monomers, such as HEMA, improve wettability and infiltration of dental adhesives into moist dentin [ , ]. However, hydrophilic monomers create a less stable hybrid layer [ ]. From the substrate perspective, the collagen inter-fibrillar spaces are essential for resin infiltration [ ]. The negative charges of GAGs contribute to incomplete water displacement from the demineralized dentin matrix [ ], which can impair collagen encapsulation by adhesive monomers [ , ], leading to faster breakdown of the dentin-resin interface.
Despite large evidence of the ultrastructural and mechanical roles of PGs in dentin, there is limited knowledge of the roles these non-collagenous proteins have in the infiltration and interactions of dental resins with the dentin ECM. The aim of this study was to evaluate the effect of PGs on the physical properties of the dentin matrix and the bond strength of experimental adhesive systems with varying hydrophilicity. The investigated null hypotheses were: (1) enzymatic removal of PGs does not affect the swelling and wettability of the dentin matrix, and (2) the dentin-resin bond strength of low-hydrophilicity experimental dental adhesive to dentin will not be affected by the enzymatic removal of PGs.
2
Material and methods
2.1
Substrate selection and protocols for enzymatic removal of proteoglycans
Dentin was obtained from the crown portion of sound extracted human molars, kept frozen for no longer than 2 months after extraction (Institutional Review Board protocol #2011-0312). An enzymatic digestion protocol was selected to remove PGs [ , ], using 1 mg/mL trypsin – Try (TPCK, Sigma-Aldrich, St. Louis, MO) in 0.2 M ammonium bicarbonate buffer (pH 7.5) for 24 h at 37 °C.
2.2
Visualization of PGs-GAGs – transmission electron microscopy (TEM)
Removal of PGs was determined by visualization of GAGs following staining with cupromeronic blue (CB) as published previously [ , ]. Five (5) teeth were pre-fixed in 10% buffered neutral formalin at 4 °C for 24 h. Then, mid-coronal dentin sections (1.5 × 1.5 × 0.5 mm) were obtained using a low-speed diamond saw (series 15LC, Buehler, Lake Bluff, IL, USA) under water irrigation and further fixed in 10% buffered neutral formalin for 3 days at 4 °C. Specimens were demineralized with 10% phosphoric acid (Ricca Chemical Company, Arlington, TX, USA) for 5 h under agitation and thoroughly washed with distilled water. The enzymatic removal of PGs was performed as described above (n = 5). Untreated dentin (control, n = 5) were incubated in 0.2 M ammonium bicarbonate. Specimens were immersed in 0.05% CB diluted in 25 mM NaAc containing 0.1 M MgCl 2 and 2.5% glutaraldehyde (pH 5.8) for 10 min (repeated 3 times), followed by immersion in 34 mM sodium tugnstate (pH 9.4) for 30 min. After, specimens were dehydrated in ascending concentration of ethanol (25%, 50% and 75% ethanol for 15 min each; 90% for 30 min; 95% for 30 min twice and 100% for 1 h twice). Specimens were embedded in LR white resin, sectioned into 70 nm thick sections, and sections were mounted on copper grids. No staining of collagen was performed to facilitate visualization of GAGs staining. Sections were analyzed and imaged in TEM (JEM-1220, JEOL, Peabody, MA) at 80 kV.
2.3
Water content of the dentin matrix – swelling ratio
The mid-coronal dentin of twelve (12) teeth were serially sectioned into 1.7 width × 6.0 length × 0.5 mm thickness specimens with a slow speed diamond saw (series 15LC, Buehler). The specimens were demineralized as described in section 2.2 . The enzymatic removal of PGs was performed as described in 2.1. The dry mass of demineralized dentin specimens of each group (n = 12) was measured after 24 h in a desiccator containing anhydrous calcium sulfate. Specimens were then immersed in distilled water for 1 h, kept overnight in 10 M PBS (pH 7.4), excess moisture was blotted dry and weighed immediately (wet mass) [ ]. The specimens were then placed in distilled water for 10 min to remove buffer salts, dried in a desiccator and weighed until a constant mass was obtained (no variance above 0.02 mg, approximately 1 h drying). The swelling ratio (Q) was calculated as the ratio between the weight of the swollen (wet mass) specimen to that of the dry specimen [ ]. Data were statistically analyzed by one-way Anova and Tukey’s post-hoc tests (α = 0.05).
2.4
Wettability of the dentin matrix – contact angle method
The wettability of the dentin surface was assessed by measuring the water contact angle. Twenty (20) teeth were divided into two groups (n = 10). The occlusal enamel was removed with #180 silicon carbide paper, followed by #320 and #600 grits under water to simulate a smear-layer covered dentin surface. The wettability was assessed on the dentin surfaces after acid etching with 35% phosphoric acid gel (3M Oral Care, St. Paul, MN, USA) (control) or acid etching + PG removal (Try-treated) under different conditions: wet (dentin kept visually moist as for the wet-bond adhesive technique) and dry (air-dried for 3 min) and re-wet (air-dried for 3 min and re-wetted for 1 min). One drop of water (2 μL) was placed on the dentin surface and the contact angle (θ) was assessed using a standard contact angle goniometer model 200-F4 (Ramé-hart instrument co., Succasunna, NJ, USA). The distance between the tip and the dentin surface was kept constant and all tests were performed under controlled temperature (23 ± 1 °C) and humidity (greater than 30%) [ ]. Images were acquired within 10 s and the water contact angle was calculated using the software DropImage (Ramé-hart instrument co.). Data were statistically analyzed by two-way ANOVA and Games-Howell post-hoc tests (α = 0.05).
2.5
Resin adhesion to dentin – microtensile bond strength
The dentin-resin adhesion was evaluated using a standard microtensile bond strength test (TBS). Thirty (30) teeth were divided into three groups (n = 10), had their occlusal enamel removed with #180 silicon carbide paper, and flat mid-coronal dentin exposed using #320 and #600 grits under running water. Dentin surfaces were etched with 35% phosphoric acid gel (3M Oral Care) for 30 s, rinsed for 60 s and either treated with trypsin (Try-treated) or not (control), as described in section 2.1 . Surfaces were restored with either a low hydrophilicity experimental adhesive (H 6 ) containing 6% HEMA or a high hydrophilicity experimental adhesive (H 18 ) containing 18% HEMA (composition in Table 1 ). A commercial adhesive system was used as control for adhesion experiments [Adper Single Bond Plus – SB (3M Oral Care, St. Paul, MN, USA)]. All adhesive systems were applied as recommended for SB. After bonding procedures, a resin composite (Filtek Supreme, 3M Oral Care) was placed in three increments of 2 mm as build-up for testing. Each increment was light polymerized for 40 s. A halogen light-curing unit operated at 600 mW/cm 2 (Optilux, Demetron; Danbury CT, USA) was used for adhesive and resin composite polymerization. Teeth were stored in distilled water at 37 °C for 24 h and then sectioned longitudinally across the bonded interface with a low-speed diamond saw (Buehler) under water irrigation to obtain specimens of approximately 1.0 mm 2 (± 0.02 mm 2 ), measured with a digital caliper (Digimatic Caliper, Mitutoyo; Tokyo, Japan). Specimens were mounted onto a Ciucchi’s jig and tested at crosshead speed of 0.5 mm/min using EZ-Graph instrument (Shimadzu Co., Tokyo, Japan). TBS was determined and data statistically analyzed by two-way ANOVA and Scheffe’s post hoc tests (α = 0.05). The fracture pattern was observed under a stereomicroscope at 40× magnification, and classified as adhesive, cohesive or mixed failures [ ].
Adhesive System | Composition | Application protocol |
---|---|---|
Adper Single Bond Plus – SB (3M Oral Care) | Bis-GMA, DMA, UDMA, HEMA, CQ, nanofillers, water, ethanol, methacrylate functional copolymer of polyacrylic and polytaconic acids | Leave dentin surface moist Apply three consecutive coats of adhesive Lightly dry to ensure removal of the solvent Light cure for 40 s |
H 6 (Experimental adhesive) |
41.25% Bis-GMA, 12% TEGDMA, 6% HEMA, 0.6% EDMAB, 0.15% CQ, ethanol | |
H 18 (Experimental adhesive) |
41.25% Bis-GMA, 18% HEMA, 0.6% EDMAB, 0.15% CQ, ethanol |
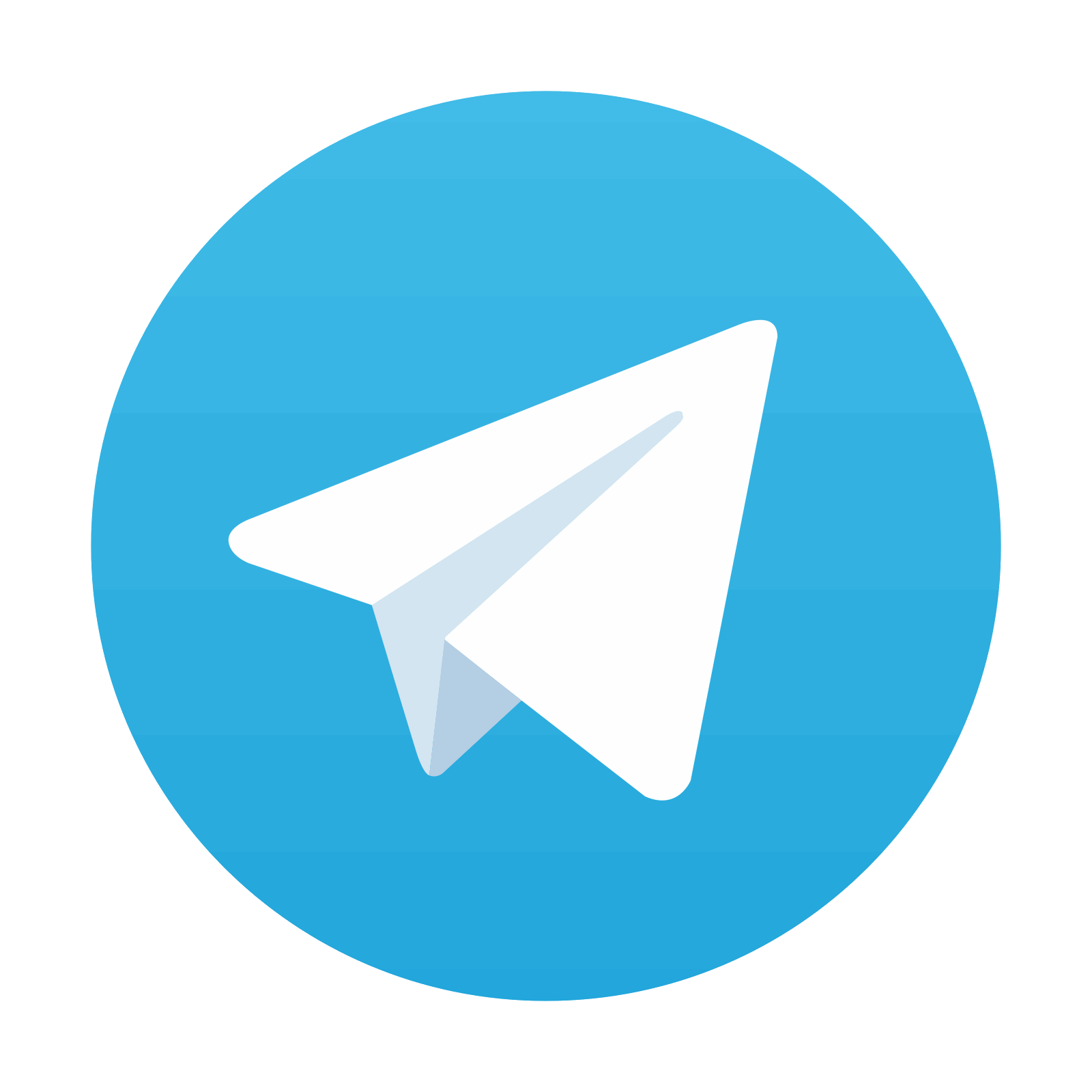
Stay updated, free dental videos. Join our Telegram channel

VIDEdental - Online dental courses
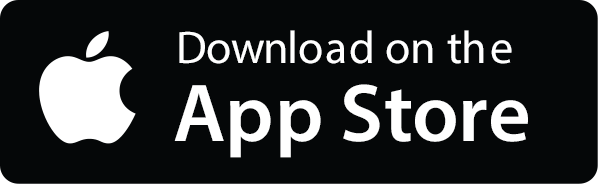
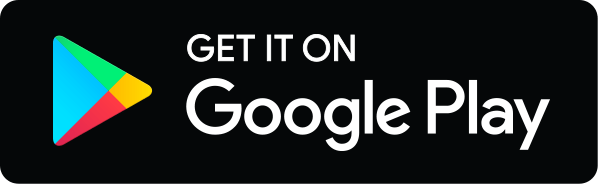
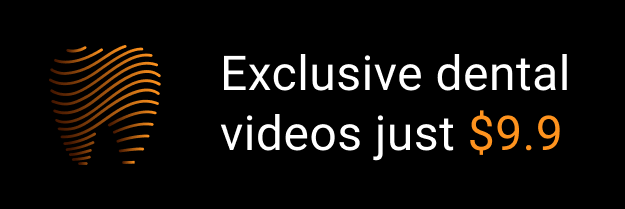