This article focuses on glass-ionomer cement (GIC) and its role in the clinical management of caries. It begins with a brief description of GIC, the mechanism of fluoride release and ion exchange, the interaction between GIC and the external environment, and finally the ion exchange between GIC and the tooth at the internal interface. The importance of GIC, as a tool, in caries management, in minimal intervention dentistry (MI), and Caries Management by Risk Assessment (CAMBRA) also will be highlighted.
This article will focus on glass-ionomer cement (GIC) and its role in the clinical management of caries. It begins with a brief description of GIC, the mechanism of fluoride release and ion exchange, the interaction between GIC and the external environment, and finally the ion exchange between GIC and the tooth at the internal interface. The importance of GIC, as a tool, in caries management, in minimal intervention dentistry, and Caries Management by Risk Assessment (CAMBRA) also will be highlighted.
The philosophy of minimal intervention density (MI) and the CAMBRA approach is gaining popularity worldwide as both integrate the new understanding that caries is a biofilm-related, multifactorial and lifestyle-associated bi-directional disease. There is now an acceptance that to manage caries disease effectively, it is important to move from the surgical approach to a combined surgical—medical approach, with special focus on engaging patients in changing their lifestyle. The following statement is a consensus of 165 experts, comprising of clinicians, scientists, educators, and health service managers from 15 countries, who gathered in Bangkok for a consultative meeting to discuss the MI philosophy (personal communication, 2009):
“The current surgical model of caries management does not fully address the multi-factorial nature of dental caries. This model often results in unnecessary removal of tooth structure, can impact negatively on general health and quality of life and can impose substantial cost.”
Glass-ionomer cement (GIC) is an important tool in the fight against caries. It can be thought of as a reservoir of fluoride and other ions in the oral cavity, a mechanical barrier that protects the tooth surface against bacteria; most importantly, it can provide a long-lasting seal under the most challenging clinical circumstances.
GIC is very versatile. It may be utilized as a definitive restorative material, a preparation liner, a restorative base material, a luting cement, or a fissure sealant. Recently, it was suggested that GIC also could be useful in the preventive arena as therapeutic coating. This new terminology is utilized to describe a material that can be painted on a susceptible surface and form a long-lasting coat to protect, both mechanically and chemically, against accumulation of plaque where patients are unable to render effective hygiene in certain parts of the oral cavity. Clinical examples of these applications will be given at the end of the article.
Historical perspective
There is a spectrum of tooth-colored restorative materials, spanning from GIC at one end to the resin-based materials at the other end. Wilson and McLean introduced the GIC family of materials to the dental profession in 1988. The four families of acid–base materials used in dentistry are: silicate, zinc phosphate, polycarboxylate, and glass-ionomer. They all utilize acid and base components ( Table 1 ). It was over 100 years ago when silicate cements were introduced to dentistry as a restorative material. There was anecdotal evidence that silicate cements were an effective anticaries agent that was attributed mainly to the high level of fluoride release from the silicate material. The material’s solubility was high, however, and the search for its replacement led to the development of polycarboxylate cements, and eventually GIC. Currently, glass-ionomer is the only restorative material that is water-based and like silicate has an anticaries effect. Current esthetic high-strength GICs are considered to be durable restorative dental cements.
Acid-Base Cement | Acid | Base |
---|---|---|
Silicate | Calcium-fluoro-alumino-silicate | Phosphoric acid |
Zinc Phosphate | Zinc oxide | Phosphoric acid |
Polycarboxylate | Zinc oxide | Polyalkenoic acid |
Glass-Ionomer | Calcium-fluoro-alumino-silicate | Polyalkenoic acid |
How do GIC acquire their anticaries effect?
GICs are true acid–base cements where the base is the fluoroaluminosilicate glass powder and the liquid containing acid comes from the polyalkenoic family. In some glass formulations, the F rich phase of the glass can be visible ( Fig. 1 ) and physically distinct. Apart from the base and acid, the third major component is water, the major component of the liquid. The total water content of the set cement is somewhere between 11% and 24%. Being a true water-based material, GIC also is recognized as the only biological active restorative material that is currently available.
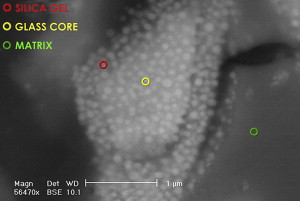
The precise glass composition varies from one material to another. Traditionally, GIC powder was based on calcium (Ca); however, there are several materials where the calcium has been substituted by strontium (Sr). This was done to impart radiopacity to the material. It is interesting to note that because of their similarity in polarity and atomic size, these two elements are interchangeable in the composition of GIC as well as hydroxyapatite (HAP). Some of the Ca in HAP can be substituted with Sr without any significant disadvantage. It is interesting to note that, at a population level, there is some evidence to show that Sr has anticariogenic properties. Curzon reported on the caries-reducing effect of drinking water containing strontium. He noted a reduction in DMFT in a population exposed to drinking water containing strontium. The importance of this information will become apparent in the discussion of a study relating to the internal remineralization technique, where both F and Sr from GIC were found to migrate from GIC to the soften dentine left at the base of cavities.
For restorative GIC, approximately 60% of the liquid is water, which plays an important role in the setting reaction and in the final structure of the set cement, as well as contributing to the observed biocompatibility and the well known ionic exchange. Water allows the acid–base reaction to occur and the migration of the various ions out of and into the matrix of the set cement. When the powder is brought into contact with the liquid, the acidity allows the matrix-forming cations, Ca or Sr and Al, to leach out of the glass. These will cross-link with the polyalkenoic chains to form metal polyacrylate salts, which form the matrix and solidify the mix.
How do GIC acquire their anticaries effect?
GICs are true acid–base cements where the base is the fluoroaluminosilicate glass powder and the liquid containing acid comes from the polyalkenoic family. In some glass formulations, the F rich phase of the glass can be visible ( Fig. 1 ) and physically distinct. Apart from the base and acid, the third major component is water, the major component of the liquid. The total water content of the set cement is somewhere between 11% and 24%. Being a true water-based material, GIC also is recognized as the only biological active restorative material that is currently available.
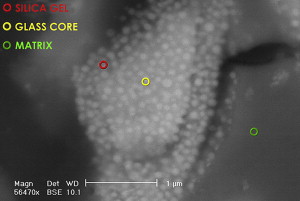
The precise glass composition varies from one material to another. Traditionally, GIC powder was based on calcium (Ca); however, there are several materials where the calcium has been substituted by strontium (Sr). This was done to impart radiopacity to the material. It is interesting to note that because of their similarity in polarity and atomic size, these two elements are interchangeable in the composition of GIC as well as hydroxyapatite (HAP). Some of the Ca in HAP can be substituted with Sr without any significant disadvantage. It is interesting to note that, at a population level, there is some evidence to show that Sr has anticariogenic properties. Curzon reported on the caries-reducing effect of drinking water containing strontium. He noted a reduction in DMFT in a population exposed to drinking water containing strontium. The importance of this information will become apparent in the discussion of a study relating to the internal remineralization technique, where both F and Sr from GIC were found to migrate from GIC to the soften dentine left at the base of cavities.
For restorative GIC, approximately 60% of the liquid is water, which plays an important role in the setting reaction and in the final structure of the set cement, as well as contributing to the observed biocompatibility and the well known ionic exchange. Water allows the acid–base reaction to occur and the migration of the various ions out of and into the matrix of the set cement. When the powder is brought into contact with the liquid, the acidity allows the matrix-forming cations, Ca or Sr and Al, to leach out of the glass. These will cross-link with the polyalkenoic chains to form metal polyacrylate salts, which form the matrix and solidify the mix.
Moisture control and the clinical performance of GIC
The setting reaction of GIC is a two-phase process. In the first phase, immediately after mixing, there is cross-linking of the poly-acid chains by either the Ca or Sr ions. This cross-linking during this first phase is not stable and can be easily affected by excessive water loss or gain. Clinically, this means that the restoration must be protected, against initial water contamination and desiccation, immediately after placement. In the second phase, within the solidified cement, the poly-acid chains are further cross-linked by trivalent Al ions. This second phase gives the material increased physical properties and reduced solubility.
In the set GIC, water molecules can be classified as either loosely bound or tightly bound. As the material matures, the ratio between the loosely bound and tightly bound water decreases, and the material will show increased in physical properties. This is important for two reasons. First, the loosely bound water is essential for ion release and uptake, and second, it is important to maintain the water balance right through the life of a GIC restoration. Early exposure to excess water during setting and desiccation, at any stage, will lead to poor clinical performance.
Ion exchange between GIC and the external environment
Because of the combined effect of release and uptake of ions, GIC is a rich reservoir of apatite forming ions such as fluoride, calcium, strontium, and phosphate. In the aqueous environment of the oral cavity, these are released through an ion exchange process with the environment. There is a natural exchange between Sr and Ca ions. As Sr leaves the set cement, an equivalent number of Ca ions from saliva enter the matrix of the cement to maintain electrolytic balance ( Fig. 2 ). Nicholson postulated that this leads to the hardening of the surface, and this was confirmed by Okada when it was found that the surface hardness of a GIC can increase by 39% after 40 days storage in natural saliva. This was demonstrated by immersing 24 hours-old buttons of strontium containing GICs in an artificial saliva solution, and then a distribution map of Ca was acquired using electron probe micro analysis (EPMA), which clearly showed that Ca was exchanged for Sr in the matrix of the surface layer of the GIC buttons (see Fig. 2 ). It was postulated that the increase in surface hardness was related to the exchange of Sr by Ca in the matrix; however, the mechanism involved has not yet been identified. This phenomenon means that an exposure to Ca-rich saliva is beneficial to the long-term clinical performance of GIC.
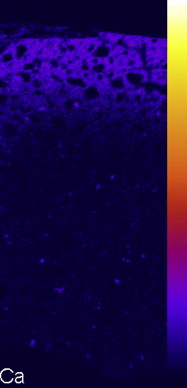
One of the major advantages of GIC is the long-term release of fluoride and other ions. This is characterized by an initial high peak that will decline rapidly to a lower level and sustained over a number of years. It has been shown that there is a topping-up effect with the material up-taking fluoride from external sources such as toothpaste and topical fluoride gel. Wilson studied this over a period of 20 months and found that these species still were being released at the end of the experimental period, although at a diminished rate. This fluoride release also can suppress the acidogenicity of the biofilm. From the above observations and what is known about the importance of fluoride in the demineralization and remineralization process, one can understand the protective effect that GIC has on tooth structure in its immediate vicinity. As the level of fluoride, and other ions, in GIC can be recharged, this class of material can be used as a reservoir for fluoride and may act as a slow F release device. GIC also can provide protection by the combined effect of mechanical and chemical means. For example, GIC can be used successfully to protect fissures, and despite a lower retention rate than resin-based fissure sealants, there is evidence that it reduces caries incidence in permanent teeth. In addition, a long-term clinical trial reported an effective rate of 80.6%, and suggested traditional retention is not essential for caries prevention. This is probably due to the effect of the chemical protection the material provides, and the fact that GIC tends to fail cohesively, so there is always a remaining layer on the surface, even when GIC is no longer clinically detectable.
This ion exchange is not only restricted to fluoride. The essential elements in a GIC include Ca, or Sr, Al, Si, and F. After the completion of the setting reaction, a portion of these ions is available for transfer from the matrix to the surrounding environment. As shown in ( Fig. 3 ), the level of release of Sr far exceeds that of F or Al ions. It is well accepted that the release is caused by an exchange process, so it is unlikely to adversely affect the clinical performance of the material.
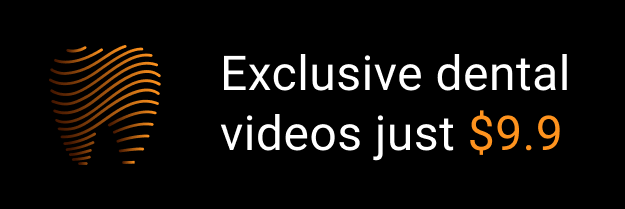