Introduction
The purpose of this study was to compare galvanic currents generated by different combinations of commonly used brackets and archwires.
Methods
As-received stainless steel, nickel-titanium, and beta-titanium wires were coupled to stainless steel and titanium brackets in an artificial saliva medium. The galvanic current and amount of charge transferred for each pair were monitored with a zero resistance ammeter for 10 hours.
Results
Two-way analysis of variance (ANOVA) showed a significant difference in charge and galvanic currents when factored for type of bracket ( P <0.001), but no significant difference between them when factored by type of wire ( P >0.05). Specifically, a brazed stainless steel bracket was significantly greater in charge transferred and 10-hour galvanic current than metal injection molded stainless steel and titanium brackets ( P <0.001), which were not different from each other ( P >0.05).
Conclusions
The method of bracket manufacturing might be of equal or more relevance to galvanic corrosion susceptibility than bracket composition.
Technologic advances in wire selection and bracket design have improved treatment efficiency and allowed longer intervals between appliance adjustments. This implies that wires will remain in the mouth for a correspondingly longer time, where they are subject to electrochemical reactions, mechanical forces of mastication, and generalized wear. These various phenomena can cause different forms of corrosion, an electrochemical process that results in the loss of the essential properties of a metal. Several types of corrosion can take place through 1 of 2 mechanisms. Metal ions can be deposited directly into the saliva, or the protective surface film on the metal can progressively dissolve. Galvanic corrosion refers to the electrochemical reactions that occur when 2 different metals, immersed in the same solution, are electrically coupled to each other. When this happens, both metals are polarized, with 1 metal generally becoming a cathode and the other an anode. Compared with its uncoupled rate, the anode experiences an increased corrosion rate, whereas the corrosion rate of the cathode decreases.
Most orthodontic materials are metallic. For example, bands, brackets, facebows, archwires, many types of retainers, and other adjunctive appliances and devices are made of different metals and, therefore, are subject to galvanic and other types of corrosion. Not surprisingly, several studies have observed corrosion on the surface of various orthodontic appliances. Most research on corrosion has involved the measurement of ion release, with nickel as the major focus of attention, or the corrosion properties of individual materials. Fewer studies have investigated galvanic corrosion with various combinations of brackets and wires.
There are several consequences of corrosion in orthodontics. It has been stated that corrosion is perhaps the single most relevant property of a casting alloy to its biologic safety, since the release of metal ions—ie, corrosion—is a necessary condition for an adverse biologic reaction. This is most likely true for orthodontic alloys as well. Corrosion of orthodontic alloys will result in the release of the respective metal constituents. In terms of nickel release, it appears that orthodontic treatment does not induce nickel sensitivity in patients unless they have a history of cutaneous piercings or of allergic reactions. Still, for patients in these categories, and it was estimated that 4000 orthodontics patients per year develop nickel (or chromium) sensitivity in North America, minimizing elemental release should be a goal in materials development by manufacturers and in material selection by clinicians. Among other consequences of corrosion, it has been proposed to be among the factors associated with clinical wire fracture, and it increases the surface roughness of the metal, resulting in increased friction. With these considerations, the purpose of this study was to focus specifically on galvanic current by using different combinations of brackets and archwires that are commonly used in most orthodontic practices.
Material and methods
Three compositional types of wires and brackets, respectively, were the subject of this research. All similar specimens were from the same batch number and tested in “as-received” condition from the manufacturers. The brackets consisted of a brazed stainless steel bracket (Orthos, Ormco, Glendora, Calif), an injection-molded stainless steel bracket (Equilibrium 2, Dentaurum, Ispringen, Germany), and an injection-molded titanium bracket (Equilibrium Ti, Dentaurum). All 3 types were maxillary central incisor brackets. The wires were composed of stainless steel (Tru-Arch, Ormco), nickel-titanium (NiTi, Ormco), and beta-titanium (TMA, Ormco), each with a diameter of 0.016 in.
All wires were sectioned at the midline marker, and each half arch was placed in a 3.8-cm long polyvinyl chloride pipe and embedded in acrylic (Sampl-Kwick, Buehler, Lake Bluff, Ill). The ends of the wire extended out of the acrylic, with 1 end to be used for connection to the electrochemical measuring equipment and the other submerged in solution. The submerged end was painted with nail polish to expose only 9 mm of wire, simulating the average width of a maxillary central incisor. The brackets were individually connected to a 15.2-cm long strand of insulated copper wire, with 1 end flattened to provide a broader contact area. This end was secured to the pad of each bracket with sticky wax, and the wax covered the entire pad to isolate the face of the brackets. The remaining exposed copper wire was insulated with nail polish, and the electrical continuity of the connection was ensured with a multi-meter. The solution used in this study was aerated phosphate buffered saline solution (pH = 7.4) at room temperature (21°C ± 1°C). The phosphate buffered saline solution (Fisher Scientific, Houston, Tex) had the following concentration: 137 mmol/L of sodium chloride, 2.7 mmol/L of potassium chloride, and 10 mmol/L phosphate ion and has been used in previous orthodontic wire-corrosion research.
Electrochemical measurements were made with a potentiostat (Gamry Instruments, Warminster, Pa) connected to a desktop computer. The study was divided into 2 phases. In phase I, standard corrosion parameters for each bracket and wire type were measured individually. The electrochemical cell consisted of a saturated calomel reference electrode and a graphite counter electrode. The following protocol was used.
- 1.
The open circuit potential (OCP) was monitored for 10 hours. This time was chosen to be consistent with testing in phase II.
- 2.
A potentiodynamic scan was conducted at 5 mV per second from 300 mV below to 700 mV above each specimen’s OCP.
The corrosion current was calculated from the generated data. Four specimens of each bracket and wire type were prepared and tested, with fresh solution used each time.
Phase II was the main focus of this research and involved measuring the galvanic current between each possible combination of bracket and wire. The potentiostat was set up as a zero resistance ammeter, which electrically couples the 2 metals of interest and measures the current flowing between them. To determine the proper electrical connection for each bracket-wire pair, the OCP data from phase I were used to assign the cathode (counter-electrode lead) to the more electropositive metal and the anode (working electrode) to the more electronegative metal. The reference electrode for phase II was also a saturated calomel electrode. The galvanic current of the couple was monitored for 10 hours. Ten hours was chosen based on a pilot study that showed that galvanic current plateaus after a few hours. This time is also consistent with other dental galvanic current research. The total charge was calculated by integrating the area under the current vs time curve. Each combination was tested 4 times with new specimens each time.
Statistical analysis
Current and charge data were not normalized for surface area as is the conventional practice. This was done primarily because measuring the current flowing between a single bracket and a corresponding segment of wire was desired. Additionally, although the wires were standardized to a specific size, standardization of bracket area is not feasible, and measuring the surface area of the brackets is difficult. The measured and calculated corrosion parameters in phase I were subject to 1-way analysis of variance (ANOVA) followed by the Tukey post-hoc test when indicated (SPSS, Chicago, Ill). Two-way ANOVA and the Tukey post-hoc tests were used for phase II data, with type of wire and bracket as factors. The significance level was set at α = 0.05.
Results
The means and standard deviations for the OCP at 10 hours and corrosion current for each type of wire and bracket measured in phase I are shown in Table I . The OCP of the stainless steel wire was significantly greater than all other materials tested ( P <0.05). There were no significant differences between the other 5 materials ( P >0.05). Potentiodynamic curves of each wire and bracket are shown in Figure 1 . With regard to corrosion current, the stainless steel brackets (Equilibrium 2 and Orthos) had significantly higher values than did all other materials ( P <0.05). There were no significant differences between the remaining 4 materials with respect to corrosion current ( P >0.05).
Material | OCP at 10 hours (mV vs SCE) | Corrosion current (nA) |
---|---|---|
Wires | ||
NiTi | −41 ± 21 | 4.0 ± 0.9 |
Beta-titanium | −37 ± 26 | 6.4 ± 3.3 |
Stainless steel | 170 ± 49 | 2.9 ± 0.3 |
Brackets | ||
Equilibrium Ti | −58 ± 27 | 31.3 ± 30.3 |
Equilibrium 2 | −24 ± 36 | 74.6 ± 17.4 |
Orthos | −25 ± 22 | 92.4 ± 15.2 |
∗ The OCP of the stainless steel wire was significantly greater ( P <0.05) than all other materials. The corrosion currents of the stainless steel brackets (Equilibrium 2 and Orthos) were significantly greater ( P <0.05) than all other materials.
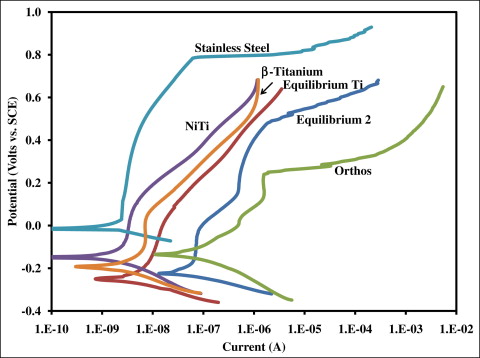
In phase II of the study, the galvanic current between each possible combination of wire and bracket was measured for 10 hours. Typical initial segments of current vs time for stainless steel individually coupled to the 3 brackets are shown in Figure 2 . The galvanic corrosion was greatest upon immersion in the electrolyte and then decreased over time. The other combinations had similar curves but are not shown for clarity. The means and standard deviations of calculated charge and galvanic current measured at 10 hours for each combination of wire and bracket are shown in Table II . Two-way ANOVA showed a significant difference in charge and galvanic current when factored for type of bracket ( P <0.001). Post-hoc Tukey tests subsequently showed a significant difference ( P <0.001) between galvanic couples with the Orthos brackets vs couples with either Equilibrium Ti or Equilibrium 2 brackets. There was no significant difference between galvanic couples when the brackets were Equilibrium Ti or Equilibrium 2 ( P = 0.97 for charge, P = 0.96 for galvanic current). With regard to the 3 wires (beta-titanium, nickel-titanium, and stainless steel), there were no significant differences between them when factored by type ( P = 0.14 for charge, P = 0.68 for galvanic current). Additionally, there was no significant interaction between wire and bracket for either measure ( P >0.05). Figure 3 shows a comparison of galvanic currents measured from hours 8 to 10 for all wire-bracket couples. In general, the galvanic currents when the wires were coupled with the Orthos bracket were much greater than when the wires were coupled with the other 2 brackets.
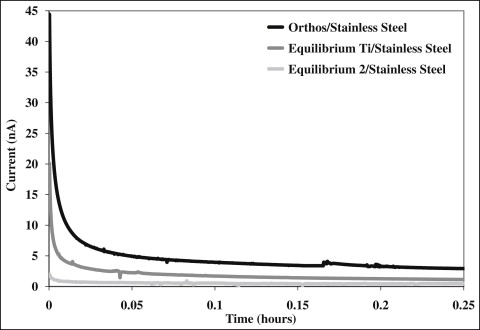
Combination | Charge (μC) | Galvanic current at 10 hours (pA) | |
---|---|---|---|
Wire | Bracket | ||
Beta-titanium | Equilibrium Ti | 3.6 ± 3.0 | 144 ± 202 |
Beta-titanium | Equilibrium 2 | 7.9 ± 4.6 | 163 ± 174 |
Beta-titanium | Orthos | 37.9 ± 25.0 | 1112 ± 680 |
NiTi | Equilibrium Ti | 3.4 ± 3.3 | 120 ± 177 |
NiTi | Equilibrium 2 | 6.2 ± 4.6 | 267 ± 477 |
NiTi | Orthos | 18.4 ± 13.5 | 625 ± 567 |
Stainless steel | Equilibrium Ti | 11.3 ± 3.6 | 226 ± 96 |
Stainless steel | Equilibrium 2 | 7.7 ± 6.5 | 192 ± 82 |
Stainless steel | Orthos | 38.8 ± 20.6 | 955 ± 653 |
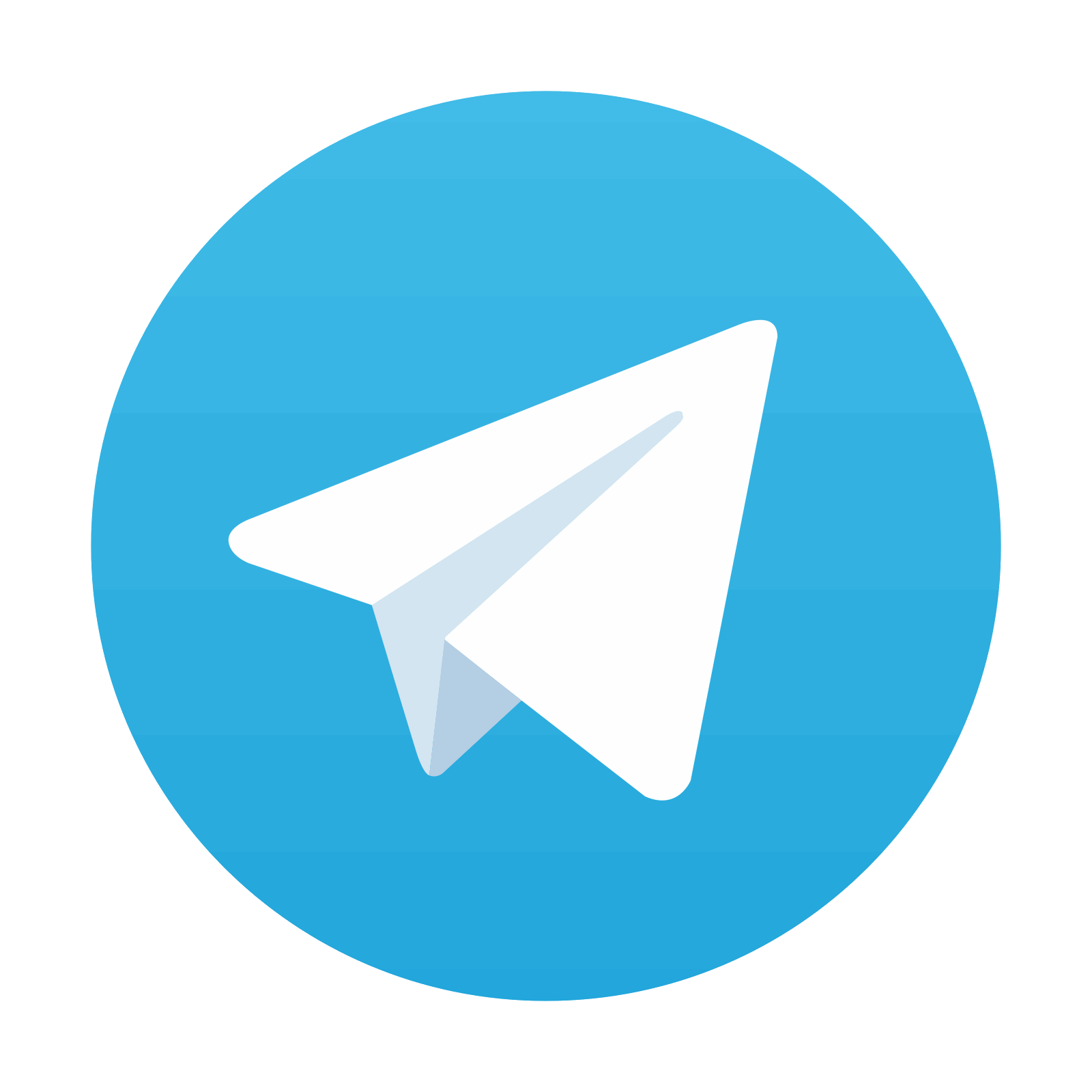
Stay updated, free dental videos. Join our Telegram channel

VIDEdental - Online dental courses
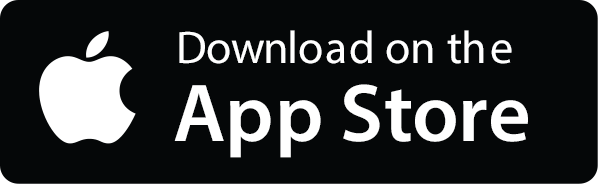
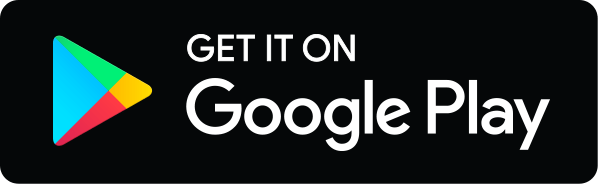
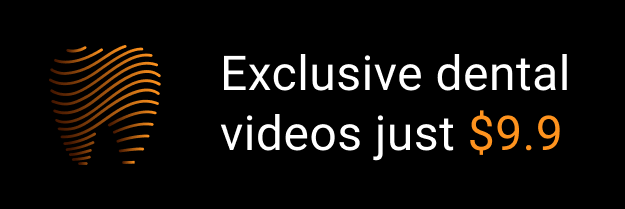