Abstract
The aim of this study was to measure the heat generated when using a self-drilling miniscrew at speeds of 50, 100, 150, and 250 rpm. Specimens were classified into two groups: in the thin group the cortical bone thickness was 1.2 ± 0.02 mm on average and in the thick group it was 2.0 ± 0.03 mm on average. The thin group was used to model maxillary bone and the thick group to model mandibular bone in humans. The temperature in the 1.2-mm and 2.0-mm cortical bone specimens was measured according to revolution speed. As the revolution speed increased, the temperature significantly increased in both bone thicknesses. The temperature increased significantly more in the thicker cortical bone. The temperature increase in the 2.0-mm thick bone at 250 rpm exceeded 10 °C, regarded as the threshold for bone damage in this study; other temperature increases were below this threshold. Installing self-drilling screws at high speeds with an implanter is not recommended; low speeds of less than 150 rpm should be used.
Anchorage control in orthodontic treatment has a major influence on the treatment period and results. Extraoral appliances, such as headgear, have been used chiefly in clinics, but if tooth anchorage is insufficient and tooth movement occurs, optimal treatment results cannot be achieved. The orthodontic miniscrew can be used to address these problems and obtain absolute anchorage .
Miniscrews can be fixed by self-tapping and self-drilling . Self-tapping requires the drilling of a pilot hole and then screwing in the implant body. Self-drilling only requires screwing in the implant body, no pilot hole is required. Self-drilling has the advantage of greater work efficiency since the working time can be reduced.
There has been much research concerning the stability of miniscrews owing to their poor success rate. Factors affecting the initial stability of miniscrews include the diameter , length, shape of the thread ridge, thread pitch, shape of the abutment , postoperative infection , root proximity , excessive orthodontic force, and amount of frictional heat generated during drilling. H olmgren et al. determined that the widest diameter implant was not necessarily the best choice when considering stress distribution to the surrounding bone, and the success rate did not necessarily increase with size. C hen et al. showed that an increased failure rate with drilling implants was associated with local inflammation in the surrounding soft tissue. K uroda et al. revealed that failures occurred between the drilling site and the root of the tooth.
B enington et al. drilled into bovine mandibular bone, and measured the temperature change in the surrounding bone. The temperature increased significantly. This temperature increase can result in heat-induced bone injury. No previous report has investigated the generation of heat by self-drilling screws. In clinics, self-drilling screws are usually placed without irrigation, and it is clinically important to know the limits of revolution speed while not using irrigation. The authors measured the heat generated when installing self-drilling screws at revolution speeds of 50, 100, 150, and 250 rpm, using pig rib bone as a model.
Material and methods
The authors used self-drilling screws (Biodent Co., Ltd., Tokyo, Japan), 1.6 mm in diameter and 8.0 mm long ( Fig. 1 ). Frozen pig rib bone was stripped and cut into 2-cm long sections. The cortical bone thickness of the specimens was measured using digital vernier calipers. The specimens were divided into thick and thin groups. On average, cortical bone thickness was 1.2 ± 0.02 mm in the thin group and 2.0 ± 0.03 mm in the thick group. The 1.2-mm thick bone was used as a model of human maxillary bone, and the 2.0-mm thick bone was used as a model of human mandibular bone.

Specimens were defrosted to room temperature (25 ± 0.69 °C, on average), and were kept from drying out to reproduce clinical conditions when drilling in a living body. To place the screw perpendicular to the bone surface, the specimens were fixed so that the bone surface was parallel to the floor. A thermosensor (SD16 #04, Shimaden, Inc., Tokyo, Japan) was positioned on the bone surface 0.5 mm away from the implant drilling site. Silicone heat-transfer compound (COH-4000, Wide Works, Inc., Tokyo, Japan) was applied between the thermosensor and the bone surface to facilitate heat conduction ( Fig. 2 ). The self-drilling screw was then screwed into the bone using an implanter (Implanter, Densply, Tochigi, Japan).

The revolution speeds were fixed at 50, 100, 150, and 250 rpm. The temperature on the bone surface was measured 10 times and averaged at each revolution speed. No irrigation was used during drilling. One operator performed all the drilling procedures. The initial temperature, maximum recorded temperature, and change in temperature from the initial temperature were measured for each thickness and revolution speed.
The Sheffe test was used to compare same-thickness groups. The unpaired t -test was used to evaluate the influence of cortical bone thickness. All statistical analyses were carried out using SPSS software (SPSS Japan, Inc., Tokyo, Japan).
Results
The mean initial temperature, mean maximum recorded temperature, and mean change from the initial temperature are shown in Table 1 . For the 1.2-mm thick bone, the changes in temperature at 50, 100, 150, and 250 rpm were 2.6, 4.4, 4.5, and 4.7 °C, respectively. For the 2.0-mm thick bone, the changes in temperature at 50, 100, 150, and 250 rpm were 5.0, 6.9, 8.0, and 11.1 °C, respectively. The changes in temperature for 1.2-mm thick bone between 50 and 100 rpm ( P < 0.001), between 50 and 150 rpm ( P < 0.001), and between 50 and 250 rpm ( P < 0.001) were statistically significantly different. The changes in temperature between all four drilling speeds in the 2.0-mm thick bone showed statistically significant differences ( P < 0.001). As the revolution speed increased, so did the change in temperature. The changes from initial temperatures according to cortical bone thickness for each revolution speed are compared in Fig. 3 . There were significant differences between the 1.2-mm thick and 2.0-mm thick bones at all revolution speeds ( P < 0.001).
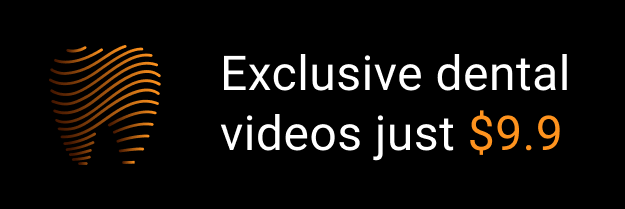