Introduction
The force applied to the teeth is a variable of orthodontic treatment that can be controlled. Poor control of the applied force can lead to adverse biologic effects as well as undesirable tooth movements. The selected archwire-bracket combination is a primary determining factor in the force level applied to a tooth during orthodontic treatment. The aim of this research was to use an experimental biomechanical setup to measure forces generated during complex orthodontic tooth movements with various archwire-bracket combinations.
Methods
The materials consisted of 3 types of 0.022-in slot orthodontic brackets: (1) conventional brackets (Victory Series [3M Unitek, Monrovia, Calif] and Mini-Taurus [Rocky Mountain Orthodontics, Denver, Colo]), (2) self-ligating brackets (SmartClip [3M Unitek] and Time3 [American Orthodontics, Shegoygan, Wis]), and (3) a conventional low-friction bracket (Synergy [Rocky Mountain Orthodontics]); and 4 archwire types: (1) 0.012-in stainless steel (3M Unitek), (2) 0.0155-in coaxial (Advanced Orthodontics [Näpflein, Düsseldorf, Germany]), (3) 0.012-in Orthonol (Rocky Mountain Orthodontics), and (4) 0.012-in Thermalloy (Rocky Mountain Orthodontics). Stainless steel ligatures and elastomeric rings were used. The materials were used in different combinations in a simulated malocclusion that represented a maxillary central incisor displaced 2 mm gingivally (x-axis) and 2 mm labially (z-axis).
Results
The lowest forces were measured when the brackets were combined with either the coaxial or the Thermalloy archwires; the forces ranged from 3.4 ± 0.2 to 0.7 ± 0.1 N in the x-axis direction, and from 4.5 ± 0.3 to 0.5 ± 0.1 N in the z-axis direction. The highest forces were measured in combination with stainless steel archwires; the forces ranged from 6.3 ± 0.3 to 3.0 ± 0.1 N in the x-axis direction, and from 6.3 ± 0.3 to 1.7 ± 0.1 N in the z-axis direction.
Conclusions
We recommend 0.0155-in coaxial and 0.012-in Thermalloy archwires for leveling and alignment. Elastomeric rings, when used with conventional brackets, increased the force applied to the teeth.
The concept of optimal force level has been a controversial subject over the years. A consensus advocates the use of light forces or, more specifically, the lightest force capable of achieving orthodontic tooth movement. Contrary to many variables influencing orthodontic treatment that cannot be controlled, such as growth and tissue response, the force applied to the tooth during orthodontic treatment should be a controllable variable. Inability to control the applied force could not only lead to adverse biologic effects but also result in undesirable tooth movements. Von Böhl et al found that high forces did not move teeth faster than low forces, but teeth with higher forces had more areas of hyalinization. Gonzales et al found generally that lighter forces produced greater tooth movement, especially after 14 and 28 days of the initial force applications. Heavier forces produced greater root resorption. Yee et al concluded from their clinical study on the rate of canine retraction that initial tooth movement would benefit from light forces, since there was no significant difference between the rates of canine retraction with light and heavy forces, but heavier forces increased the anchorage loss and the rotational effect on the retracted canine. From a mechanical point of view, their study and that of Badawi et al evaluating 3-dimensional force measurements during high canine correction showed that different force systems have a wide influence on resulting tooth movements.
Both the behavior of elastic materials and mechanical factors in the response of the teeth must be considered in the design of an orthodontic appliance. Orthodontists should build a solid background in the biomechanical principles that control the effects of orthodontic appliances. The selected archwire-bracket combination is a primary determining factor in the force level applied to a tooth during orthodontic treatment. Self-ligating brackets are increasingly replacing conventional brackets for many reasons; a major reason is their suggested reduced friction compared with conventional brackets, especially when coupled with smaller archwires used in the initial leveling and alignment stage. However, since the introduction of nickel-titanium alloys into orthodontics, there is an increased tendency to use larger continuous archwires early in treatment.
Because it is difficult to measure directly the stresses and strains in the periodontal ligament of loaded teeth, only measuring the forces that are applied directly to teeth with known root surface areas can provide an estimate of these parameters. In this respect, laboratory orthodontic studies strive to help orthodontists design and select orthodontic mechanics that are both efficient and biologically safe.
This study was designed to measure the forces generated during complex orthodontic tooth malalignment correction with various small cross-sectional archwire-bracket combinations by using an experimental biomechanical approach.
Material and methods
The study examined 3 types of orthodontic brackets: 2 conventional ligating brackets (Victory Series [3M Unitek, Monrovia, Calif] and Mini-Taurus [Rocky Mountain Orthodontics, Denver, Colo]), 2 self-ligating brackets (SmartClip, a passive self-ligating bracket [3M Unitek] and Time3, an active self-ligating bracket [American Orthodontics, Sheboygan, Wis]), and a conventional low-friction bracket with a special design (Synergy; Rocky Mountain Orthodontics). All brackets had a nominal 0.022-in slot size. The brackets were combined with 4 archwires: 0.012-in stainless steel (3M Unitek), 0.012-in Orthonol (Rocky Mountain Orthodontics), 0.012-in Thermalloy (Rocky Mountain Orthodontics), and 0.0155-in coaxial (Advanced Orthodontics; Näpflein, Düsseldorf, Germany). Archwires were combined with the conventional brackets with both stainless steel ligatures of 0.010-in size (Advanced Orthodontics) and elastomeric rings with an outer diameter of 2.94 mm (3M Unitek). All material combinations tested in the study are listed in Table I .
Bracket type | Bracket width (central incisor) | Bracket width (lateral incisor) | Type of ligation | Type of wire | Group observations | Total observations |
---|---|---|---|---|---|---|
Mini-Taurus | 3.6 mm | 3.1 mm | Stainless steel ligation Elastic ligation |
|||
Victory Series | 3.6 mm | 2.9 mm | Stainless steel ligation Elastic ligation |
0.012-in stainless steel 0.0155-in coaxial |
||
Synergy | 3.4 mm | 3.4 mm | Stainless steel ligation Elastic ligation |
0.012-in Orthonol 0.012-in Thermalloy |
20 | 640 |
Smart-Clip | 3.8 mm | 3.3 mm | Self-ligation | |||
Time3 | 2.5 mm | 2.4 mm | Self-ligation |
Resin replicas (Palavit G 4004; Heraeus Kulzer, Hanau, Germany) were constructed from a Frasaco model (Frasaco, Tettnang, Germany) of a normally aligned maxillary arch. The right central incisor was removed from the resin model to allow for placement of a sensor of the experimental setup, the custom-made orthodontic measurement and simulation system (OMSS) developed at the University of Bonn.
Brackets were bonded from second premolar to second premolar on the resin models with a cyanoacrylate adhesive. A jig was used to standardize the bonding process of the right central incisor bracket to a bracket holder that was attached to a force sensor of the OMSS.
The self-ligating brackets in this study were used in the closed position. For the conventional brackets, the stainless steel ligatures were tied by using a needle holder; the ligature was first tightened around the bracket wings and then loosened by 1 turn to allow free movement of the archwire. Also, the archwires were not cinched back to allow free movement of the archwire throughout the test. The elastomeric ligatures used were positioned with a needle holder, with a 3-minute waiting period before testing, to allow a reproducible amount of stress relaxation to occur, as recommended by Henao and Kusy.
The OMSS comprises 2 force-moment sensors capable of registering forces and moments in the 3 planes of space simultaneously. The 2 sensors are mounted on motor-driven positioning tables that can move freely in 3 planes of space. Commands regarding the conditions of the experiment are given to the OMSS through a personal computer. Two microcomputer-based sensor electronics deliver the digital output of the force moment vectors to the personal computer, where the resultant force-deflection curves are recorded. The whole mechanical assembly of the OMSS is built in a temperature-controlled chamber, which is especially important when testing temperature-dependant alloys, such as nickel-titanium.
To prepare the setup for measurements, the resin model was mounted on the OMSS table, and the bracket holder with the bracket of the right central incisor bonded to it was fixed to the left force sensor ( Fig 1 ). The sensor was then adjusted so that the bracket was in the correct position in the prepared space in the resin model. The whole assembly now simulated the originally aligned arch. The malocclusion simulated in this study comprised a coupled incisogingival movement of 2 mm and labiolingual movement of 2 mm in 0.01-mm increments. The intrusion-extrusion movement coincided with the global x-axis, whereas the labiolingual movement was represented by the z-axis of the setup. Thus, the OMSS was set to move the maxillary right central incisor from the initial position 2 mm gingivally and 2 mm labially, and then to move back to the initial position in 0.01-mm increments. Before starting the measurements, the initial forces and moments exerted to the bracket and recorded by the sensors were adjusted as close to zero as possible. The initial bracket position was reproduced before each measurement.
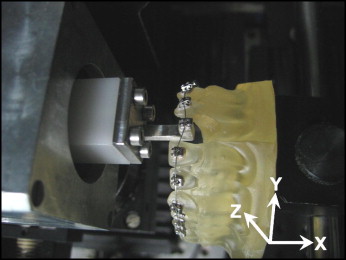
Each bracket-archwire combination was tested 20 times, for a total of 640 measurements. During testing of both Orthonol and Thermalloy wires, the temperature was kept at 37°C (±1°C).
The maximum absolute values of the forces generated by bracket displacement in the 2 directions were recorded directly with the OMSS software. Descriptive statistics including means, standard deviations, and maximum and minimum values were calculated for each archwire-bracket combination and then statistically analyzed with 2-way analysis of variance (ANOVA) followed by 1-way ANOVA.
Results
Descriptive statistics of the maximum forces exerted along the x-axis, representing the intrusion-extrusion movement, and the z-axis, representing the labiolingual movement, are presented in Tables II and III , respectively. Figures 2 and 3 show bar graphs of the different bracket-wire combinations sorted by wire and bracket type, for steel ligation ( Fig 2 ) and elastic ligation ( Fig 3 ).
Wire type | n | Bracket type | |||||||
---|---|---|---|---|---|---|---|---|---|
Mini-Taurus steel ligation | Mini-Taurus elastic ligation | Victory Series steel ligation | Victory Series elastic ligation | Synergy steel ligation | Synergy elastic ligation | Smart-Clip | Time3 | ||
Steel | 20 | 4.3 ± 0.1 | 5.9 ± 0.2 | 3.8 ± 0.2 | 6.3 ± 0.3 | 3.5 ± 0.2 | 3.1 ± 0.2 | 3.9 ± 0.2 | 3.0 ± 0.1 |
Coaxial | 20 | 1.3 ± 0.1 | 3.4 ± 0.2 | 1.4 ± 0.2 | 4.6 ± 0.2 | 1.0 ± 0.1 | 1.1 ± 0.1 | 1.1 ± 0.1 | 0.7 ± 0.1 |
Orthonol | 20 | 1.8 ± 0.1 | 3.6 ± 0.3 | 1.5 ± 0.1 | 3.7 ± 0.3 | 1.6 ± 0.2 | 1.2 ± 0.1 | 1.6 ± 0.1 | 1.2 ± 0.2 |
Thermalloy | 20 | 1.4 ± 0.2 | 3.7 ± 0.2 | 1.1 ± 0.2 | 4.1 ± 0.3 | 1.1 ± 0.1 | 1.0 ± 0.1 | 1.2 ± 0.1 | 1.0 ± 0.1 |
Wire type | n | Bracket type | |||||||
---|---|---|---|---|---|---|---|---|---|
Mini-Taurus steel ligation | Mini-Taurus elastic ligation | Victory Series steel ligation | Victory Series elastic ligation | Synergy steel ligation | Synergy elastic ligation | Smart-Clip | Time3 | ||
Steel | 20 | 3.5 ± 0.2 | 5.7 ± 0.2 | 3.3 ± 0.2 | 6.3 ± 0.3 | 2.2 ± 0.1 | 2.4 ± 0.1 | 3.5 ± 0.1 | 1.7 ± 0.1 |
Coaxial | 20 | 1.3 ± 0.1 | 4.0 ± 0.2 | 1.7 ± 0.2 | 5.0 ± 0.2 | 0.5 ± 0.1 | 0.7 ± 0.1 | 1.1 ± 0.1 | 0.4 ± 0.1 |
Orthonol | 20 | 1.6 ± 0.2 | 4.2 ± 0.2 | 1.6 ± 0.1 | 4.7 ± 0.4 | 0.9 ± 0.2 | 0.8 ± 0.1 | 1.2 ± 0.2 | 0.9 ± 0.2 |
Thermalloy | 20 | 1.6 ± 0.2 | 4.4 ± 0.2 | 1.0 ± 0.2 | 4.5 ± 0.3 | 0.7 ± 0.1 | 0.7 ± 0.1 | 1.0 ± 0.1 | 0.7 ± 0.1 |
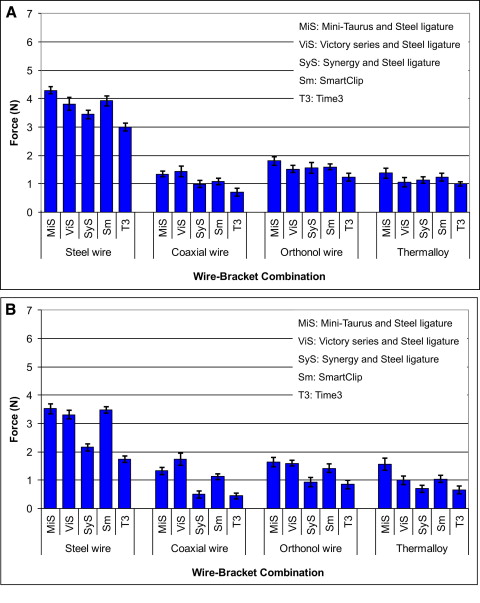
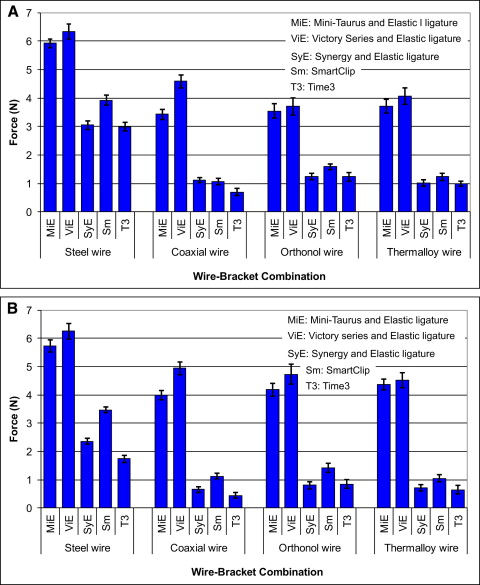
The 2-way ANOVA ( Table IV ) indicated significant bracket type effect ( P ≤ 0.000) and wire type effect ( P ≤ 0.000) on the force level in the x-direction. The 2-way ANOVA ( Table IV ) indicated significant bracket type effect ( P ≤ 0.000) and wire type effect ( P ≤ 0.000) on the force level in the z-direction. The results also indicated an interaction between these 2 variables in both the x-direction ( P ≤ 0.000) and z-direction ( P ≤ 0.000).
Mean square | df | F | P value | |
---|---|---|---|---|
Force in x-direction | ||||
Brackets | 120.71 | 7 | 914.73 | ≤0.000 ∗ |
Wires | 215.11 | 3 | 1630.04 | ≤0.000 ∗ |
Brackets × wires | 1.84 | 21 | 13.91 | ≤0.000 ∗ |
Force in z-direction | ||||
Brackets | 208.73 | 7 | 662.46 | ≤0.000 ∗ |
Wires | 112.17 | 3 | 355.99 | ≤0.000 ∗ |
Brackets × wires | 2.07 | 21 | 6.58 | ≤0.000 ∗ |
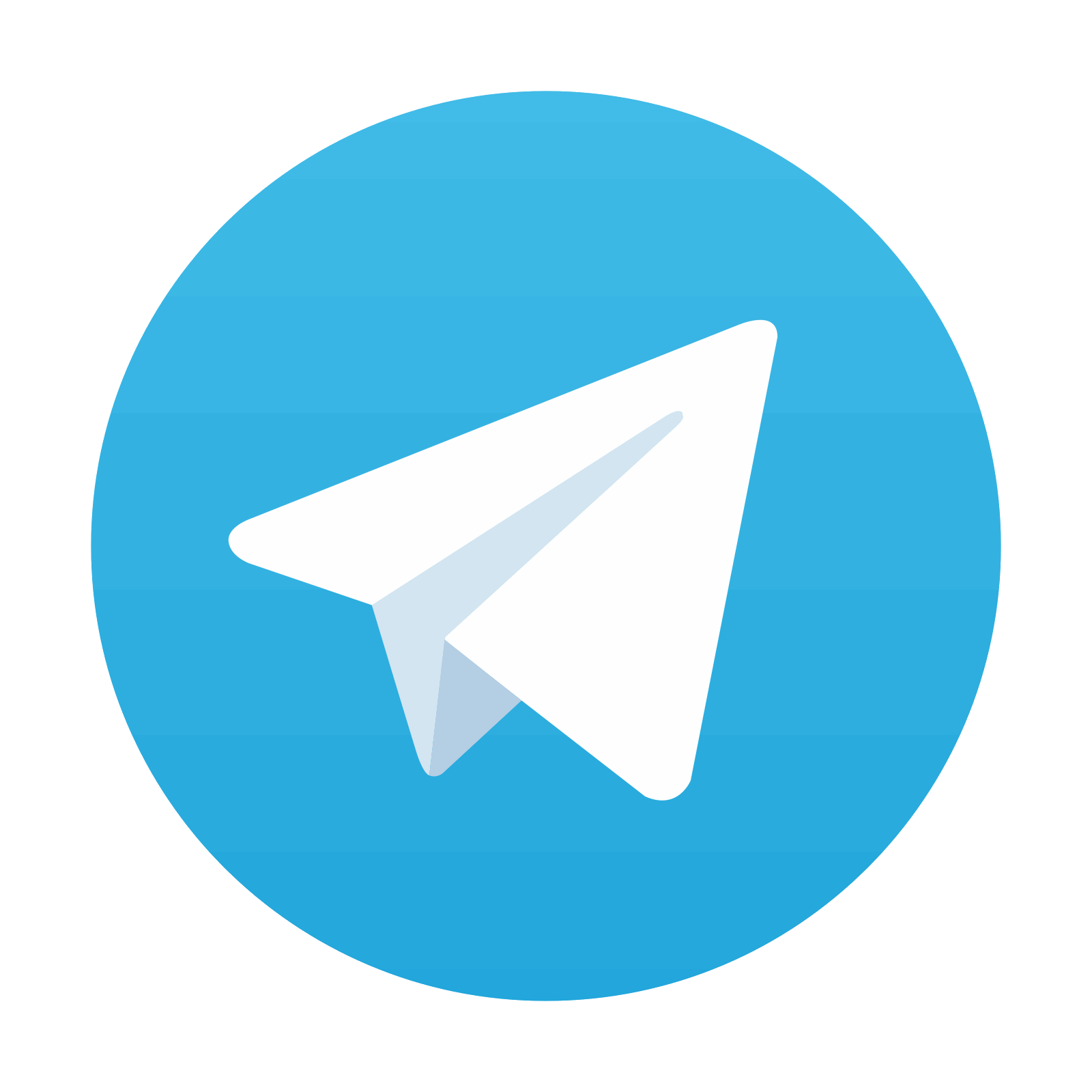
Stay updated, free dental videos. Join our Telegram channel

VIDEdental - Online dental courses
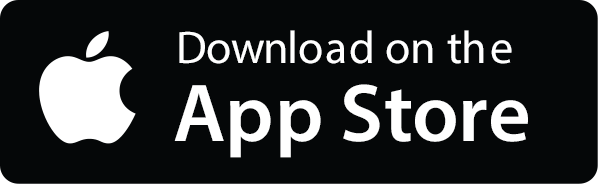
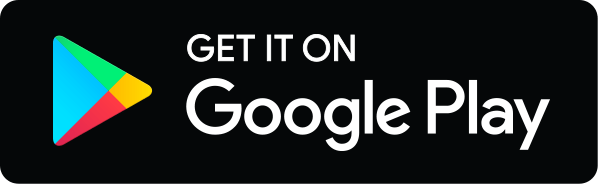
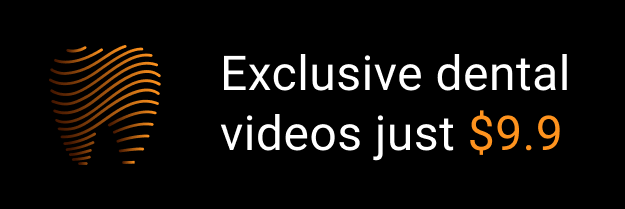