Introduction
The purpose of this study was to analyze pharyngeal airflow using both computational fluid dynamics (CFD) and fluid structure interactions (FSI) in obstructive sleep apnea patients before and after maxillomandibular advancement (MMA) surgery. The airflow characteristics before and after surgery were compared with both CFD and FSI. In addition, the presurgery and postsurgery deformations of the airway were evaluated using FSI.
Methods
Digitized pharyngeal airway models of 2 obstructive sleep apnea patients were generated from cone-beam computed tomography scans before and after MMA surgery. CFD and FSI were used to evaluate the pharyngeal airflow at a maximum inspiration rate of 166 ml per second. Standard steady-state numeric formulations were used for airflow simulations.
Results
Airway volume increased, pressure drop decreased, maximum airflow velocity decreased, and airway resistance dropped for both patients after the MMA surgery. These findings occurred in both the CFD and FSI simulations. The FSI simulations showed an area of marked airway deformation in both patients before surgery, but this deformation was negligible after surgery for both patients.
Conclusions
Both CFD and FSI simulations produced airflow results that indicated less effort was needed to breathe after MMA surgery. The FSI simulations demonstrated a substantial decrease in airway deformation after surgery. These beneficial changes positively correlated with the large improvements in polysomnography outcomes after MMA surgery.
Highlights
- •
Both CFD and FSI simulations indicated less effort was required to breathe after MMA surgery.
- •
FSI simulations showed a large decrease in airway deformation postsurgery.
- •
Airway simulation changes correlated positively with polysomnography improvements.
Obstructive sleep apnea (OSA) is a condition characterized by recurring episodes of partial and complete pharyngeal airway collapse during sleep. OSA has been reported to affect 9% to 24% of the middle-aged adult population and 1% to 4% of children. Severe cases of OSA can have many medical consequences due to the chronic reduction of air reaching the lungs. There is a wide range of side effects from OSA, from minor, such as dry mouth and fatigue, to life threatening, such as hypertension and stroke.
There are many treatment options for OSA. The use of the continuous positive airway pressure machine during sleep is considered the gold standard treatment for OSA. However, noninvasive, lifestyle modifications are often the first line of treatment used for OSA, especially in milder cases. Examples of treatment include weight management, cessation of smoking, exercise, sleep positional changes, and avoiding alcohol or sedatives before bedtime. Oral appliances are being used more and more to manage OSA because of their relative noninvasiveness and ease of use. In some patients with OSA, surgical intervention may be the only effective way to treat the condition. Although there are many possible surgical treatments, maxillomandibular advancement (MMA) surgery has been shown in many studies to be an effective, definitive treatment for OSA, leading to substantial reductions in apnea-hypopnea index numbers in sleep studies.
Studying the upper airway airflow is challenging due to the complexities of airway anatomy and physiology, the high cost of in-vitro studies, and the limitations of in-vivo studies. Because of this, fluid mechanics methodologies such as computational fluid dynamics (CFD) and fluid structure interactions (FSI) have been applied to airflow studies. These techniques can take the anatomy of an airway structure, the intrinsic properties of the structure and fluid, and the starting flow conditions to simulate how the fluid flows through the structure.
Studies have successfully validated the use of CFD to simulate airflow in the airway accurately. However, CFD assumes that the airway is a rigid, inflexible structure, whereas FSI allows for flexibility in the airway tube. This flexibility allows for a level of structure deformation that can greatly alter the airflow properties. The collapsible airway of OSA patients makes FSI a superior method of analyzing the airway compared with CFD.
The purpose of this pilot study was to simulate the pharyngeal airflow characteristics of patients with OSA before and after MMA surgery, using both CFD and FSI.
Material and methods
The adult patient pool of the private practice of an oral and maxillofacial surgeon (R.M.) in St. Louis, Missouri, was screened to identify appropriate subjects for this retrospective pilot study.
Inclusion criteria were (1) diagnosed with OSA from polysomnography, (2) having had MMA surgery, and (3) having follow-up polysomnography after surgery.
Exclusion criteria were (1) diagnosed with craniofacial syndromes and (2) having had surgical maxillary expansion.
Two patients meeting these criteria were selected. Postsurgery polysomnography tests and cone-beam computed tomography (CBCT) scans were done at least 6 months after surgery to allow swelling to go down, healing to be complete, and results to have stabilized. All CBCT scans were done with the patients in natural head position.
The surgical procedures were carried out by the same oral surgeon using the same surgical techniques. Virtual surgical planning was performed to predetermine the surgical movements. In both patients, a nonsegmental Le Fort I osteotomy advanced the maxilla and rotated it counterclockwise. Bilateral sagittal splint osteotomies advanced the mandible. Both jaws were rigidly fixated. In addition, bilateral partial inferior turbinectomies and septoplasties were done. The presurgery and postsurgery CBCT scans for both patients are shown in Figures 1-4 .




The presurgery and postsurgery CBCT scans for the subjects were imported into ITK-SNAP open-source software (version 3.4.0) as DICOM files. A volumetric image of the scanned area was generated, and the airway of interest was isolated. The upper airway was segmented from the nares through the nasal cavity and down the pharynx to the base of the epiglottis. Semiautomatic segmentation of the airway using a threshold-defined algorithm was performed. Manual segmentation was used to remove unwanted structures and capture any missed details. The oral cavity, tongue, and sinuses were not included. The epiglottis was removed to prevent artifacts. A transverse plane parallel to the ground at nares and base of the epiglottis delineated the start and end of the upper airway to be studied. Figure 5 shows the entire upper airway viewed in the software after segmentation in 3 dimensions, as well as from all 3 axes. Next, the walls of the model were separated into 2 parts, rigid wall and flexible wall. The flexible portion of the airway was superiorly bounded by a transverse plane through the posterior hard palate parallel to the floor. The inferior boundary of the elastic portion was the outlet or base of the epiglottis. All parts of the airway superior and anterior of the flexible portion were considered rigid . The segmented airway was exported as a solid 3-dimensional structure as a stereolithography file ( Figs 6 and 7 ).



The airway models were imported into SC/Tetra preprocessor software (version 12; Software Cradle, Osaka, Japan) to create the mesh models. Wrapping was performed to smooth the surface and reduce the discontinuity of the curvatures. The inlet, outlet, and wall boundaries were defined manually.
A hybrid mesh (tetrahedral mesh with prism layers on the walls) was generated with the base mesh size about 0.6 mm. The size was chosen to use the same protocol as did Huynh et al.
Computational meshes generated with the SC/Tetra preprocessor were imported into SC/Tetra solver (version 12; Software Cradle) for airflow simulation. A standard k-ε turbulence model was used to simulate turbulent airflow in the pharyngeal airway. The standard k-ε model was chosen because of its minimal computing power required for flow simulation. A fixed time step of 0.0005 seconds was used to ensure that the results converged for each step. The simulations were run for 4000 steps or 2 seconds each, which represents a single inhale cycle. Using a volumetric flow rate as a function of time, the flow rate behaved in a sinusoidal manner with a maximum flow rate of 166 ml per second occurring at the middle time point of 1 second. The transient flow rate was applied as a boundary condition at the inlet. For all models, the same flow rate was applied. The outlet boundary condition was set at a static pressure of 0 Pa. Material properties for the flexible region of the airway were density of 1200 kg per cubic meter, Young’s modulus of 7540 Pa, and Poison’s ratio of 0.45.
The simulations were run as CFD only for the entire airway and also as FSI simulations for both rigid and flexible regions. For the CFD-only simulations, both walls were treated as stationary walls with a no-slip condition. For the FSI simulations, the rigid wall was treated as a no-slip wall, but the flexible wall was used to map the fluid load onto the structure. This surface was allowed to move based on the structural response, so it was set as a wall whose velocity was based on the mesh velocity. Airflow simulations were completed using the SC/Tetra solver function on a personal computer with a Core i7 CPU 970 (3.2 GHz; Intel, Santa Clara, Calif) with 24 GB of RAM. SC/Tetra postprocessor software was used to analyze and visualize the fluid flow data.
The maximum magnitude of airflow velocity was measured in both patients before and after surgery. All measurements were recorded in the midsagittal plane of the airway. Velocity information was extracted using the SC/Tetra postprocessor. The Reynolds number, a measure of airflow turbulence, was calculated from these measurements at 3 areas (top, middle, and bottom of the flexible region) during the simulation.
The information regarding the pressure drop (Δp) was requested as an output from the solver. Δp values for the entire airway and for the flexible region for both patients before and after surgery were calculated. Airway resistance, R , was calculated for the patients before and after surgery. Resistance was calculated with Δp by using the equation R = Δp/Fua, where Fua is the mass flow rate. The mass flow rate can be derived from the equation Fua = Qρ, where Q is the volumetric flow rate and ρ is the density of air.
The FSI simulations were run using the SC/Tetra Abaqus module (version 12.0; Software Cradle) on the flexible region of the airway.
There are several ways to couple the CFD and finite element analysis solvers. In this study, weak coupling was used, where the governing equations of CFD and FEA were solved independently, and there was an exchange of information between the solvers after every time step. In addition, Gauss-Seidel coupling was applied, where the structural response was calculated based on the fluid solution from the CFD analysis, and the CFD solver calculated the solution referring to the solution from the FEA solver. The mesh deformation in the CFD solver was calculated based on the surface displacement information from FEA calculation. The mesh moving velocity was determined based on the surface displacement vector from the FEA. The effect of mesh deformation was considered using the arbitrary Lagrange Eulerian method. Since the mesh used in both disciplines can be different, first-order interpolations of both shapes and variables were used for the exchange of the information. The data transfer was handled in the software by using the cosimulation engine interface developed by Simulia. This eliminated the requirement of third-party software to handle data transfer between both softwares.
The coupling analysis was bidirectional fluid structure coupling. The CFD solver sends pressure information to the FEA solver, and the FEA solver sends displacement information back to the CFD solver. This type of coupling analysis is performed when the effect of the structure deforming on the flow is too large to ignore, and the deformation is affected mainly by the fluid force.
Identical measures were extracted in the same manner from the FSI simulations as the CFD simulations. Additionally, animations using color mapping to visualize the velocity and magnitude of deformation were generated for the entire airway. Finally, the airway was sliced in the transverse plane every 5 mm, and an animation superimposed the before and after simulations on each other to further help visualize the magnitude and direction of airway deformation for all simulations.
Results
Both patients had an overall increase in airway volume of about 40% to 50%. Most of the increase came from the flexible regions, which more than doubled for both patients. The rigid section showed a modest increase for patient 1, but patient 2 had a decrease in volume in this region. A summary of the airway volume change is given in Table I .
Total | Rigid | Flexible | |
---|---|---|---|
Patient 1 presurgery (mm 3 ) | 26,026 | 20,760 | 5,266 |
Patient 1 postsurgery (mm 3 ) | 39,130 | 28,413 | 10,718 |
Increase (mm 3 ) | 13,104 | 7,653 | 5,452 |
Increase (%) | 50.35 | 36.86 | 103.53 |
Patient 2 presurgery (mm 3 ) | 47,975 | 30,536 | 17,439 |
Patient 2 postsurgery (mm 3 ) | 68,775 | 29,990 | 38,785 |
Increase (mm 3 ) | 20,800 | (546) | 21,346 |
Increase (%) | 43.36 | –1.79 | 122.40 |
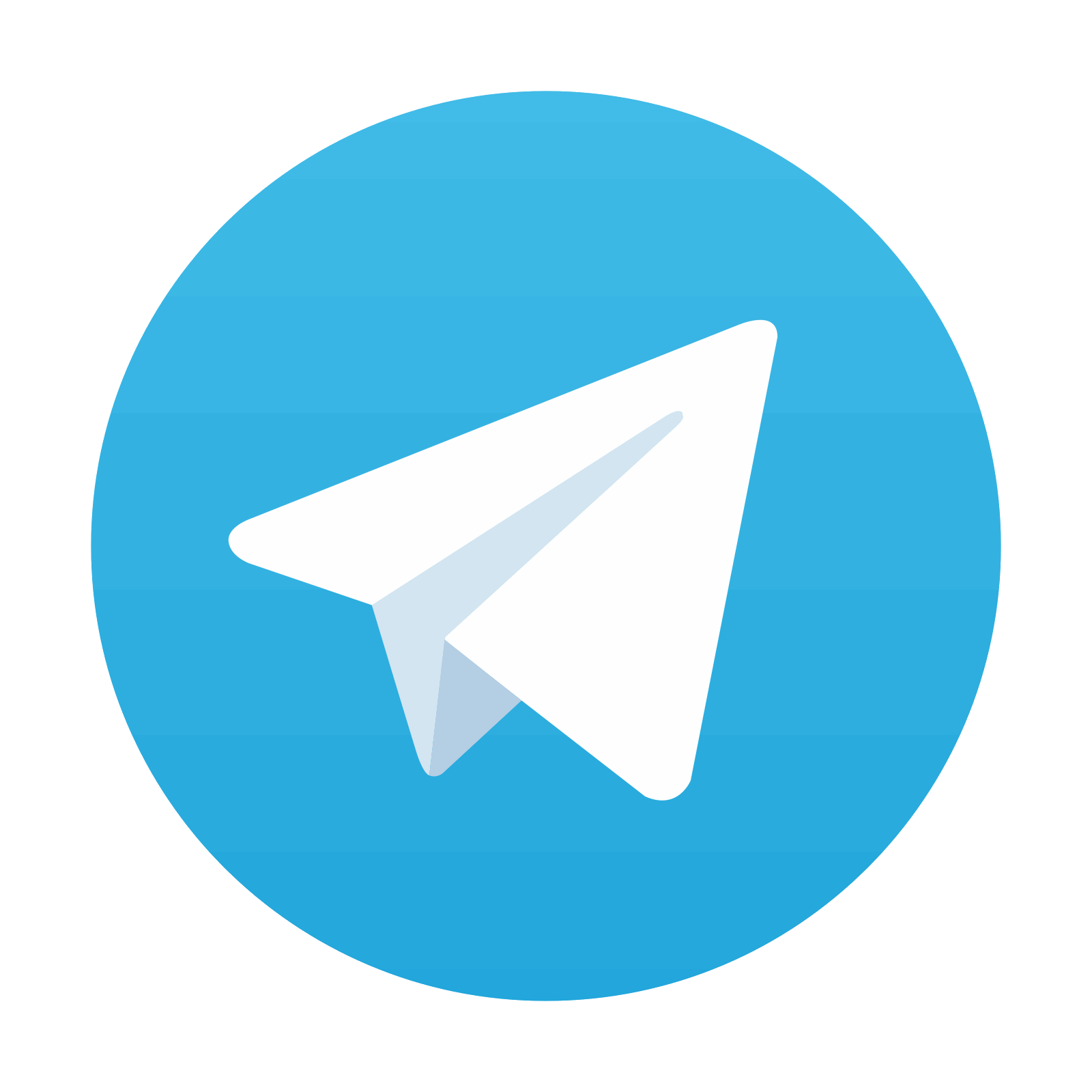
Stay updated, free dental videos. Join our Telegram channel

VIDEdental - Online dental courses
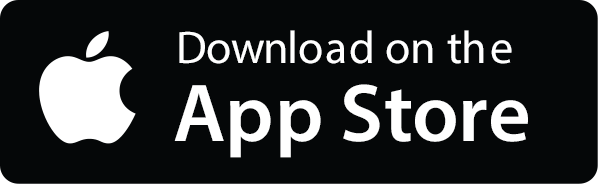
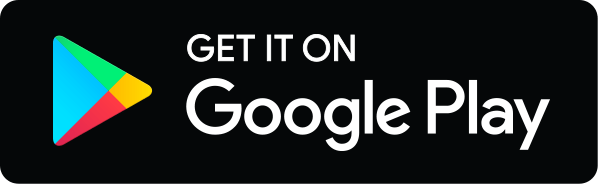
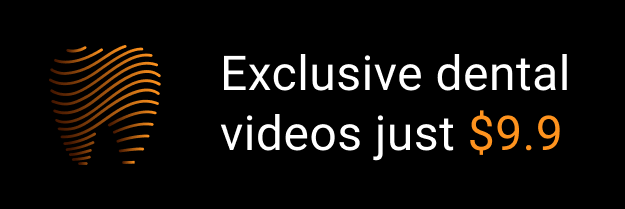