Introduction
C-expanders are tissue- and bone-borne maxillary expanders that are anchored by 6 orthodontic miniscrews, 3 on each side of the palate. The purpose of the study was to investigate the effect of C-expanders on the circummaxillary sutures and bucco-palatal axis of teeth in 3-dimensional finite element analyses when anchor screw vectors are different.
Methods
Five expansion models were studied on the basis of the vertical positions of anchor screws on the palate. Anchor screws for models A, B, and C were placed symmetrically at 4 mm, 7 mm, and 15 mm below the cementoenamel junction (CEJ), respectively. Anchor screws for models D and E were placed asymmetrically at 4 mm and 15 mm below CEJ and 7 mm and 15 mm below CEJ, respectively. Stress, displacement, and angular changes of the bone and teeth were measured in elastoplastic behavior models using a static-nonlinear simulation in an implicit method.
Results
Symmetrical and asymmetrical anchor screw placement with different vertical vectors were compared using finite element analyses on 5 models.
Conclusions
Using different vectors of anchor screws for C-expanders does change the pattern of palatal expansion (null hypothesis was rejected). The current investigation presents a promising future of controlled asymmetric skeletal maxillary expansion when asymmetric maxillary architecture needs to be corrected for successful orthodontic outcomes without involving orthognathic surgeries.
Graphical abstract

Highlights
- •
Stress was concentrated around the anchor screws in all 5 models.
- •
Maxillary molars were displaced posteriorly, buccally, and superiorly in all models.
- •
Different vectors of anchor screws do not change the pattern of palatal expansion.
Depending on the degree of dental compensation in a given maxillary transverse discrepancy, the intraoral manifestation of the posterior crossbite can vary significantly, and the orthodontic treatment strategy to achieve a stable and healthy occlusion in each situation should differ accordingly. Rapid palatal expansion is used to address maxillary deficiencies in the transverse dimension such as posterior crossbite and maxillary constriction, as well as to increase dental arch perimeter in patients with tooth-size and arch-length discrepancies. It becomes even more complicated when the patient presents with a posterior crossbite that is caused by a combination of varying degrees of skeletal transverse discrepancy and bucco-palatal dental compensation between the right and left sides of the maxillary arch.
Historically, tooth-borne palatal expanders have been commonly used to treat posterior crossbites. , Among conventional tooth-borne palatal expanders, a modified W-arch, quad helix, transpalatal lingual arch, or acrylic bonded appliance with an occlusal locking mechanism have been used for adult patients to correct unilateral posterior crossbite primarily by dental compensation. Some of the reported side effects of tooth-borne palatal expanders include buccal tipping of posterior teeth, loss of buccal bone thickness and buccal crestal bone level, buccal root resorption, and postretention relapse. To avoid these side effects, palatal expansion can be accompanied by a LeFort I level partial maxillary osteotomy under general anesthesia, as a form of surgically assisted rapid palatal expansion (SARPE). However, the added invasive surgery can be difficult for patients to accept because of the time off from work and managing the escalated cost of treatment. In addition, the primary effect of SARPE on the maxilla is shown to be a lateral rotational movement rather than a true horizontal skeletal translation. This effect is due to the fact that conventional tooth-borne palatal expanders are anchored to teeth which inherently prescribe the force of expansion to be applied at the occlusal level, far from the center of rotation at the midpalatal suture, which is a surgically created fulcrum in SARPE.
In recent years, the use of orthodontic skeletal anchorage devices such as orthodontic miniscrews and mini-plates has expanded to maxillary expander designs that can generate skeletal translational expansion while minimizing unwanted effects on the teeth. Examples include a miniscrew-assisted rapid palatal expander (MARPE) as a tooth-borne and bone-borne expander, , a Dresden distractor as a pure bone-borne expander, and a Biocreative Bone-borne expander (C-expander) as a tissue- and bone-borne expander. , Successful palatal expansion of these expanders has been consistently reported with emphasis on the increase of the maxillary arch width without remarkable side effects on teeth and periodontium. Yoon et al have reported the effects of variable locations of anchor screws of a MARPE in a finite element (FE) analysis. However, the study analyzed the effects of variable anchor placement vectors in the symmetrical anteroposterior direction only. The effects of different vertical vectors of anchor screws for bone-borne palatal expanders have not yet been reported, and thus, remain vastly unknown to orthodontic professionals who need to make a strategic decision on the final locations of anchor screws.
The purpose of this study was to investigate the effects of C-expanders in FE analysis when different placement vectors of anchor screws are selected in the vertical dimension, both symmetrically and asymmetrically. The null hypothesis is that different anchor placement vectors of a C-expander in the vertical dimension do not change the pattern of palatal expansion.
Material and methods
The geometric information of a human skull was imported into Visual-mesh software (version 7.0; ESI Group, Paris, France) to generate a tetrahedral FE mesh. The model was divided into the areas of cortical and trabecular bone of the maxilla, teeth, periodontal ligaments, and other bones ( Fig 1 ). The information from the C-expander was imported the same way and converted to a FE model. The size of a tetrahedral mesh was set at 2.0 mm, except the mesh area around an anchor screw, which was set at 0.2 mm. Alveolar bones, teeth, periodontal ligaments were considered to be homogenous and isotropic. The number of elements is shown in Table I , and the material properties of each component are shown in Table II . Bilinear elastoplastic modeling was used to build a virtual human skull. The midpalatal suture and the nasofrontal suture were programmed to respond in an elastoplastic behavior. The bilinear model provides a more realistic representation of the difference in suture stiffness before and after its breakup or separation. The initial Young’s modulus was set at 1 MPa, threshold stress (transition) was at 0.1 MPa, and the final elastic modulus was set at 0.01 MPa as suggested by Serpe et al The foramen magnum was set as immobile to serve as the origin point. The coordinates were defined using the sagittal plane as the x-axis, the transverse plane as the y-axis, and the vertical plane as the z-axis.

Component | No. of meshes |
---|---|
Maxilla, cortical | 169,927 |
Maxilla, trabecular | 234,628 |
Tooth | 35,175 |
Periodontal ligament | 13,580 |
Expansion screw and devices | 490,656 |
Other bones | 296,785 |
Miniscrew | 52,575 |
Total | 1,250,751 |
Component | Young’s modulus (GPa) | Poisson’s ratio |
---|---|---|
Cortical bone | 13.7 | 0.3 |
Trabecular bone | 1.37 | 0.3 |
Dentin | 20.7 | 0.3 |
Periodontal ligament | 1.68 × 10 −4 | 0.49 |
Titanium | 110 | 0.35 |
C-expander was selected as the expander of choice because of its versatility of allowing various vertical positions of anchor screw placement in the actual clinical situation. The components of the C-expander included 6 anchor screws with 3 on each side of the palate, an expansion screw with 4 connector arms, and 2 resin pads that harbor 3 anchor screws and 2 connector arms in each pad. , The anchor screw has a diameter of 1.6 mm and a length of 8.0 mm (BIo-Action screws; Jin Biomed, Bucheon, Korea). Three anchor screws were placed on each side of the palate as the first step of the C-expander installation process. These screws were placed at the spaces between the canine and the first premolar, between the first and second premolars, and between the second premolar and first molar. An alginate impression of the maxillary arch was then taken to custom fabricate the C-expander on the stone model. The expansion screw (Forestadent, Pforzheim, Germany) was positioned in the middle of the palate in a manner that was slightly away from the palatal mucosa with terminal connector arms fully embedded in a resin pad on each side of the palate. Initially, the resin pad had a hole that was large enough to have clearance from the already-placed anchor screws (Forestacryl; Forestadent). After the expansion screw and the resin pads were positioned inside the patient’s mouth, the large hole inside the resin pad was filled and cured using hard acrylic resins to integrate the anchor screws and the resin pads completely. The thickness of the resin pad was approximately 1 mm.
Five expansion models were studied on the basis of the vertical positions of anchor screws on the palate. Anchor screws for models A, B, and C were placed symmetrically at 4 mm, 7 mm, and 15 mm below the CEJ, respectively. Anchor screws for models D and E were placed asymmetrically at 4 mm and 15 mm below CEJ and 7 mm and 15 mm below CEJ, respectively ( Fig 2 ). To avoid unwanted contact to the root of the teeth, anchor screws were placed at an angulation of 60° to the palatal surface when they were at 4 mm below CEJ, 75° at 7 mm below CEJ, and 90° at 15 mm below CEJ. The expansion screw was activated by 0.25 mm in the y-axis in all 5 models. The values of von Mises stress, which show the possibility of yield and deformation of teeth and bones, were obtained. The static-nonlinear simulation was used with the implicit method using a Virtual Performance Solution (version 2008; ESI Group, Paris, France). All simulations were performed on a computer with Intel Xeon CPU E5-2680 v4 at 2.40 GHz × 28 core (Intel Corp, Santa Clara, Calif) and the 128 gigabytes random access memory.
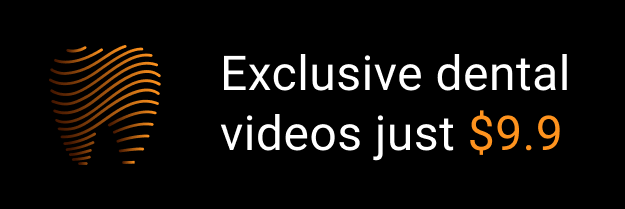