Facial skeletal augmentation is one of many techniques used to enhance facial aesthetics. It is especially useful in the malar, mandibular angle, and genial areas. For many years, correction of facial contour deformities posed challenges for reconstructive surgeons. Two-dimensional radiographic and photographic imaging modalities provided limited diagnostic and treatment planning information. An arduous procedure could be undertaken to take a facial impression and create a stone facial model of the facial soft tissues. This three-dimensional (3D) model simulated the patient’s face but provided no information on the underlying bony contours. The surgeon primarily used their artistic ability to diagnose and treat facial contour deformities. Treatment was limited to the use of stock implants that were placed as is or altered at the time of surgery. With the more widespread use of computed tomography (CT) in the 1980s, 3D representations of the patient’s facial skeletal anatomy became available. Computer technology has advanced to allow an accurate duplication of a patient’s facial skeletal and soft tissue anatomy. According to Winder and Bibb, medical rapid prototyping is defined as the manufacture of dimensionally accurate physical models of human anatomy derived from medical image data . This technology was originally described by Mankowich and colleagues in 1990 . With the use of this technology, the ability to manufacture or fabricate custom craniofacial implants has evolved.
Proprietary software programs allow computer-assisted design (CAD) and prototyping through computer-assisted manufacturing (CAM). These technologies allow the fabrication of custom implants to replace or augment the facial skeleton and enhance a surgeon’s ability to treat facial contour abnormalities .
The creation of custom implants with CAD/CAM technology provides the surgeon with several advantages over the use of stock implants. The 3D information and virtual software provide for better patient evaluation and treatment planning, especially in cases of facial asymmetry. If a surgeon wants to correct unilateral deformities, mirror imaging software can be used to fabricate an implant that duplicates the facial skeleton of the opposite side. Customized implants have a more precise fit because the undersurface of the implant is manufactured to fit precisely to the patient’s skeletal anatomy. These implants adapt to sharp curvatures and bony abnormalities that may be present . This is particularly advantageous in posttraumatic facial contour abnormalities, in which the custom implants fit into irregular defects and the edges of the implants blend into the facial anatomy and are not visible or palpable. Custom implants require little if any surgical time to hand carve. It is not necessary to alter the bony anatomy as is often the case with stock implants, reducing surgical and anesthesia time. Disadvantages of 3D modeling for custom implants include the need for CT scans, increased preoperative time for planning, and increased expense to the patient for the CAD/CAM process.
The purpose of facial augmentation is cosmetic enhancement, not functional improvement. It is important to consider the patient’s concerns and expected outcome when planning treatment. The clinical examination allows the best evaluation of contour deformities. 3D imaging allows the best means of quantifying the deformity and planning treatment. The area of interest is evaluated for symmetry, height, width, depth, and its relationship to the entire face. In most cases, aesthetic criteria and the surgeon’s experience blend to provide the optimal treatment of an individual patient.
Surgeons have alternatives for facial implants. They may use stock implants, customized stock implants, or custom fabricated implants, all made from a variety of materials. Both stock implants and custom implants have been found to have a low complication rate and good long-term success . The surgeon must evaluate the pros and cons of each technique in the context of each individual patient and their presenting problems to determine the most appropriate treatment.
Technology
The initial step in fabricating custom implants begins with a 3D image. Historically, medical CT scanners have been used. During the past 10 years, cone beam CT (CBCT) has become available as an office-based alternative. There are important differences between the 2 technologies. Currently, 64-slice and 128-slice medical CT scanners are commonly available. They can scan a patient’s maxillofacial region in little more than a second, thus reducing movement artifact . CT scans are more accurate and of higher quality than CBCT scans . CBCT scans have less metal artifact (scatter) and expose the patient to less than 10% of the radiation received from a CT scan . Manufacturers have software that can improve the 3D reconstructions from CBCT scans, making them acceptable for custom facial implants (Andrew Christiansen, Medical Modeling Inc, Golden, CO, USA, personal communication, 2011). Because of the reduced radiation and convenience, the office-based CBCT scanner is a good choice for the surgeon.
Whether using CT or CBCT, it is important to follow the specific protocol required by the manufacturer that is providing the technical support and implant fabrication. The area of interest and any surrounding area necessary to aid in treatment should be scanned. For instance, to augment the mandibular angle or malar area it is usually necessary to scan the entire face and orbits. Among other things, this strategy enables the surgeon and software designer to create a facial midline and to compare matched anatomic parts. Once the scan is completed, the imaging data or DICOM (Digital Imaging and Communications in Medicine) information is transferred to the commercial manufacturer, where the design process begins.
The commercial manufacturer uses proprietary software to reformat the DICOM images into 3D skeletal and soft tissue images. The images allow the surgeon to quantify and further evaluate the patient’s contour deformity. The software can be used to create an anatomic resin stereolithographic model that illustrates the position of vital structures such as the mandibular canal and any teeth that are present ( Figs. 1 and 8 A ).

The surgeon has a few alternatives, varying from a handmade approach to the complete virtual creation of the custom implants. Both techniques create implants that adapt well to the patient’s bony anatomy (see Fig. 8 C).
Techniques
Handmade Approach
A 3D resin stereolithographic model is obtained from the manufacturer. The model allows direct measurements of the deformed area and its relationship to other areas of the face. The surgeon uses a modeling material to custom sculpt the shape of the implant. The soft material can be carved, trimmed, or shaped as desired. A needle can act as a depth gauge and be passed through the material to check its thickness. When the surgeon is satisfied with the shape, the modeling material is allowed to dry and harden. It is then sent to the manufacturer, where it is duplicated in the implant material of choice (see Fig. 1 ).
Virtual Technique
This technique is completely digital, thus alleviating the need for a resin stereolithographic model. The surgeon works with a software designer at the manufacturing company to create the optimal shape and size of the implant. The surgeon can start with a block of digital modeling compound or with the typical shape of a stock implant, modifying it as needed ( Fig. 2 ). One benefit of computer planning is the ability for mirroring. Mirroring allows the comparison of the affected side with that of its normal counterpart. The surgeon can accurately determine the size and shape of the implant needed to match the shape of the normal counterpart ( Fig. 3 ). An amount of asymmetry is always present in human paired and unpaired parts. Regarding the facial skeleton, the more asymmetry that is present the harder it is to determine a midsagittal plane and the less reliable mirroring is .
Techniques
Handmade Approach
A 3D resin stereolithographic model is obtained from the manufacturer. The model allows direct measurements of the deformed area and its relationship to other areas of the face. The surgeon uses a modeling material to custom sculpt the shape of the implant. The soft material can be carved, trimmed, or shaped as desired. A needle can act as a depth gauge and be passed through the material to check its thickness. When the surgeon is satisfied with the shape, the modeling material is allowed to dry and harden. It is then sent to the manufacturer, where it is duplicated in the implant material of choice (see Fig. 1 ).
Virtual Technique
This technique is completely digital, thus alleviating the need for a resin stereolithographic model. The surgeon works with a software designer at the manufacturing company to create the optimal shape and size of the implant. The surgeon can start with a block of digital modeling compound or with the typical shape of a stock implant, modifying it as needed ( Fig. 2 ). One benefit of computer planning is the ability for mirroring. Mirroring allows the comparison of the affected side with that of its normal counterpart. The surgeon can accurately determine the size and shape of the implant needed to match the shape of the normal counterpart ( Fig. 3 ). An amount of asymmetry is always present in human paired and unpaired parts. Regarding the facial skeleton, the more asymmetry that is present the harder it is to determine a midsagittal plane and the less reliable mirroring is .
Implant materials
Alloplastic implants offer many advantages over autogenous grafting, including predictable long-term results, reduced patient morbidity, and reduced surgical time. Over the years many different alloplastic materials have been used for maxillofacial augmentation. Although various biomaterials may be used for augmentation, only a few can be used for custom implant fabrication with the CAD/CAM process.
The ideal implant should be biocompatible and resistant to infection. It should be easy to customize, allow for easy removal, and be resistant to migration . Each material offers advantages and disadvantages, but the decision on the material selection is usually based on a surgeon’s experience and preference. The following implant materials are currently available in the United States for use as a custom implant.
Hard Tissue Replacement (HTR)
Hard tissue replacement (HTR) (Biomet Microfixation, Jacksonville, FL, USA) is a nonresorbable, porous, polymeric composite consisting of a polymethylmethacrylate substrate sintered with a polyhydroxyethylmethacrylate and calcium hydroxide coating. The external surface aids in imparting hydrophilic properties with extensive porosity. The interbead porosity ranges from 150 to 350 μm with a 200 μm intrabead pore size. Despite the porous nature of the material, it has significant compressive strength (5000 psi in moldable form) . As a result, it is relatively stiff and is the most rigid of the materials discussed in this article. The easiest way to alter the material is with trimming burs. One significant benefit of this material is that there is no bony erosion over time around the implant. Karras and Wolford showed no bony erosion in HTR chin implants over an average length of follow-up ranging from 12 to 44 months .
Medpor
Medpor (Stryker Corporation, Newman, GA, USA) is a solid porous polyethylene implant with a pore size ranging from 125 μm to 250 μm, which allows for tissue ingrowth . It produces a minimal foreign body reaction, resulting in a thin fibrous capsule. The material is available in stock implants of various shapes and sizes for facial augmentation. Because of the tissue ingrowth and limited potential for movement after tissue healing, some surgeons place these implants without screw fixation. However, Yaremchuk comments that screw fixation is critical for the successful use of these implants. In addition, he reports that by fixating these implants, potential movement that may lead to hematomas or seromas is minimized .
Silicone
Silicone facial implants were first placed in 1953. Because of its molecular makeup, silicone is highly resistant to degradation. It is highly biocompatible, with minimal to no clinical toxicity or allergic reactivity. Most commonly, this material is used in a vulcanized form that can be trimmed with the ease of a scalpel blade. These implants retain their strength and flexibility throughout a wide range of temperatures, allowing for easy sterilization. Because of the chemical inertness of the material, a fibrous capsule forms around the implant, with no tissue ingrowth . The implant and its capsule are not attached to the bone, and movement can be detected when palpated. This property allows for easier removal of the implant if necessary. One frequently reported disadvantage of this material is that these implants cause variable amounts of bone resorption, especially in areas of muscle function such as the chin area. This characteristic results in implant settling, making long-term stability and aesthetic outcomes less predictable .
PEEK Optima-LT (polyetheretherketone)
PEEK Optima-LT (Synthes, West Chester, PA, USA) has been engineered for strength, stability, and biocompatibility. Its stiffness and strength closely resembles those of cortical bone . Standard plates and screws may be used to stabilize these implants. However, according to the manufacturer, the screw holes must be predrilled away from the surgical site. This material is being used more frequently for craniofacial applications .
All of the above materials are radiolucent. Many surgeons soak the porous materials in an antibiotic solution before placing them. It has been shown that the most effective way of infiltrating antibiotics into porous materials is to place them in a large syringe with an antibiotic solution. Negative pressure is created in the syringe by a repeated pumping action while tapping the syringe to knock off the bubbles that form. The result is to replace the air in the pores on the surface with the antibiotic solution. There are no available studies to show the effectiveness of this technique in reducing the perioperative risk of infection .
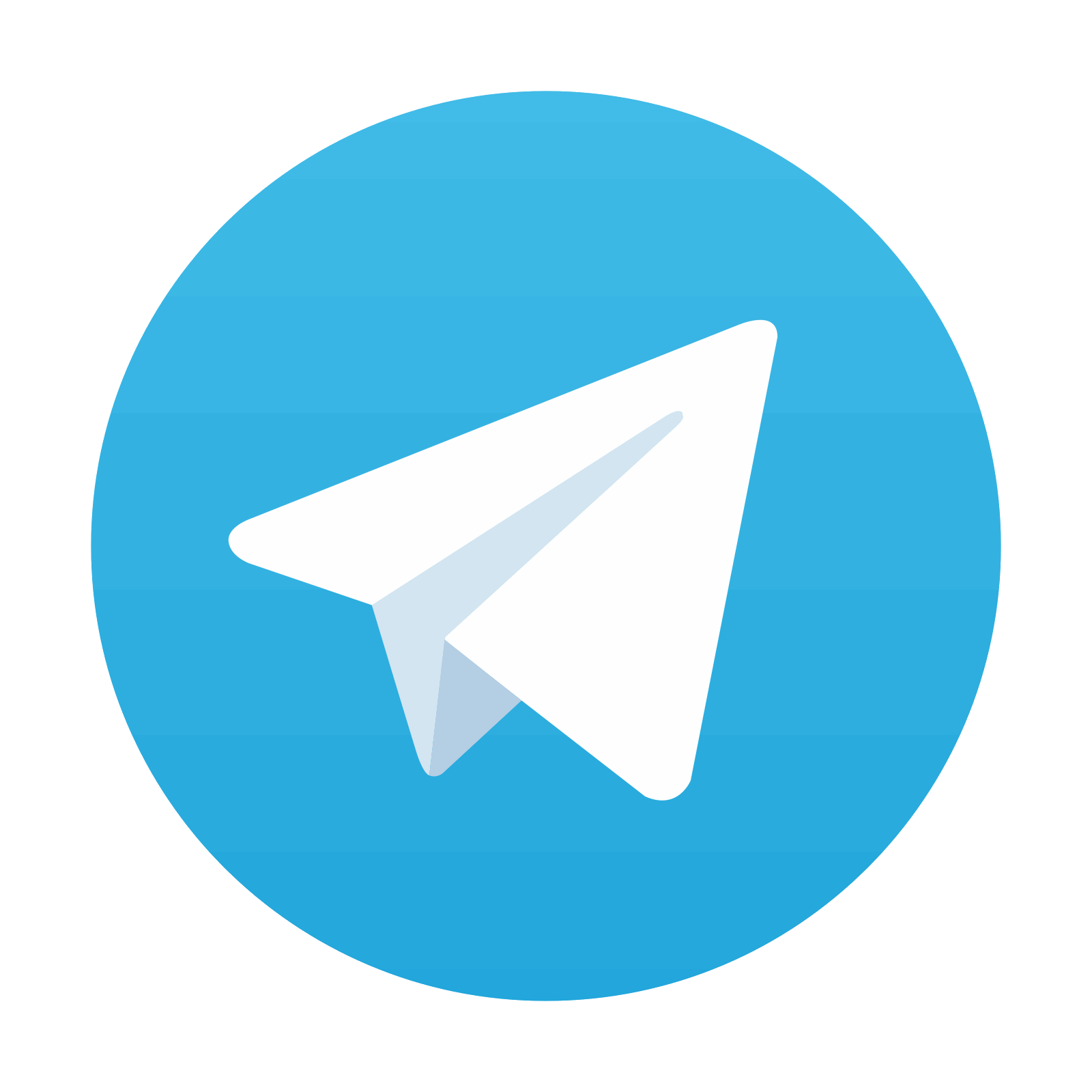
Stay updated, free dental videos. Join our Telegram channel

VIDEdental - Online dental courses
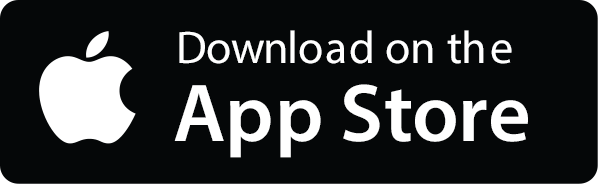
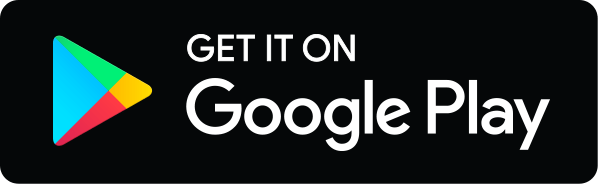