Primary base of occlusal rim in triad material for the mandible

Secondary wax rim which fits over the primary part

Primary base of occlusal rim in triad material for maxilla

Wax rims created to average dimensions

Primary parts of rims screw retained intraorally

Midline marked on maxillary rim

Level of mandibular occlusal rim set

Vertical dimension and centric relation registered
13.1 High-Performance Polymer: PEEK
The high-performance polymer, polyether etherketone (PEEK) , was first introduced as a load-bearing biomaterial for spinal fusion surgery over 15 years ago (Invibio Biomaterials Solutions). Since then, medical grade PEEK polymers have matured into an established biomaterial. Until recently its use in dentistry was limited to healing caps and temporary abutments. Perhaps the most interesting property of PEEK for use as a framework material is its Young’s elastic modulus (4 GPa), which allows the PEEK substructure to more closely match the biomechanical characteristics of the jaw’s natural bone (2–12 GPa). PEEK is quite favourable in that it is strong and resistant to repetitive cyclical loading cycles, yet is slightly elastic, lightweight and able to dissipate stress forces placed on it. It is these ‘bone-like’ properties that make PEEK a more biomechanically engineered substructure material.
The Young’s elastic modulus of PEEK (4 GPa) is similar to the acrylics (2 GPa) and is a lot lower than titanium (100 GPa), but still retains sufficient stiffness for rigidity of structure. However, unlike acrylic, PEEK also has sufficient strength (120 MPa flexural strength versus 40 MPa for acrylic) and excellent flexural fatigue resistance to cyclical loads that make it fit for the purpose of long-term restorations. PEEK’s resistance to failure from flexural fatigue may be of interest to potentially address some of the technical complications associated with cantilever design and lab-based tests have been published that show resistance to failure with distal cantilevers of up to 19 mm length [1].
Shock absorption tests have demonstrated a strong correlation between the damping behaviour of implant-supported crowns and their material composition [2]. Studies have shown that the shock-absorbing capacity of polymer materials was higher than that of ceramics and metals. In recent studies that compared titanium, cobalt chrome, zirconia, lithium disilicate, PMMA (acrylic) and PEEK, it was the latter material that demonstrated the biggest differential between forces applied at an occlusal crown surface and forces transferred through to the implant fixture side [3]. This property of a framework material may be of interest for aspects of patient comfort or for particular cases with parafunctional considerations. The weight of a PEEK framework (e.g. 5 g) is also significantly less than one replicated in titanium (17 g).
PEEK as a permanent framework material has become an option in recent times through the availability of CAD/CAM forms. However, clinical data is typically limited at present to case studies [4–6]. Prospective studies are becoming available but there is currently no long-term follow-up [7].
13.2 Laboratory Fabrication Process
PEEK frameworks can be designed according to the following guidelines for full-arch implant-supported prosthetics [8]. In general, when designing any structural polymer part, there should be the avoidance of any notches or sharp angles into the framework. These notches, scoops and grooves can create potential areas of weakness for polymers and propagate cracks. If unavoidable then the framework thickness behind the area should be at least 2 mm and the angle must be greater than 45°.
Anatomical implant substructures—If the prosthetic is screw retained then a bonded metal connector can be used or in instances where the PEEK material is direct to implant then considerations should be made for the interface of PEEK with the particular implant system and screw design. For example, screw head designs that are conical and of smaller diameter will more readily cut into the polymer if in direct contact, while wider flat-headed screws are more compatible. Abutment wall minimum thicknesses should be 1 mm. Current guidance for minimum widths of the framework anterior and posterior base is 8 and 9 mm, respectively. Minimum framework height should be 5 mm and at sites of the implants the material should be at least 1.5 mm on the buccal side and 2 mm on the lingual side.
Implant bars—The bar height should have a minimum of 4 mm. Posterior wall thickness should be a minimum of 6 mm and anterior wall thickness a minimum of 5 mm. Abutment wall thickness minimum should be 1 mm.
- 1.
The correct selection of compatible bonding agents, adhesives and cements: The user should refer to the material supplier’s guidelines since PEEK is not compatible with all cements and veneering systems that are typically used for traditional materials.
- 2.
The correct surface preparation of the framework prior to bonding is important to roughen the surface and create additional surface area to provide a mechanical key. This can be achieved using grit blasting, for example.
A variety of traditional approaches can be used to create the aesthetics on a PEEK framework, for example, veneering with composite materials, use of injection-moulded acrylic and acrylic teeth to create a hybrid wrap-around, or hybrid designs with the use of individual crowns (ceramic, zirconia or lithium disilicate).

Failing dentition

Custom tray impressions following osseointegration

Digitised master model with laboratory analogues

Digitised model with proposed framework design

Digitised model with proposed framework design and veneers

CAD/CAM milling path calculation of the nested framework

Lateral view of the final veneered PEEK framework

Occlusal view of PEEK prosthetics after placement

Final PEEK restorations showing aesthetics that can be developed

Intraoral view of restoration in Fig. 13.17
13.2.1 Acrylic Resin Titanium Hybrid
The original frameworks made by the Branemark group had been fabricated from type III gold. Because of the high cost of this material, alloys of silver palladium and gold palladium had been used in recent years. Passivity in these types of frameworks had been notoriously difficult to achieve. Inaccuracies were the result of multiple variables, which included machining tolerances of components, distortion in the impression material, setting expansion of the die stone, expansion and contraction of alloy and wax and distortion of the framework during heat treatment [12, 13].
Common solutions to provide a passive framework had been sectioning and soldering or fabricating the framework in multiple pieces which were then soldered on a master cast. Another approach to achieve a passive screw-retained framework had been use of the adhesive-corrected implant frameworks where individual cylinders were cemented within the framework after it had been cast (KAL technique). This approach had merit in that it eliminated many of the current prosthetic and laboratory inaccuracies associated with traditional techniques [14–17].
With CAD/CAM technology a lot of the variables have been eliminated and frameworks can be produced with high precision providing that the operator has taken care in producing an accurate impression and the laboratory technician has exercised care in pouring it.

Comparison of traditional gold and titanium frameworks

Wrap-around framework
- 1.
Bulk for strength
- 2.
Adequate access for oral hygiene
- 3.
Minimal display of metal
- 4.
Retention for acrylic resin
- 5.
Adequate space for acrylic resin
- 6.
Adequate thickness of framework in the cantilever area
- 7.
Attention to cross section
- 8.
Minimising the cantilever
There are essentially two types of titanium framework that exist.
Wrap-around—This is a minimalist framework where acrylic resin is wrapped around the titanium framework. The tissue surface is acrylic resin and can be readily relined. Little is known of its biomechanical longevity. In the authors’ experience common problems include fracture of the bar. Whether this is due to inadequate dimensions or excessive cantilever is unknown (Fig. 13.20).

L-shaped framework
- 1.
Fracture of the bar
- 2.
Fracture of the restorative material
- 3.
Wear of the teeth
Length of the cantilever is the most common cause of restoration failure. If looking at deflection of the restoration it is proportional to the length of the cantilever and thickness of the cross section. Small increases in length have a dramatic effect of deflection. Most articles recommend a cantilever length which is two times the anteroposterior spread. It is the authors’ opinion that the cantilever should be minimised as much as possible and should only be extended as minimally as possible [19].

Milled metal occlusal surfaces to maintain vertical dimension
13.3 Laboratory Fabrication Process
Attention to detail starts with pouring the impression using a low-expansion die stone. Appropriate powder liquid ratios and vacuum mixing for the time indicated by the manufacturer must be adhered to. A verification index must be provided to the clinician to verify accuracy of the master cast. This index is made from impression plaster. A brittle material that is not forgiving is used. The verification index is tried in with one screw. If the plaster index breaks the master cast is deemed inaccurate and a new impression must be made. The use of an acrylic resin material for verification of the master cast may give the clinician a false-positive result and is not recommended. Jaw relation records utilising a two-part rigid occlusal rim must be accomplished after which a wax trial set-up must be evaluated intra-orally.
The wax trial prosthesis must be verified for correct aesthetics, phonetics, occlusal plane, vertical dimension of occlusion and centric relation.
- 1.
Bulk for strength—I- or L-shaped bars are preferred for maximum rigidity. If a conventional wrap-around bar is being used a minimum cross section of 4 mm by 4 mm must be used. The cylinders around the screw access holes should measure at least 0.65 mm in wall thickness.
- 2.
Adequate access for oral hygiene—The undersurface should be designed with a convex surface which compresses the tissue. Immediately adjacent to the implants/abutments there should be sufficient space to allow the patient to utilise an interproximal brush.
- 3.
Minimal display of metal—This should be designed into the framework and finish lines placed appropriately.
- 4.
Retention for acrylic resin—There should be sufficient characteristics on the framework to prevent separation of the resin from the metal framework.
- 5.
Adequate space for acrylic resin—There should be a sufficient thickness of acrylic resin for strength. 2–3 mm thickness is considered optimal.
- 6.
Cantilever—This is based on the anteroposterior spread of the implants and should be designed so that the cantilever is biomechanically favourable.
- 7.
Attention to cross section—The area distal to the most terminal implant is the area that is under the most load. Specific attention to cross section in this region must be given to limit the flexibility.
- 1.
A putty matrix (A) is made of the wax tooth try-in made on the articulator (Figs. 13.23, 13.24, 13.25 and 13.26).Fig. 13.23
Mandibular wax-up being prepared for indexing on articulator
Fig. 13.24Plaster base mounted on articulator with notches to receive putty index
Fig. 13.25Putty index to maxillary base (A)
Fig. 13.26Intaglio of putty index
- 2.
A putty matrix (B) is made of the wax tooth try-in to index the buccal and incisal surfaces (Fig. 13.27).Fig. 13.27
Buccal and incisal putty index (B)
- 3.
- 4.
The teeth are removed leaving the wax substructure intact (Fig. 13.31).Fig. 13.31
Teeth removed from wax-up
- 5.
The teeth are placed in matrix (B) and set back onto the model (Fig. 13.32).Fig. 13.32
Teeth put in matrix (B)
- 6.
The space that was previously occupied by the wax will be utilised for the titanium substructure. This space is evaluated to ensure that there is sufficient space for the bar, acrylic resin and tooth. If space is insufficient the teeth are adjusted to create space (Fig. 13.33a, b). 3 mm of acrylic thickness is required. The bar dimensions must be sufficient for rigidity.Fig. 13.33
(a) Matrix with teeth on cast to evaluate space on right side. (b) Matrix with teeth on cast to evaluate space on left side. (c) Teeth waxed into matrix (B) to secure them into place
- 7.
Wax is applied to the teeth in matrix B to secure them into place (Fig. 13.33c).
- 8.
- 9.
A light body impression material is injected into the holes to occupy the space held previously by the wax. This forms an intaglio index (Fig. 13.36).Fig. 13.36
Soft light body impression material injected into holes to capture intaglio of index and teeth
- 10.
The intaglio index is trimmed and cleaned. This index will be used for scanning to create the titanium substructure (Figs. 13.37, 13.38, 13.39 and 13.40).Fig. 13.37
Lingual index removed to show intaglio index
Fig. 13.38Intaglio index cleaned up and adjusted to ensure sufficient space between teeth and index
Fig. 13.39Intaglio index which will be used for scanning
Fig. 13.40Lateral view of intaglio index
- 11.
The master cast is also scanned so that the CAD software can recognise the implant platform.
- 12.
The titanium framework is designed using CAD software (Figs. 13.41, 13.42, 13.43, 13.44, 13.45 and 13.46).Fig. 13.41
Master cast scanned
Fig. 13.42Intaglio index scanned
Fig. 13.43Framework designed in reference to the intaglio index to ensure that framework is designed with tooth position in mind
Fig. 13.44Buccal view of framework design
Fig. 13.45Occlusal view of framework design
Fig. 13.46Definitive framework design
- 13.
- 14.
The framework is verified on the cast in reference to the putty matrix. The putty matrix (A) with and the titanium substructure on the master cast are replaced on the articulator for a final verification of the required space for acrylic resin. If space is required teeth may need to be adjusted as necessary (Fig. 13.50a, b).Fig. 13.50
(a) Framework with putty matrix (B) in place to check space for acrylic resin. (b) Framework with putty matric (A) to check space for acrylic resin
- 15.
The framework is degreased and cleaned with acetone.
- 16.
The framework is sandblasted with 50 microns aluminium oxide (Fig. 13.51).Fig. 13.51
Framework sandblasted with 50 microns aluminium oxide
- 17.
A metal primer is applied (Fig. 13.52).Fig. 13.52
Metal primer applied
- 18.
The first opaque layer is applied which has a higher degree of opacity to block the greyness of the titanium framework. Polymer powder is sprinkled onto the opaque layer to enhance the bonding surface (Figs. 13.53, 13.54, 13.55).
Stay updated, free dental videos. Join our Telegram channel
VIDEdental - Online dental courses
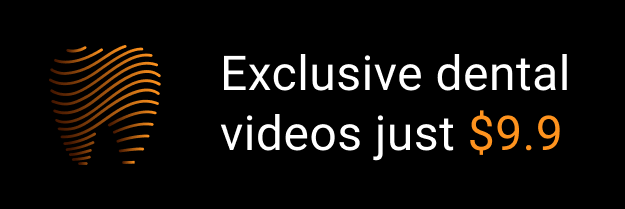