Abstract
Objectives
The characterization of the physical properties of dental resin composites is fraught with difficulties relating to significant intra and inter test parameter variabilities and is relatively time consuming and expensive. The main aim of this study was to evaluate whether optical fiber Bragg grating (FBG) sensing system may become a viable tool to study dental material characteristics. Of particular focus was the potential for the system to demonstrate a multi parameter all-in-one feature.
Methods
A miniature FBG was embedded in six different dental resin composites and employed as a sensor to evaluate linear polymerization shrinkage, thermal expansion and water sorption. Six commercially available dental composites with different filler types and volume are evaluated. The tests are repeated with three sets of samples. The curing characteristics and residual strain gradient exhibited by the cured dental composites were also observed and commented.
Results
Among the studied samples, SDR shows lowest polymerization shrinkage, while Beautifil FO3 shows the highest. The results also show clear distinction between particle filler type and fiber reinforcement based composites in their polymerization shrinkage properties. The agreement of the results with existing literatures show that FBG based system provides accurate results. Polymerization shrinkage rate of the samples are also obtained. Thermal expansion of the composites are measured using the FBG sensing method for the first time and is correlated with resin type, volume, filler type and glass transition temperature. The water sorption characteristics of the dental composite are also successfully measured using the FBG sensing method. The high level of repeatability and the low standard deviations shown in the results indicate good reliability with the use of FBG sensors.
Significance
This study demonstrates how optical fiber technology can provide simple and reliable methods of measuring the critical physical properties of dental composites. In addition due to the embedding and preservation of the sensor within the samples multiple parameters can be tested for with the same sample. These features are expected to greatly assist material science researchers in dentistry as well as other biomedical fields. Of some interest the phenomenon of stress relaxation of dental composite at higher temperature was observed.
1
Introduction
Dental composites have become the material most often employed in the restoration of teeth . This may be due to many factors including greater esthetic demands, toxicity concerns and trends toward the conservative management of dentitions. These materials are composed of varying amounts of radio-opaque particulate fillers dispersed in a dimethacrylate resin matrix. During polymerization the resin polymer centers grow by propagation reactions where weak (4 Å) van der Waals forces are replaced by stronger but significantly shorter (1.5 Å) covalent bonds and continues until the maximum degree of conversion of double carbon bonds (C C) to single carbon bonds (C C) has occurred.
Most of the observed shortcomings of dental composite such as weakness and marginal gap formation generally relate to the relatively weak resin matrix. Methods used to improve composite properties include: increasing the filler loading; using different size and shaped fillers (hybrid composites); altering the resin chemistry and modifying the reaction kinetics. A better understanding of the physical and chemical characteristics is essential for this. Some of the characteristic properties of dental composites that are of interest to clinicians include polymerization shrinkage stress and strain, degree and depth of curing, fracture strength and toughness, elastic modulus, surface hardness and wear, thermal expansion coefficient and water sorption characteristics. Typical volumetric shrinkage range is between 1 and 6% and is related to resin volume as well as the level of curing conditions such as irradiance rate and total power . If the shrinkage forces are greater than interfacial bond strength then marginal gap formations occur with associated sensitivity, staining, micro leakage and recurrent caries . Thermal expansion and their water sorption capabilities are two other characteristics that play a crucial role in determining the longevity of dental restorations. A difference in the thermal expansion coefficient between tooth and the restorative dental material can lead to stress within the material when there is a temperature change, and can lead to the formation of micro leakages . Water sorption of dental composites is mainly dependent on the type and volume loading of the resin matrix and results in a decrease in flexural properties due to the plasticizing effect of water . Therefore a more reliable and all-in-one characterization tool will less parameter variability would be highly beneficial for dental materials research.
In this paper a fiber optic sensor based method is introduced as a potential an all-in-one approach for dental composites characterization. Fiber-optic sensing technology is a powerful and potentially rich technology that is currently implemented in a wide variety of applications, where the optical fiber itself is acting as the sensor head . This technology can provide novel solutions to many challenging instrumentation requirements of the medical/dental industry and is recognized as one of the most promising approaches to sensing a wide variety of measurands . Because of their minimal weight, small size, high bandwidth, and immunity to electromagnetic interference, fiber-optic sensors have significant performance advantages over traditional electrical and mechanical sensors. Also, due to their miniature size (250 μm diameter) it is possible to embed the fiber optic sensor into the dental composites without impacting the inherent material properties.
Among the different type of fiber-optic sensors, fiber Bragg gratings (FBGs) , are widely used and considered as the most popular technology for implementing in monitoring systems and the preferred choice for many composite materials. Typically, an FBG consists of a single-mode optical fiber with a short grating (3–20 mm) written within the core of the fiber. If an FBG sensor is embedded into a host material, any change of the strain and temperature in the structure can be detected by measuring the Bragg reflected wavelength of light of the grating . Measuring the strain/temperature in the optical fiber would allow measurement of the strain/temperature in the structures if good adhesion between the optical fiber and composite is achieved.
Only a few medical studies using FBG sensors have been reported for dental material characterization. Initial work reported in measurement of shrinkage stress/strain and water absorption in dental composites shows promising results. Probably the first dental application of FBG sensors was the force and temperature monitoring of dental splints used by patients suffering from sleep apnea . The use of FBG sensors has been demonstrated in the monitoring of polymerization shrinkage and setting expansion of dental materials as well as in measuring strains at the mandible surface of a dental implant . Polymerization shrinkage and hygroscopic expansion of fiber reinforced composites using FBGs are also reported . With the feasibility of the approach is already demonstrated, the focus should be more on a comprehensive study to develop a multi parameter sensing tool, where one embedded sensor can provide all the necessary characteristic properties of the dental composite. In this paper a miniature FBG sensor is embedded in a range of commercially available dental composites to characterize linear polymerization shrinkage, thermal expansion and water sorption are evaluated using a single sensor embedded in one sample. Also to the authors knowledge this study is the first to assess thermal expansion with FBG sensors. Curing characteristics of the dental composites are also reported as well as the residual strain gradient, and stress relaxation at high temperatures.
2
Materials and methods
2.1
Dental material samples
Commercially available dental composites that represents different types such as – fiber reinforced, bulk fill, nano hybrid and flowable – are used in this study. Details of the samples including manufacturer, composition, mechanical properties, and reported polymerization shrinkage are shown in Table 1 . Details from both manufacturer data sheet as well original scientific publications were taken . The selections of the samples were based on a criterion to satisfy a broad range of materials with different type and varying percentage of resin and filler content. The sample set consists of materials that are nano hybrid to fiber reinforced with resin volume ranging from 29% (Nulite) to 55% (SDR).
Samples | Sample names | Manufacturer | Type | Resin type | Filler | Resin (%) | Fiber aspect ratio | Polym. shrinkage (%vol.) | Flexural modulus (GPa) | References | |
---|---|---|---|---|---|---|---|---|---|---|---|
vol | wt | ||||||||||
S1 | Beautifil II | Shofu, Japan | Nano Hybrid Universal | Bis-GMA/TEGDMA | Surface pre reacted glass ionomer nano particles (S-PRG) | 31.4 | 16.7 | 2.7 | 12.2 | [21–23] | |
S2 | Beautifil F03 Flow Plus | Shofu, Japan | Nano hybrid Flowable | Bis-GMA/TEGDMA | S-PRG | 53.7 | 4.4 | 7.6 | [23–25] | ||
S3 | SDR | Dentsply, USA | Bulk Fill | TEGDMA/EBADMA | Barium borosilicate glass | 55 | 32 | 3.5 4.5 |
6.9 | [23,25,26] | |
S4 | Ever X Posterior | GC, Japan | Fiber Reinforced Base | Bis-GMA/TEGDMA/PMMA | Glass fibers 1–2 mm long/barium silicate glass fillers | 37 | 23 | ∼100 | 2.9 | 12.3 | [26,27] |
S5 | Nulite F | BDT, Australia | Fiber Reinforced Hybrid | Bis-GMA | Glass fiber (36%)/filler (35%) | 29 | 17 | <20 | 1.56 | 16.0 | [28,29] |
S6 | Venus Diamond | Kulzer, Germany | Nano Hybrid | Tricyclodecan (TCD)-Urethane | Barium aluminum fluoride glass plus nano particles | 36 | 1.8 | 4.5 | [30,31] |
2.2
Fiber Bragg grating sensors
An elementary fiber Bragg grating comprises of a short section of single-mode optical fiber in which the core refractive index is modulated periodically using an intense optical interference pattern, typically at UV wavelengths. This periodic index modulated structure enables the light to be coupled from the forward propagating core mode into backward propagating core mode generating a reflection response. The basic operating principle of an FBG sensor is illustrated in Fig. 1 . The light reflected by periodic variations of the refractive index of the Bragg grating, having a central wavelength λ G , is given by ,
where n eff is the effective refractive index of the core and Λ is the periodicity of the refractive index modulation.

The basic principle of operation of any FBG based sensor system is to monitor the shift in the reflected wavelength due to changes in measurands such as the strain and temperature. The strain sensitivity of the Bragg wavelength arises from the change in period of the fiber coupled with a change in refractive index arising from the strain-optic effect, while the sensitivity of the Bragg wavelength to temperature arises from the change in period associated with the thermal expansion of the fiber, coupled with a change in the refractive index arising from the thermo-optic effect.
where ρ e is the photo elastic coefficient. For a silica core fiber the value of (1 − ρ e ) is usually 0.78. For the measurement of temperature change Δ T , the corresponding wavelength shift is given by,
where α is the coefficient of thermal expansion of the fiber material and ξ is the fiber thermo-optic coefficient. Typical strain and temperature sensitivity of standard silica FBGs at 1550 nm are 1.2 pm/μɛ and 10 pm/°C respectively. By measuring the wavelength shift, changes in temperature or strain and other measurands such as water sorption that induce such effects in the dental composites can be determined depending on which parameter the FBG sensor is being used to measure.
To measure the thermal expansion coefficient of the specimen material, for a known applied temperature and known temperature sensitivity for the FBG, CTE ( α s ) can be obtained from the expression below ;
The left-hand-side of Eq. (4) is the net wavelength shift caused by the thermal strain, where the first term Δ λ λ G α + T is the wavelength shift induced by thermal expansion and temperature, where the 2nd term Δ λ λ G T corresponds to the wavelength shift due to temperature change only. Thus by measuring the shift in the peak reflected wavelength of the FBG, polymerization shrinkage, thermal expansion and water absorption of the dental materials can be obtained.
The FBG used in the study are 3 mm long FBGs with peak reflected wavelength of 1550 nm with a reflectivity greater than 70%. The grating is fabricated on single mode silica fiber with polyimide buffer coating and has a diameter of circa 150 μm.
2
Materials and methods
2.1
Dental material samples
Commercially available dental composites that represents different types such as – fiber reinforced, bulk fill, nano hybrid and flowable – are used in this study. Details of the samples including manufacturer, composition, mechanical properties, and reported polymerization shrinkage are shown in Table 1 . Details from both manufacturer data sheet as well original scientific publications were taken . The selections of the samples were based on a criterion to satisfy a broad range of materials with different type and varying percentage of resin and filler content. The sample set consists of materials that are nano hybrid to fiber reinforced with resin volume ranging from 29% (Nulite) to 55% (SDR).
Samples | Sample names | Manufacturer | Type | Resin type | Filler | Resin (%) | Fiber aspect ratio | Polym. shrinkage (%vol.) | Flexural modulus (GPa) | References | |
---|---|---|---|---|---|---|---|---|---|---|---|
vol | wt | ||||||||||
S1 | Beautifil II | Shofu, Japan | Nano Hybrid Universal | Bis-GMA/TEGDMA | Surface pre reacted glass ionomer nano particles (S-PRG) | 31.4 | 16.7 | 2.7 | 12.2 | [21–23] | |
S2 | Beautifil F03 Flow Plus | Shofu, Japan | Nano hybrid Flowable | Bis-GMA/TEGDMA | S-PRG | 53.7 | 4.4 | 7.6 | [23–25] | ||
S3 | SDR | Dentsply, USA | Bulk Fill | TEGDMA/EBADMA | Barium borosilicate glass | 55 | 32 | 3.5 4.5 |
6.9 | [23,25,26] | |
S4 | Ever X Posterior | GC, Japan | Fiber Reinforced Base | Bis-GMA/TEGDMA/PMMA | Glass fibers 1–2 mm long/barium silicate glass fillers | 37 | 23 | ∼100 | 2.9 | 12.3 | [26,27] |
S5 | Nulite F | BDT, Australia | Fiber Reinforced Hybrid | Bis-GMA | Glass fiber (36%)/filler (35%) | 29 | 17 | <20 | 1.56 | 16.0 | [28,29] |
S6 | Venus Diamond | Kulzer, Germany | Nano Hybrid | Tricyclodecan (TCD)-Urethane | Barium aluminum fluoride glass plus nano particles | 36 | 1.8 | 4.5 | [30,31] |
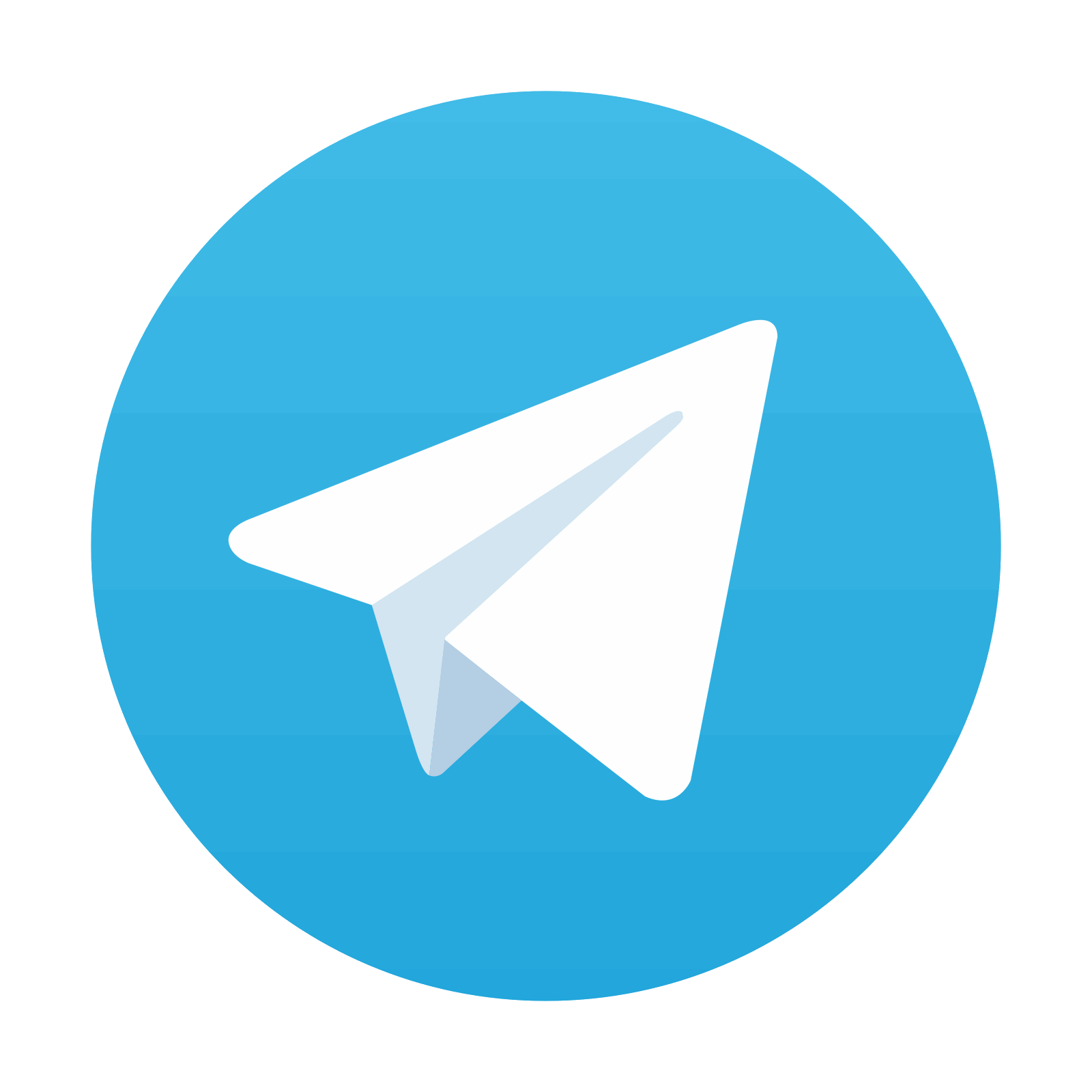
Stay updated, free dental videos. Join our Telegram channel

VIDEdental - Online dental courses
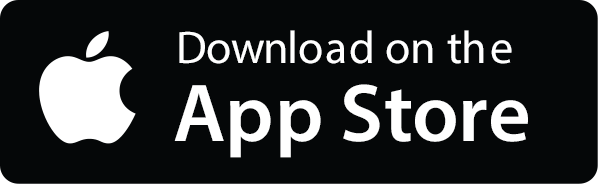
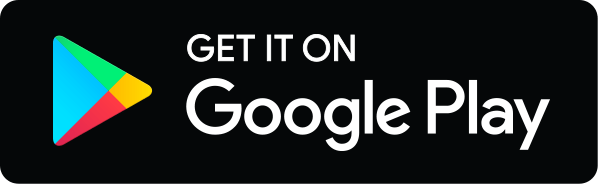
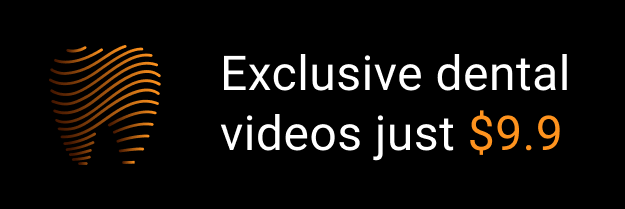