Highlights
- •
Nine monomers are evaluated as alternatives to HEMA in dental materials.
- •
Photopolymerization kinetics of these monomers are investigated by photo-DSC.
- •
Some of the tested monomers are promising candidates to replace HEMA in luting composites and self-etch adhesives.
Abstract
Objective
The objective of this work is to find potential alternative monomers to 2-hydroxyethyl methacrylate (HEMA) for dental materials (self-etch adhesives and luting composites).
Methods
Monomers 1–9 were tested as potential HEMA substitutes. Methacrylates 4 , 5 and 6 and ( N -methyl)acrylamides 7–9 were synthesized and characterized by 1 H NMR spectroscopy. The reactivity of each monomer was studied using photo-DSC. Mixtures of monomers 1–9 with the urethane dimethacrylate UDMA (1/1: wt/wt) were formulated and cured. The water sorption and solubility of these materials were determined according to ISO 4049. Luting composites based on monomers 1–9 or on HEMA were formulated. The flexural strength and modulus of elasticity were measured using a three-point bending setup, according to ISO 4049. Self-etch adhesives containing monomers 1–9 or HEMA were prepared and used to mediate a bond between the dental composite Tetric EvoCeram ® and both dentin and enamel. The shear bond strength (SBS) was measured using a Zwick universal testing machine.
Results
Polymerizable diols 3 and 4 as well as ( N -methyl)acrylamides 7–9 were found to be significantly more reactive than HEMA. Resins based on the hydrophilic monomers 3 , 7 and 8 exhibited a significantly higher water sorption than the corresponding HEMA-containing material. Luting composites containing monomers 2 , 3 , 6 and 7 showed similar or even improved mechanical properties compared to the reference material containing HEMA. Self-etch adhesives containing monomers 4 and 9 provided significantly higher dentin SBS than the reference material.
Significance
Some of the evaluated monomers are promising candidates for the development of HEMA-free dental materials.
1
Introduction
2-Hydroxyethyl methacrylate (HEMA) is the most frequently used hydrophilic monofunctional monomer that can be found in various dental materials such as dental adhesives, resin-modified glass ionomer cements (RMGICs) or luting composites . Depending on the etching process, dental adhesives can be classified into two main categories: total-etch (TEAs) and self-etch adhesives (SEAs) . When using TEAs, the dental hard tissues are typically etched using a phosphoric acid gel prior to the infiltration of an adhesive resin. On the other hand, SEAs are aqueous adhesive formulations containing an acidic monomer (e.g. 10-methacryloyloxydecyl dihydrogen phosphate (MDP)), which are able to simultaneously demineralize and infiltrate the dental tissues. SEAs are widely used in dentistry because of their short and reliable application procedure. The incorporation of HEMA in dental adhesives has many advantages . Regarding TEAs, it has been shown that the addition of HEMA to primers may lead to an improvement of dentin adhesion . Besides, an interesting property of HEMA is its ability to improve comonomer diffusion into the demineralized dentin matrix. One of the main problems associated with the use of one-step SEAs is the phase separation which can occur after drying . Indeed, SEAs contain both hydrophilic and hydrophobic monomers, which are often poorly miscible. A major advantage of HEMA relies on its ability to improve the miscibility between hydrophilic and hydrophobic monomers. As a consequence, the incorporation of this monomer to one-step SEAs can prevent the phase separation and therefore enhance the adhesive performance. Glass ionomer cements (GICs) are dental materials which set upon an acid–base reaction between a polyalkenoic acid and an ion-leachable fluoroaluminosilicate glass in the presence of water . GICs are able to adhere to the tooth substance and are well known for their anticariogenic effect due to the release of fluoride. RMGICs are hybrid materials that contain, in addition to the fluoroaluminosilicate glass and the polyalkenoic acid, HEMA. These cements are set upon both an acid–base reaction and a free-radical polymerization. The addition of HEMA results in an improvement of the mechanical properties and in a reduction of the moisture sensitivity . RMGICs are also easier to handle clinically than common GICs. HEMA has been selected for the development of RMGICs because it acts as a ‘co-solvent’. The presence of HEMA in luting composites allows an increase of the material hydrophilicity, resulting in a better compatibility between the composite and the hydrophilic primer.
Although the use of HEMA in dental materials can be advantageous, it also has several drawbacks. Indeed, HEMA is well known to exhibit a high allergenic potential . Uncured HEMA is able to penetrate through gloves and skin, causing contact dermatitis, a common disease among dentists . Moreover, uncured HEMA can diffuse through dental tubules, leading to pulp apoptotic cell death . A major problem associated with the use of HEMA in adhesives and cements is the significant increase of the water uptake . A high water sorption results in a decrease of the mechanical properties and can also facilitate the degradation of the dental polymer matrix. HEMA is also known to be low reactive and is not hydrolytically stable. There is therefore a need for alternative monomers. In this context, Münchow et al. and Zanchi et al. proposed various dimethacrylates to replace HEMA in adhesive systems . However, those crosslinking monomers exhibit a high molecular weight and are mostly highly viscous. Consequently, such compounds do not fulfill the requirements expected for HEMA alternative monomers. In order to improve the stability of the resulting dental materials, (meth)acrylamides such as N -(2-hydroxyethyl) acrylamide, N -methylolacrylamide (MEAA) or N -methylolmethacrylamide (MEMA) have been proposed to replace HEMA . Such monomers were shown to be more reactive in the free-radical polymerization than HEMA. Primers based on MEAA or MEMA provided better dentin adhesion than the corresponding primer containing HEMA.
In this context, the objective of this work is to evaluate monomers 1–9 as alternatives to HEMA in dental materials ( Fig. 1 ). Monomers 1 , 2 , and 7–9 were selected because of their improved stability toward hydrolysis. Moreover, different spacer lengths were chosen. The objective was to tune the hydrophilic/hydrophobic properties of the monomer and to study the influence of this parameter on various properties of the resulting dental materials. Monomers 3 and 4 were targeted in order to investigate the influence of the number of hydroxyl groups on the monomer performance. Monomers 5 and 6 were selected because of their structural similarity with HEMA and their ease of synthesis. The polymerization behavior of these monomers as well as their influence on the water sorption and solubility of corresponding polymer networks was first investigated. The properties of self-etch adhesives (dentin and enamel shear bond strength) and luting composites (mechanical properties) based on monomers 1–9 were subsequently studied. The influence of the monomer structure (nature of the polymerizable group, spacer length, number of hydroxyl groups, etc.) on the measured properties is discussed.

2
Materials and methods
2.1
Materials
Ethyl 2-(hydroxymethyl)acrylate 1 and Ivocerin ® were purchased from Synthon Chemicals GmbH (Germany). 2,3-Dihydroxypropyl methacrylate 3 was purchased from ABCR (Germany). 4-(2,2-Dimethyl-1,3-dioxolan-4-yl)butan-1-ol 10 as well as ethyl 2-(4-hydroxy-2-oxabutyl)acrylate 2 and N -methyl- N -(2-hydroxyethyl)acrylamide 7 were synthesized according to procedures reported in the literature . Aerosil OX50 and R709, 2,2-bis[4-(2-hydroxy-3-methacryloyloxypropoxy)-phenyl]propane (Bis-GMA), HEMA and glycidyl methacrylate were purchased from Evonik (Germany). Boron trifluoride diethyl etherate was purchased from Alfa Aesar (Germany). MDP was purchased from Orgentis (Germany). Camphorquinone (CQ) was purchased from Rahn (Switzerland). Bis-[(2-methacryloyloxyethoxy-carbonyl)-amino]-2,2,4(2,4,4)-trimethylhexane (UDMA) and N , N ′-diethyl-1,3-bis-(acrylamido)propane (DEBAAP) were produced by Ivoclar Vivadent AG (Liechtenstein) and MinAscent Technologies GmbH (Germany), respectively. Ytterbium fluoride was purchased by Sukgyung AT (Korea). All other reagents were purchased from Sigma-Aldrich (Switzerland). Column chromatographies were performed on Macherey-Nagel silica gel 60 (40–63 μm).
2.2
Measurements
1 H NMR spectra were recorded on a DPX-400 spectrometer using TMS as internal reference for spectroscopic chemical shifts. Data are given in the following order: chemical shift in ppm, multiplicity (s, singlet; br s, broad singlet; d, doublet; t, triplet; m, multiplet), coupling constant in Hertz, assignment. The viscosity of the monomers was measured at 23 °C with an Anton-Paar Rheometer MCR 302 using a CC17-SN3382 measuring system.
2.3
Syntheses
2.3.1
Synthesis of monomer 4
2.3.1.1
4-(2,2-Dimethyl-1,3-dioxolan-4-yl)butyl methacrylate 11 ( Fig. 2 )
Methacryloyl chloride (42.8 g, 409.5 mmol) was added dropwise, at 0 °C, to a solution of alcohol 10 (67.95 g, 390 mmol) and triethylamine (43.6 g, 430 mmol) in dichloromethane (DCM) (500 mL) ( Fig. 2 ). The reaction mixture was stirred for 10 min at 0 °C and for 3 h at room temperature (RT). Deionized water (250 mL) was added and the solution was stirred for 30 min. The phases were separated and the aqueous phase was extracted with DCM (1 × 250 mL). The combined organic layers were dried over sodium sulfate and the solvent was removed under reduced pressure. Hexane (150 mL) was added and the mixture was filtered through a pad of aluminum oxide (150 g). The solution was concentrated under reduced pressure. 62.2 g (256.7 mmol) of the desired product were isolated.
Yield: 66%. Aspect: colorless oil. 1 H NMR (400 MHz, CDCl 3 ): δ = 1.35 (s, 3H, CH 3 ); 1.41 (s, 3H, CH 3 ); 1.39–1.76 (m, 6H, CH 2 ); 1.94 (s, 3H, CH 3 ); 3.51 (t, 3 J HH = 6.5 Hz, 1H, CH 2 O); 4.01–4.13 (m, 2H, CH 2 O and CHO); 4.15 (t, 3 J HH = 6.5 Hz, 2H, CH 2 OCO); 5.54–5.57 (m, 1H, C CH 2 ); 6.10 (s, 1H, C CH 2 ).
2.3.1.2
5,6-Dihydroxyhexyl methacrylate 4
Pyridinium p -toluenesulfonate (PPTS, 1.13 g, 4.5 mmol) was added to a solution of methacrylate 11 (9.53 g, 39.3 mmol) in methanol (MeOH) (250 mL). The reaction mixture was stirred for 16 h. The solution was concentrated under reduced pressure and further MeOH (250 mL) was added. The solution was stirred for 5 additional hours at RT and was concentrated under reduced pressure. The crude product was purified by column chromatography (eluent = hexane/ethyl acetate: 1/1). 5.95 g (29.4 mmol) of the desired product were isolated.
Yield: 75%. Aspect: slightly yellow oil. 1 H NMR (400 MHz, CDCl 3 ): δ = 1.35–1.60 (m, 4H, CH 2 ); 1.64–1.76 (m, 2H, CH 2 ); 1.94 (s, 3H, CH 3 ); 2.13 (br s, 1H, OH); 2.31 (br s, 1H, OH); 3.44 (dd, 2 J HH = 10.9 Hz, 3 J HH = 7.5 Hz, 1H, CHC H 2 O); 3.67 (dd, 2 J HH = 10.9 Hz, 3 J HH = 3.0 Hz, 1H, CHC H 2 O); 3.69–3.77 (m, 1H, CHO); 4.17 (t, 3 J HH = 6.5 Hz, 2H, CH 2 OCO); 5.54–5.57 (m, 1H, C CH 2 ), 6.10 (s, 1H, C CH 2 ).
2.3.2
Synthesis of monomers 5 and 6
2.3.2.1
2-Hydroxy-3-methoxypropyl methacrylate 5
A solution of glycidyl methacrylate (43.90 g, 309 mmol) in MeOH (200 mL) was added dropwise, at 0 °C, to a solution of boron trifluoride diethyl etherate (1.80 g, 12.7 mmol) in MeOH (450 mL). The mixture was stirred for 1 h at RT. The solvent was removed under reduced pressure. The crude product was dissolved in ethyl acetate (100 mL) and hexane (200 mL) was added. The cloudy solution was filtered through a pad of silica gel and the solvent was removed under reduced pressure. The material was purified by distillation (bp: 57 °C (0.02 mbar)). 41.26 g (237 mmol) of the desired monomer were isolated.
Yield: 77%. Aspect: colorless liquid. The NMR spectroscopic data of the major isomer is provided below. 1 H NMR (400 MHz, CDCl 3 ): δ = 1.95 (s, 3H, CH 3 ); 2.56 (br s, 1H, OH); 3.40 (s, 3H, CH 3 ); 3.43 (dd, 2 J HH = 9.6 Hz, 3 J HH = 6.1 Hz, 1H, C H 2 OCH 3 ); 3.49 (dd, 2 J HH = 9.6 Hz, 3 J HH = 4.3 Hz, 1H, C H 2 OCH 3 ); 4.01–4.09 (m, 1H, CH OH); 4.22 (t, 3 J HH = 5.0 Hz, 2H, CH 2 OCO); 5.58–5.61 (m, 1H, C CH 2 ); 6.13 (s, 1H, C CH 2 ).
2.3.2.2
2-Hydroxy-3-ethoxypropyl methacrylate 6
Monomer 6 was synthesized according to the procedure described for the synthesis of monomer 5 . Glycidyl methacrylate (28.80 g, 203 mmol) and dry ethanol (400 mL) were used for the synthesis. The monomer was purified by distillation under vacuum (bp: 67 °C (0.03 mbar)). 24.25 g (129 mmol) of the desired monomer were isolated.
Yield: 64%. Aspect: colorless liquid. The NMR spectroscopic data of the major isomer is provided below. 1 H NMR (400 MHz, CDCl 3 ): δ = 1.21 (t, 3 J HH = 7.0 Hz, 3H, CH 2 CH 3 ); 1.96 (s, 3H, CH 3 ); 2.60 (br s, 1H, OH); 3.43–3.59 (m, 4H, CH 2 O); 4.01–4.10 (m, 1H, CH OH); 4.18–4.28 (m, 2H, CH 2 OCO); 5.58–5.62 (m, 1H, C CH 2 ); 6.14 (s, 1H, C CH 2 ).
2.3.3
Synthesis of monomers 8 and 9
2.3.3.1
N -Methyl- N -(3-hydroxypropyl)acrylamide 8
Monomer 8 was prepared from 3-(methylamino)propan-1-ol (54.05 g, 606 mmol) according to the procedure described for the synthesis of monomer 7 . 43.25 g (302 mmol) of the desired monomer were isolated.
Yield: 50%. Aspect: colorless liquid. 1 H NMR (CDCl 3 ): δ = 1.63–1.80 (m, 2H, CH 2 ); 2.93 and 3.01 (2s, 3H, CH 3 N); 3.40–3.62 (m, 4H, CH 2 OH and CH 2 N); 4.10 (br s, 1H, OH); 5.59 and 5.67 (2dd, 2 J HH = 2.0 Hz, 3 J HH = 10.5 Hz, 1H, CH C H 2 ); 6.24 and 6.27 (2dd, 2 J HH = 2.0 Hz, 3 J HH = 16.8 Hz, 1H, CH C H 2 ); 6.53 and 6.62 (2dd, 3 J HH = 10.5 Hz, 3 J HH = 16.8 Hz, 1H, C H CH 2 ).
2.3.3.2
N -Methyl- N -(6-hydroxyhexyl)acrylamide 9
Magnesium oxide (6.00 g, 148.9 mmol) was dispersed for 15 min in a mixture of 6-(methylamino)hexan-1-ol (4.05 g, 30.9 mmol), tetrahydrofuran (THF) (30 mL) and deionized water (12 mL). The solution was then cooled down to −10 °C. A solution of acryloyl chloride (2.72 g, 30.0 mmol) in THF (10 mL) was added dropwise to the reaction mixture. After stirring for 2 h at 0 °C, the mixture was filtered through Celatom ® . The solvent was removed under reduced pressure and the crude product was purified via column chromatography (first ethyl acetate/acetonitrile (3/1) than ethyl acetate/methanol (9/1)). 3.17 g (17.1 mmol) of the desired product were isolated.
Yield: 57%. Aspect: slightly yellow oil. 1 H NMR (CDCl 3 ): δ = 1.27–1.47 (m, 4H, CH 2 ); 1.52–1.66 (m, 4H, CH 2 ); 1.76 (br s, 1H, OH); 2.99 and 3.05 (2s, 3H, CH 3 N); 3.35 and 3.45 (2t, 3 J HH = 7.5 Hz, 2H, CH 2 N); 3.62 and 3.65 (2t, 3 J HH = 6.4 Hz, 2H, CH 2 O); 5.67 and 5.68 (2dd, 2 J HH = 2.0 Hz, 3 J HH = 10.4 Hz, 1H, CH C H 2 ); 6.32 and 6.33 (2dd, 2 J HH = 2.0 Hz, 3 J HH = 16.8 Hz, 1H, CH C H 2 ); 6.56 and 6.58 (2dd, 3 J HH = 10.5 Hz, 3 J HH = 16.8 Hz, 1H, C H CH 2 ).
2.4
Photopolymerization procedure
Photopolymerizations were carried out with a Perkin Elmer differential scanning calorimeter (DSC, Pyris Diamond). 0.5 mol% Ivocerin ® was added to each monomer as a photoinitiator. The experiments were performed under a stream of nitrogen. A sample (ca. 1.0 mg) of each mixture was placed in an uncovered aluminum DSC pan. The DSC chamber was purged with nitrogen for 4 min. The acquisition was then started. After 1 min of acquisition, the samples were irradiated for 2 min at 37 °C with a LED curing light (Bluephase, monowave LED with a maximum intensity at 470 nm, Ivoclar Vivadent AG). The incident light intensity was about 20 mW cm −2 . Each experiment was repeated three times. The heat flux was monitored as a function of time using the DSC under isothermal conditions. Double-bond conversion (DBC) was calculated as the quotient of the overall heat of polymerization evolved [Δ H p (J g −1 )] and the theoretical heat of polymerization obtained for 100% conversion of the mixtures [Δ H 0 p (J g −1 )] (Eq. (1) ).
D B C = 100 * Δ H p Δ H 0 p
Δ H 0 p was calculated according to the following formula (Eq. (2) ):
where Δ H 0 i is the theoretical heat of polymerization of monomer i (i = methacrylates or monomer 1 or monomer 2 , Δ H 0 i = 54.8 kJ mol −1 ; i = N -(methyl)acrylamides, Δ H 0 i = 74.7 kJ mol −1 ) and M i its molar mass.
The rate of polymerization ( R p ) was calculated according to the following formula:
where Q is the heat flow per s during the reaction and m the mass of the mixture in the sample.
2.5
Water sorption and solubility
UDMA/monomers 1–9 : 1/1 (wt/wt) mixtures were prepared. Ivocerin ® (0.2 wt%) was added as photoinitiator. A similar mixture based on HEMA was also formulated as a reference. For each mixture, specimen discs (diameter = 15.0 mm, depth = 1.0 mm) were prepared and cured with a visible light source (Spectramat SP2 polymerization unit, Ivoclar Vivadent AG) for 2 × 3 min (3 min for each side of the disc). Water sorption and solubility were measured according to ISO 4049 .
2.6
Luting composite formulation
Luting composites C1-C10 based on monomers 1–9 as well as on HEMA were formulated. Those formulations contain 8.83 wt% of monofunctional monomer (HEMA or alternative monomer), 35.67 wt% of UDMA, 0.27 wt% of CQ, 0.26 wt% of ethyl 4-(dimethylamino)benzoate (EDAB), 37.83 wt% of fumed silica OX-50 and 17.14 wt% of ytterbium fluoride (YbF 3 ). The fumed silica OX-50 was modified with the silane coupling agent 3-methacryloyloxy-propyltrimethoxysilane prior to use. The composites were prepared as follows: CQ and EDAB were added to the monomer mixture and the solution was stirred until complete dissolution of the photoinitiator system. Both fillers were subsequently added. The composites were then mixed in a three roll mill (Exakt Apparatebau, Norderstedt, Germany). Mechanical properties were measured after storage in distilled water at 37 °C for 24 h, according to ISO 4049 . The flexural strength (FS) and flexural modulus of elasticity (E) were determined using a Z 010 universal testing machine (Zwick, Germany).
2.7
Adhesive formulation and shear bond strength measurement
One-step self-etch adhesives based on monomers 1–9 as well as on HEMA were formulated. Those formulations contain 10.00 wt% of monofunctional monomer (HEMA or alternative monomer), 35.40 wt% of DEBAAP, 10.00 wt% of MDP, 20.00 wt% of Bis-GMA, 15.00 wt% of water, 5.00 wt% of isopropanol, 0.90 wt% of CQ, 0.42 wt% of EDAB, 1.25 wt% of bis(2,4,6-trimethylbenzoyl)-phenylphosphine oxide (Irgacure 819), 0.03 wt% of butylated hydroxytoluene (BHT) and 2.00 wt% of fumed silica Aerosil R709. For SBS measurements, freshly extracted bovine mandibular incisors were embedded in unsaturated polyester resin (ViscoVoss). Flat dentinal and enamel surfaces were prepared with 120-grit and 400-grit wet silicon carbide paper on the labial side of the embedded teeth. The adhesive was first rubbed on the prepared surface (dentin or enamel) with a microbrush for 20 s. The adhesive layer was strongly air dried and light cured for 10 s with a LED curing light (Bluephase G2, polywave LED with a broadband spectrum from 385 to 515 nm and 2 maxima at 410 and 470 nm, Ivoclar Vivadent AG). A polyethylene mold with a central 2.38 mm diameter circular hole was fixed on the surface. A composite (Tetric EvoCeram ® , Ivoclar Vivadent AG) was inserted in the mold and light-cured (Bluephase G2) for 20 s. The samples were finally stored in water at 37 °C for 24 h before being tested. The SBS was measured using a Z010 universal testing machine (Zwick, Germany) at a crosshead speed of 0.8 mm min −1 . 10 samples were tested for each adhesive.
2.8
Statistical analysis
The experimental results were analyzed in a one-way analysis of variance (ANOVA) based on n-number of specimens: flexural modulus and strength testing (n = 8), shear bond strength (n = 10). For statistical significance the P -value was set below 0.05.
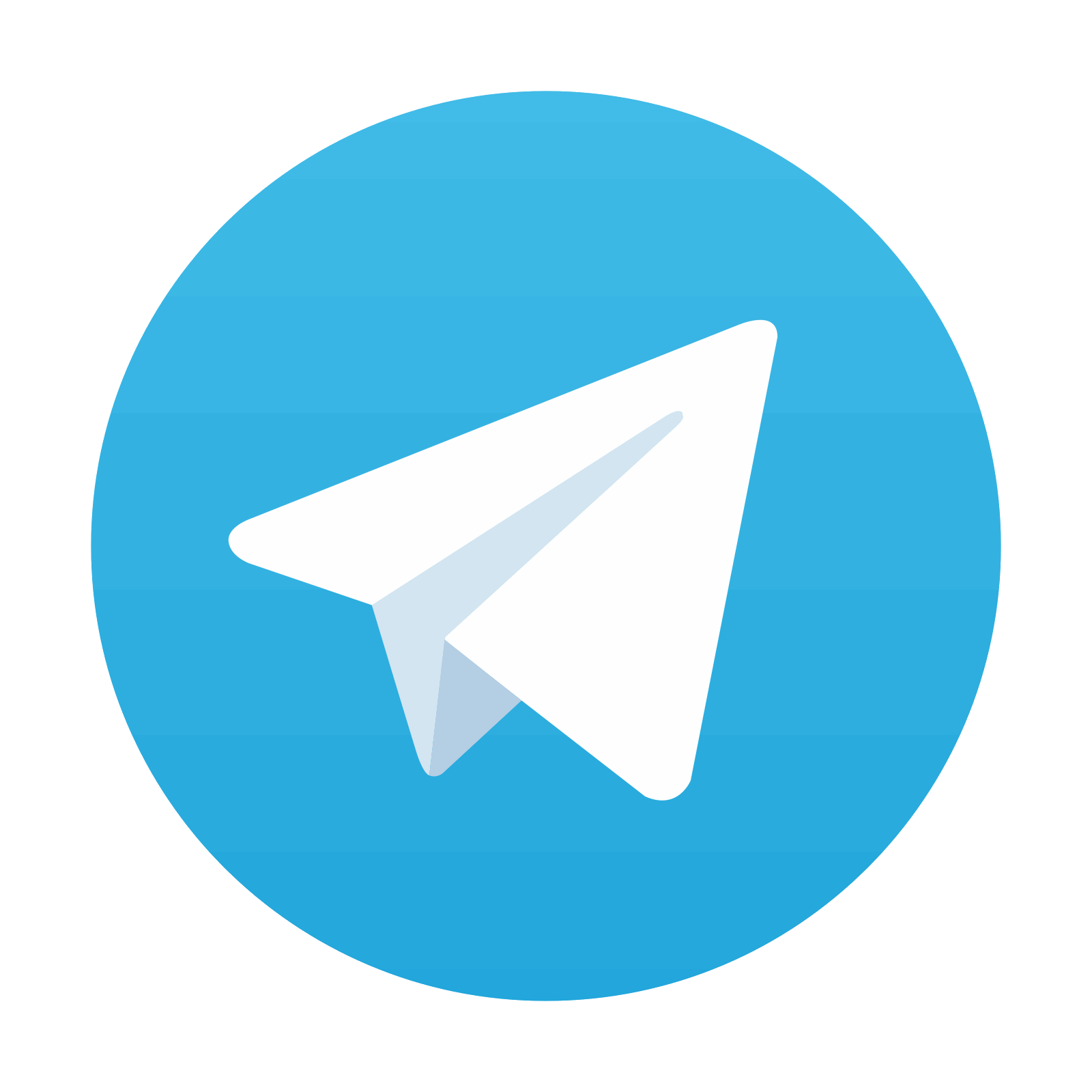
Stay updated, free dental videos. Join our Telegram channel

VIDEdental - Online dental courses
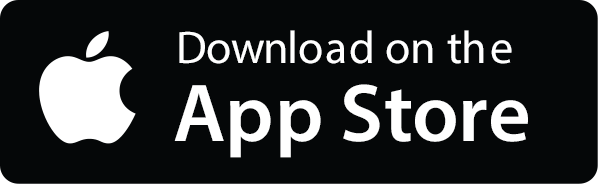
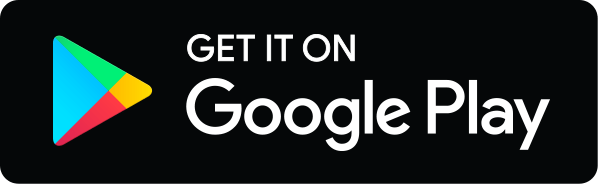