Introduction
The purposes of this study were to differentiate embryonic limb bud cells into cartilage, characterize the nodules produced, and determine their ability to heal a mouse skull defect.
Methods
Aggregated mouse limb bud cells (E12-E12.5), cultured in a bioreactor for 3 weeks, were analyzed by histology or implanted in 6 skull defects. Six controls had no implants. The mice were scanned with microcomputed tomography weekly. At 2 and 4 weeks, a mouse from each group was killed, and the defect region was prepared for histology.
Results
Chondrocytes in nodules were mainly hypertrophic. About 90% of the nodules mineralized. BrdU staining showed dividing cells in the perichondrium. Microcomputed tomography scans showed increasing minerals in implanted nodules that completely filled the defect by 6 weeks; defects in the control mice were not healed by then. At 2 and 4 weeks, the control skull sections showed only a thin bony layer over the defect. At 2 weeks, bone and cartilage filled the defects with implants, and the implants were well integrated with the adjacent cortical bone. At 4 weeks, the implant had turned almost entirely into bone.
Conclusions
Cartilage differentiated in the bioreactor and facilitated healing when implanted into a defect. Engineering cartilage to replace bone is an alternative to current methods of bone grafting.
Bone grafting has become a common procedure in dentistry. Autogenous bone grafting is essential in treating patients with cleft lip and palate and allows for placement of dental implants for missing teeth in the final stages of treatment. Other reasons that an alveolar cleft needs to be grafted include (1) closing the oronasal fistula, (2) allowing the teeth to erupt in the cleft region or allowing for orthodontic closure of the gap in the dental arch, (3) giving proper bony support to the teeth next to the cleft, (4) stabilizing the premaxillary segment of bilateral patients with bone support, and (5) creating support for the alar base. The iliac crest is often selected as a donor site because of the amount of bone available and good recovery in growing children.
In implant dentistry, ridge augmentation techniques are often needed before implant placement. Surgeons must advise patients of the availability of augmentation techniques that can significantly improve the functional and esthetic outcome of the implant. Ridge augmentation techniques improve implant placement position and allow proper emergence profiles. The reported implant success rates with maxillary and mandibular bone grafts vary from 61% in the maxilla to 98% in the mandible.
A deficient alveolar ridge can affect phonetics and esthetics. When the supporting bone is lost for pathologic reasons or from excision or trauma, it does not regenerate to its original dimension. Many materials and methods for augmentation of the deficient alveolar ridge have come and gone over the past 25 years. The advantages of autogenous bone grafts are that they are histocompatible, do not transfer disease, and retain viable osteoblasts that participate in the formation of new bone. Despite the desire for an alternative to autologous bone to simplify the surgical procedure and eliminate donor-site surgery and morbidity, autologous bone grafts are the most predictable and successful.
Although autogenous bone grafting is the gold standard, it also has shortcomings: a limited quantity of bone is available for harvest, and there is significant donor-site morbidity, including infection, pain, and increased anesthesia time. Cadaverous or biopsied bone allografts are alternatives, but these too can be in short supply. Furthermore, their efficacy can vary considerably, and they carry the serious risk of transmitting infectious disease.
Tissue engineering is a promising method to supply bone for craniofacial repair. In most of these studies, bone cells are cultured on a scaffold with or without the addition of growth factors and then implanted; however, a few laboratories have been developing cartilage systems for bone repair. The rationale behind these studies is that most of the bony skeleton forms embryonically through the process of endochondral ossification in which a cartilaginous template mineralizes, then vascularizes, and subsequently forms bone. In one study, 14-day-old chick sternal chondrocytes were cultured in sponges prepared from 4% acetylated chitosan, treated with retinoic acid to induce maturation into cartilage, and implanted subcutaneously in nude mice. Vascularization and new bone deposition in and around the cartilage and chitosan template was induced. Recently, another group placed cells from an embryonic mouse stem cell line on a carrier, differentiated them into cartilage, and implanted them into a defect in nude rats; extensive bone formation was observed. Our laboratory showed that embryonic mouse limb bud cells differentiated into mature, matrix-producing chondrocytes when cultured in a rotating bioreactor, which enhanced healing when implanted into skull defects.
We report results of a study that (1) assessed via histology the stage and amount of cartilage produced after 3 weeks of culture in a rotating bioreactor and (2) evaluated via microcomputed tomography (micro-CT) scanning and subsequent histology the ability of the cartilaginous spheroids produced in the bioreactor to heal a defect in a membranous bone.
Material and methods
All animals were handled in compliance with the Animal Welfare Act and NIH Policy and Guide, and all procedures were approved by the UTHSC-Houston Animal Welfare Committee. Fore and hind limb buds from E12 to E12.5 gestational age C57BL/6NH black mice (Harlan-Sprague-Dawley) were used to prepare cell suspensions. Limb buds were removed from embryos, minced, trypsinized, mechanically dissociated in culture medium, and filtered through Nitex (20 μm) (Tetko Co., Elmsford, NY) or a 70-μm cell strainer (BD Falcon, BD Biosciences, San Jose, Calif) to form a single-cell suspension with at least 2 × 10 6 cells per milliliter in a total of 9 mL of culture medium. The medium was BGJb-Fitton-Jackson modification (GIBCO, Invitrogen, Carlsbad, Calif) supplemented with 10% fetal bovine serum (Fisher Scientific International, Inc., Pittsburgh, Pa), 150 μg per milliliter of ascorbic acid, 1% penicillin-streptomycin solution (Sigma Aldrich, St Louis, Mo), and 0.25 μg per milliliter of Fungizone (GIBCO). To form aggregates, 4.5 mL of cell suspension was placed in each of 2 divided polystyrene petri dishes (BD Falcon) and cultured overnight on a rotating platform in an incubator (humidity; 37°C; 5% carbon dioxide). The next day, aggregates were placed in 50 mL bioreactor high-aspect ratio vessels (HARV, Synthecon, Inc., Houston, Tex) in the same incubator. Cultures were grown for 3 weeks, with half of the culture medium changed every other day. After 3 weeks, cartilage nodules were harvested and used for implantation, or photographed and fixed for histologic and immunohistochemical studies.
The synthetic thymidine analog bromodeoxyuridine (BrdU) is incorporated into a cell’s DNA when the cell divides. Antibodies against BrdU can be used to identify these cells, providing visual evidence of cell division. To determine the location of actively dividing cells, an experiment with BrdU was carried out. For this study, we used nodules that had been cultured for 2 weeks so that cells in most stages of differentiation could be seen in the nodule. Several nodules cultured for 2 weeks in the bioreactor were then cultured for 24 hours with 10 μmol/L BrdU (BD Pharmingen, San Diego, Calif). After fixing in formalin, the nodules were embedded in paraffin for immunohistochemistry. Sections on slides were deparaffinized and stained with the BrdU In-Situ Detection Kit (BD Pharmingen), which contains all components needed for the reaction. Sections were treated with BD Retrievagen A heated to 193°F for 10 minutes and then cooled to room temperature for 20 minutes. After rinsing with PBS, sections were treated with the biotinylated anti-BrdU antibody for 1 hour, rinsed again, and incubated for 30 minutes with Streptavidin-HRP (horseradish peroxsidase). After another rinse, the sections were incubated for 5 minutes with DAB (diaminobenzidine) substrate, then counterstained with hematoxylin, dehydrated, and mounted for observation. Nuclei with incorporated BrdU stained brown with this method.
For histologic studies, cartilage nodules were fixed in 10% buffered neutral formalin, embedded in paraffin, sectioned, and stained with either toluidine blue to assess cartilage differentiation and matrix production or von Kossa or alizarin red to assess mineralization. Sections of 6 nodules were used to determine the percentages of mineralization using the Image Pro Plus software (Media Cybernectics, Bethesda, Md). First, the count-size function was used to select specific pixels (in this case, black or dark brown) to be counted ( Fig 1 ). To measure the area of the entire nodule, the histology function was used; it highlighted the whole nodule, allowing its area to be determined. The percentage of each section mineralized was determined by dividing the area of the black or brown stained regions by the area of the entire nodule.
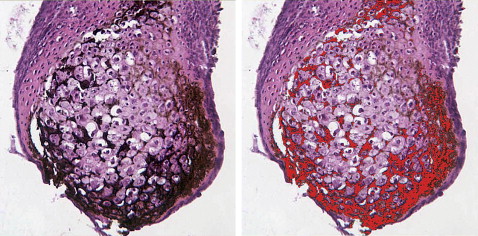
For surgeries, adult 8-week-old, C57BL/6NH mice, the same as the source for the cartilage spheroids cultured in the bioreactor, were anesthetized with 4% isoflurane initially and then maintained on 1.5% isoflurane during the procedure. The mice’s heads were shaved at the incision site and cleaned with a chlorhexidine solution. After a local subcutaneus injection with 0.25% bupivacaine (Marcaine, Hospira, Inc., Lake Forest, Ill), a 1-cm incision was made on the left parietal bone. The skin was separated, and, under saline-solution irrigation, a 2-mm circular defect was drilled with a trephine bur attached to a Micro Drill handpiece (11,000 rpm, Fine Scientific Tools, Foster City, Calif) in the superior portion of the left parietal bone. The parietal bone was chosen because of the ease of access and the lack of mechanical strength needed in this area. A 2-mm defect was created because many of our nodules fall into this size range. This is not a critical size defect, although the defect did not heal totally during the 6 weeks of imaging. The circular section of bone and periosteum was removed, and great care was taken not to damage the underlying dura. A cartilaginous nodule was implanted in the defects of 6 mice. The correspondence of the implant and defect sizes ensured that the implanted nodule would stay in place. Six mice served as controls without implants of the cartilaginous nodules. The incision was closed with 8-0 nylon swaged microsurgical sutures (S&T AG, Neuhausen, Switzerland)). All mice were treated immediately postsurgery and on the next 3 days with Tritop (Pharmacia and Upjohn, Bridgewater, NJ) ointment, which has antiseptic and anesthetic effects.
To assess healing over time, live mice were subjected to weekly (weeks 1-4 and week 6) micro-CT scanning carried out at the Small Animal Imaging Facility in the Department of Imaging Physics at M.D. Anderson Cancer Center, Houston, Texas, beginning 1 week after surgery, to visualize the new hard tissue. All animal procedures were approved by and performed under the guidelines of the University of Texas M. D. Anderson Cancer Center Institutional Animal Care and Use Committee. For the imaging procedure, each mouse was positioned prone on a half-pipe acrylic sled with a nose cone attached by plastic tubing to a vaporizer set to deliver 2% isoflurane (Halocarbon Laboratories, River Edge, NJ) with an oxygen flow rate of 2 L per minute.
The micro-CT system used was a tabletop CT scanner (model RS-9, General Electric Medical Systems, London, Ontario, Canada), a cone-beam CT geometry system designed to allow in-vivo imaging. The scanner gantry rotates in a step-and-shoot manner around the object bed, imaging the animal in 1 pass. The gantry has a tungsten anode x-ray source, operated at 80 kVp and 470 μA, fixed opposite a detector composed of a cesium iodide scintillator and a charge-coupled camera. For this free-breathing animal protocol, 360 projection images were taken at 1° increments, with each projection consisting of a 500-ms x-ray exposure.
A back-projection reconstruction method was used after the raw images were normalized against maximum and minimum x-ray exposure frames (ie, bright and dark fields); this corrected for any bad pixels in the detector and scaled the raw CT numbers. The initial reconstructed raw data CT-value grayscale was calibrated into Hounsfield units (HU) by sampling air, water, and bone material standards in the image’s field of view. With proper calibration, air has a value of –1000 HU, water has a value of 0, and bone (pure hydroxyapatite) has a value of approximately 2700 HU.
This micro-CT program was used to generate 3-dimensional renderings of the mouse skulls based on a minimum threshold signal. Any signal in the scan meeting that threshold or above will be shown, and any signal below the threshold will not be shown. The general rule is the greater the signal, the more mineral content in the tissue. All 3-dimensional renderings were generated at a threshold of 600, which we found to be the lowest CT number that corresponds to bone without incorporating nonbone (noise) as well.
One mouse of each treatment was killed after 2 and 4 weeks of implantation. Two weeks was chosen because previous studies have shown this time period to be propitious for the presence of both cartilage and bone in the implanted tissue. The skulls were removed, fixed, decalcified in EDTA, and the area containing the hole or implant was processed for histology. Bone formation was assessed by Alcian blue-Nuclear Fast Red-Tartrazine (Alcian Blue 8GX, Acros Organic, Geel, Belgium; Nuclear Fast Red and Tartrazine, Sigma Aldrich) which stains nuclei red, bone matrix yellow, cartilage blue, bone canaliculi blue-green, and cytoplasm light pink.
Results
Typical nodules are shown in Figure 2 , A and B . The irregular outline of the nodules is due to fusion of several aggregates into one. In 1 bioreactor vessel, after 2 weeks of culture, 12 aggregates were present, ranging in diameter from 0.1 to 2.1 mm. In a second vessel, 11 aggregates were formed, with sizes between 0.1 and 3 mm. Toluidine blue staining showed that the nodule was composed primarily of hypertrophic chondrocytes, although cells in other stages of the endochondral process of the embryonic limb bud—resting and proliferative—were seen ( Fig 2 , C ). The nodule was surrounded by layers of flattened and nonhypertrophic cells that varied from 2 to 10 cells thick ( Fig 2 , C and 3 , B ). The outermost cells of these layers were extremely flat. Metachromatic matrix was present in all areas of the nodule except between the flattened perichondrial-like cells. In some regions, it appeared that 2 or more nodules had aggregated and that the intervening perichondria had become incorporated in the interior of the newly formed nodule ( Fig 3 , C ).
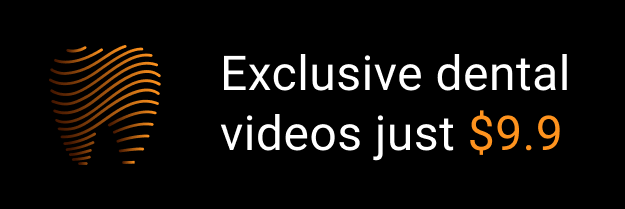