Learning Objectives
After reading this chapter, the student should be able to:
- 1.
Differentiate between stochastic and deterministic dental x-radiation biorisks and recognize which poses the greatest endodontic patient risk.
- 2.
Understand the basic design of a wall mounted x-ray unit and why it is preferred to hand-held x-ray units.
- 3.
Contrast and compare the differences between the indirect PSP plate sensors, the CCD solid state direct sensors and the CMOS solid state direct sensors used for intraoral endodontic radiography.
- 4.
Understand how shifting horizontal angles of the intraoral x-ray beam can be used to localize the relative buccal vs. lingual/palatal locations of superimposed root canals or jaw pathoses that superimpose teeth.
- 5.
Recognize FOV dimension measurements and how these relate to the coverage needed for most endodontic scan indications.
- 6.
Understand how voxel size affects storage space, uploading, downloading and transmission of CBCT volumes.
- 7.
Differentiate between DICOM file data and proprietary file data and how these interplay with various CBCT viewer software programs.
- 8.
Recognize how beam hardening and scatter artifacts degrade CBCT image quality.
- 9.
Review indications where CBCT imaging provides diagnostic and treatment advantages over the standard endodontic image requirements.
Prologue
Dr. William Herbert Rollins was already an established Harvard-trained physician and dentist when x-rays were discovered in 1895. Dr. Rollins, like his contemporaries, was fascinated by the usefulness of x-ray images. However, he was also one of the first to recognize the poorly understood―but genuine―risks associated with x-ray imaging.
Suffering radiation erythema to his hand in January 1898, and having seen the lethal effects of x-rays in his guinea pig experiments, Rollins attempted to caution his contemporaries of its dangers; however, the enthusiasm for the new x-ray technology was blinding radiographers to its morbid hazards. By the late 1920s, it was clear that there were biorisks associated with x-rays as the ranks of prominent radiographers in the New England area had been thinned. Further demonstrating this risk was the fact that at radiology meetings in the 1920s, roast beef was rarely served because the radiographers wore gloves to hide their scars and, by doing so, could not cut the roast beef with the cutlery provided.
It was not until the post-World War II atomic era, that the scientific community again began to heed the warnings of Rollins from a half century earlier.
Introduction
By virtue of its ability to image beyond the scope of the intraoral examination, radiography is an indispensable part of the diagnostic process in dentistry. Within this scope of oral health care, endodontics makes full use of this most useful of diagnostic techniques.
Imaging in the 21st century no longer relies solely on the use of x-rays for diagnostic imaging. Other imaging modalities from which dental care now benefits include optical scan imaging, magnetic resonance imaging, and on the frontier, ultrasound imaging. , However, with the advent of digital technology, x-ray imaging remains progressive with constant innovation. These innovations keep x-ray imaging an integral and up-to-date part of the diagnostic process for endodontic care.
The use of sensors in dental imaging has changed dramatically, from the earliest days of photographic emulsion on glass plates, to the development of plastics to support radiographic emulsion (i.e., film). In the 1980s, intraoral digital sensors were introduced by Dr. Frances Mouyen. Endodontics eventually embraced this technology because digital sensors dramatically facilitated the efficiency at which endodontic procedures could be completed.
In the 1960s endodontics became the eighth recognized dental specialty of the American Dental Association. At the same time, extraoral panoramic imaging became the exhilaration of dentistry with its ability to image large areas of the jaws in a single exposure. However, extraoral imaging was not as beneficial to the intricacies of endodontic patient care until the introduction of cone beam computed tomography (CBCT). In the 21st century, CBCT is a digital extraoral imaging modality that has greatly facilitated endodontic care. This chapter outlines the utility of radiographic imaging for endodontic patient care with the continued use of intraoral radiography as well as the progressive advances initiated with extraoral CBCT.
Radiation Biology
Contemporary clinicians must still deal with the reality that there are long-term risks that may be realized from exposure to x-radiation. Endodontic care will typically lead to multiple patient x-ray exposures. The radiation dosage to oral and other tissues has been calculated to be very low and extrapolated to cause minimal (but some) risk. , Table 3.1 illustrates the effective doses of various dental imaging procedures. This table provides the reader with a measure of the biorisk from intraoral exposures in relation to other dental imaging procedures. A more detailed radiation dosimetry review is available elsewhere. ,
Type of Radiographic Exam | Medain Effective Dose (μSv) | Days of Equivalent Background Radiation |
---|---|---|
Rectangular Collimation BW PSPP | 5 | 0.6 |
FMX PSP | 40 | 4 |
FMX CCD | 20 | 2.5 |
Round Collimation FMX D-speed | 400 | 48 |
FMX PSP – | 200 | 24 |
FMX CCD – | 100 | 12 |
Panoramic | 20 | –2.5 |
Cephalometric | 5 | 0.6 |
CBCT – large FOV (craniofacial) | 120 | 15 |
CBCT – medium FOV (full arch; dentoalveolar) | 100 | 12 |
CBCT -small FOV (∼3-5 teeth; <6.0cm. dia.) | 50 | 6 |
In the early days of x-ray imaging, deterministic biorisks, such as skin burns, scarring, and cataracts, were prominent, and the stochastic risks of malignancy (skin cancers and leukemias, etc.) were also very high. The reality of deterministic risks is negligible today, but the morbidity risk from x-ray–induced malignancies (i.e., stochastic risks) remains. , Practitioners must also be cognizant of the fact that the probability of getting an x-ray–induced malignant disease increases with increased numbers of exposures. With each exposure from each new endodontic procedure, small but cumulative increases in the probability of suffering an x-ray–induced malignancy exist. However, the endodontist must also remember that the overall patient x-ray biorisk is not merely from endodontic x-ray procedures. Risk will increase and be added to from additional endodontic procedures, such as other dental or medical imaging needs (e.g., dental implants, cardiovascular imaging, brain scanning, gastrointestinal [GI] series, orthopedic imaging, etc.). Therefore the risks remain real, and the need for vigilance to keep radiation exposures as low as reasonably achievable (i.e., ALARA) remains forever present. The value of imaging for any endodontic diagnosis or treatment indication should be determined on an individual basis to assure that the benefit-to-risk assessment supports the use of imaging.
In pediatric patients, the biorisks from the x-radiation are greater than the adult risks for a given radiation dose. This increased risk is due to the pediatric patient’s inherently greater radiosensitivity and because children have more remaining years of life during which a radiation-induced malignant neoplasm could develop. Hence, as with any imaging modality, the decision to use any diagnostic x-radiation is an objective-laden thought process where the benefits of the information obtained must outweigh the risks of the x-radiation.
Equipment for 2D Image Capture
Intraoral X-ray Units
Standard Wall-Mounted Units
Dental x-ray units have changed very little over the years since David Coolidge designed the first enclosed x-ray tube and tube head, the Victor CDX, in 1919.
Exposure times have decreased to less than 20% of the exposures from as little as three decades ago. Because of this, the need for large x-ray tube heads housing the larger generators needed to produce kV potentials greater that 70 kV has decreased. The need for high milliamperage (i.e., mA settings greater that 10 mA) is also no longer indicated with the shorter exposure times needed for digital receptors. Most contemporary x-ray tube heads are smaller with kV potentials between 60 and 70 kV and mA in the 6- to 8-mA range. With some exposure times now less than 0.10 seconds, most units have digital microprocessor timers that can reproducibly generate direct current (DC) exposures in the 0.05- to 0.10-second range. These smaller x-ray units produce adequate x-radiation to expose contemporary digital receptors, including American National Standards Institute (ANSI) F speed film, and reduce patient radiation dose with the shorter exposure times
Another option available in dental x-ray units is the recessed x-ray tube. Instead of the x-ray tube placed adjacent to the window within the tube head and the position indicating device (PID), contemporary x-ray tube heads have the x-ray tube recessed further away from the window ( Fig. 3.1 ). The recessed tube produces a longer focal distance (x-ray source-to-object distance), which projects a sharper image outline. With an increased focal distance, the inverse square law dictates that the exposure must be increased. However, even though the exposure needs to be greater to obtain a diagnostic image density, the patient dose is actually decreased, which is an added benefit of the recessed x-ray tube. This benefit is because the increased distance will prevent many of the low-energy x-ray photons in the beam from reaching the patient. Thus the recessed x-ray tube acts as a distance filter, which will decrease the patient dose.

Handheld Units
In the past decade, handheld x-ray units for dental imaging have become very popular in clinical practice. ,
Handheld units were originally designed for the benefit of mobility to provide dental imaging in remote locations where standard equipment is not available or for nonambulatory patients in need of dental services who cannot access standard dental facilities. Because these units are made for portability, they have become more popular in regular office designs and are used as a single unit for multiple standard operatories. Image quality is generally acceptable; however, due to the portability, the maximum kV and mA are less than those possible with standard units. The biggest issue with handheld units is the radiation dose to the operator if used without proper training. However, units have been shown to have adequate shielding to protect the operator if used properly with a backscatter shield.
Proper usage includes assuring that backscatter shields are attached to the units so that the operators can keep their bodies within the protective range of the shield ( Fig. 3.2 ).

Because of their convenience and popularity, several handheld units are now commercially available. There is concern that some newer units do not have the same degree of protective operator shielding. This aspect is a critical feature when considering a handheld unit of choice.
Despite their convenience for remote venues, the units lack the power of the wall-mounted units. However, smaller power packs are necessary to keep the unit at an appropriate lighter weight for the handheld use of the typical office staff member. Hence, remote charging and reinserting battery packs become an additional maintenance task. Care must also be taken when lifting and handling the nonergonomic units to avoid shock damage.
Furthermore, there is concern that the features of portability do present challenges for optimal image quality and safety. Consequently, unless clinical facilities demonstrate distinct advantages of handheld units over wall-mounted units where the latter installations are possible, the routine practice of using handheld units over wall-mounted units is not always a recommended guideline.
Intraoral Image Receptors
As described in this chapter’s introduction, the types of sensors used in dental imaging have changed dramatically. Digital sensors are the preferred type of sensor for endodontic management. Although it has been shown that the digital image quality has no major advantages over film images, digital sensors are by far more practical than film. Digital sensors are advantageous in that there is reduced patient radiation exposure, with increased speed of image acquisition, storage, retrieval, and transmission. An additional advantage of digital sensors over film is that digital sensors forego the arduous chemical film processing, which takes longer and also requires the use of a darkroom. Digital sensors have dramatically facilitated the efficiency at which endodontic procedures can be completed.
Types of Digital Image Receptors
Digital image formats include indirect photostimulable phosphor (PSP) plates and the direct solid-state sensors that may either be the charge-coupled device (CCD) sensor or the complementary metal-oxide semiconductor (CMOS) active pixel sensor. The latter is also abbreviated as CMOS-APS.
Photostimulable Phosphor Plates
The PSPs are considered indirect because, after exposure, the sensor must be removed from the patient’s mouth and transferred to a laser scanner so that the stored latent electronic charges of the pixels on the sensor’s surface can be scanned with a laser light to generate an electrical signal. The digital process includes assigning a numerical value to the electrical signal strength of each of the pixels. These numerical values are then assigned a gray-scale value to be used by the imaging software for display on a monitor.
Solid-State (Direct) Sensors
The solid-state direct sensors transmit the electronic signal straight from the sensor directly to a computer so the image can be displayed in as little as 3 to 5 seconds or less, depending on the computer processing speed and the server’s size and efficiency.
At this time, the CMOS is the predominantly used direct sensor. They are generally less expensive to manufacture and are faster in transmitting the electronic signal for processing and require a simple USB connection to a computer. Despite the improved CCD sensitivity to x-radiation, their slightly greater manufacturing costs and their more cumbersome digital processing sequences have now decreased their commercial availability.
Despite the advantages and increased preference for direct digital sensors, there remain some disadvantages, which delay an industry-wide total conversion to these sensors. Direct digital sensors are thicker (5 to 8 mm) and less comfortable than the thinner and more flexible traditional film or PSPs. Because of this discomfort, not all patients tolerate imaging procedures with direct sensors. Although the direct digital sensors have the approximate height and width of conventional film sensors, the actual active sensor area is smaller, ranging among different manufacturers from 20% to 25% reduction of the image surface area compared with conventional film or PSPs. ,
This problem creates issues with cut-off apices from the compromised vertical dimension and missing apices when the horizontal dimension is compromised, and horizontal angulation is needed to image the multiple root apices of multirooted teeth.
Dental Film
For those clinicians still using dental film, “F”-speed film is the film recommended for endodontic use. However, the slower “D”-speed film is still available, the increased radiation exposure and slightly higher contrast are not of any added benefit for endodontic imaging. Although the active surface areas are larger on film (as previously discussed), this technology is being replaced by direct sensor technology. For clinicians still using dental film, a more detailed discussion of their use and chemical film processing is discussed in other reference sources.
Techniques for Intraoral Image Capture
Periapical Imaging
The main image capture techniques for periapical imaging are the paralleling technique and the bisecting angle technique. These techniques are amply described in main radiology textbooks. For the paralleling technique, the receptor is ideally positioned parallel to both the long axis and the mesiodistal plane of the tooth being evaluated. The beam is then directed perpendicular to the plane of the receptor. It is necessary to stabilize the receptor in this planned position. When it is not possible to place the receptor parallel to the tooth, the bisecting angle technique may then be applied. With this technique, the receptor is stabilized against the lingual side of crown and the adjacent palatal/lingual mucosa. Because the receptor no longer parallels the long axis of the tooth, it is necessary to project the vertical angle of the x-ray beam perpendicular to the plane that bisects the angle formed by the intersecting planes of the long axis of the tooth and the vertical axis of the receptor. Frequently, clinicians choose to align all these planes freehand, with the use of simple receptor bite blocks or with other simple holding instruments (modified hemostats, Snap-a Ray devices, etc.), but without the aid of beam alignment devices ( Fig. 3.3, A and B ). However, for more predictable imaging, receptor stabilizing–beam alignment devices are available for this purpose. These instruments are preferred for best image quality with minimal projection artifact, such as cone cutting, foreshortening, elongation, and so on ( Fig. 3.3, C ).

When working length images or master cone images are needed, the rubber dam clamp does make it more cumbersome to position the receptor. Again, the use of the receptor stabilizing–beam alignment devices best facilitate obtaining the most favorable images. Specially adapted instruments are made with appropriate bite block modifications to accommodate the rubber clamp and endodontic files positioned in the canals ( Fig. 3.3, D ).
Due to the variability of human nature, it is not always possible to get patient cooperation to tolerate the placement of the instruments necessary for beam alignment. This difficulty is especially true for the “working” radiographs taken during an endodontic treatment with the rubber dam in place. Sometimes, it may require cooperation by the patient if they hold the sensor in position. In these scenarios, trying the modified paralleling technique is recommended . Essentially, the sensor is not parallel to the tooth, but the central beam is oriented at right angles to the receptor surface. In endodontic working radiographs, a further modification is made by varying the horizontal beam angle.
Tube Shift
Modification of the horizontal angle of the x-ray beam becomes a necessary modification to separate structures that superimpose one another on the 2D image. Principles of relative movement of structures and sensor orientation are applied to the differentiation of object position, as demonstrated in Figs. 3.4 and 3.5 .


These principles of tube shift have been adapted to object localization of structures in the nonvisible buccolingual dimension of 2D radiography. One of the most useful techniques in endodontics is the SLOB Rule. SLOB is an acronym― S ame L ingual O pposite B uccal, as first described by Richards.
When two objects and the sensor are in a fixed position buccal and lingual from each other, and the radiation source is moved in a horizontal or vertical direction, the images of the two objects move apart in opposite directions ( Fig. 3.6 ).

One way to visualize this effect is to close one eye and hold two fingers directly in front of the open eye so that one finger is superimposed on the other. By moving the head one way and then the other, the position of the fingers relative to each other shifts. The same effect is produced with two superimposed roots (the fingers) and the way in which they move relative to the radiation source (the eye) and the central beam (the line of sight). When the cone-shift technique is used, it is critical to know in which direction the shift was made and to determine what is buccal and what is lingual. Otherwise, serious errors may occur. Fig. 3.7 demonstrates how the horizontal angle is shifted to accomplish these localization techniques.

This horizontal tube-shifting technique can be used to separate superimposed root canals and superimposed roots or to separate roots of teeth from adjacent superimposing anatomy, such as the mental foramen in the mandible or the zygomatic process of the maxilla in the maxillary arch. Examples of this tube shift are demonstrated in Fig. 3.11, A – F , of the next section.
- 1.
The major biorisk from x-rays used for endodontic imaging is:
- a.
Deterministic
- b.
Traumatic
- c.
Cyclic
- d.
Stochastic
- a.
- 2.
A disadvantage of digital solid-state sensors for periapical imaging is the:
- a.
Smaller area for image capture
- b.
Projection of double images
- c.
Greater propensity for dimensional distortion
- d.
Inferior diagnostic sensitivity to dental film
- a.
- 3.
In comparison with handheld x-ray units, wall-mounted x-ray units:
- a.
Are more durable
- b.
Have greater portability
- c.
Produce less image distortion
- d.
Process digital images more quickly
- a.
- 4.
Handheld x-ray units:
- a.
Improve image resolution
- b.
Increase biorisk to the radiographer
- c.
Require shorter exposure times
- d.
Are used only with digital sensors
- a.
- 5.
Tube shifting is a
- a.
CBCT technique that improves image resolution
- b.
A method of directing the rotational path in a CBCT scanner
- c.
Beam alignment method that minimizes root canal length distortion
- d.
Technique used to localize the buccal and lingual position of objects
- a.

Endodontic Imaging Needs
There are numerous indications in endodontic patient management where imaging plays a vital role in decision making and treatment rendering. These indications range from diagnosing a treatment indication, to rendering the treatment, and then to follow-up of the treatment. These situations are outlined later in this section ( ).
Disease Diagnosis
Before diagnosing any disease process, the clinician must be able to distinguish the normal anatomic range of structures in the dentoalveolar complex and its supporting structures. This range would include the root and pulp anatomy of any affected dentition and their relationship to adjacent structures, whether maxillary molar roots adjacent the zygomatic process and maxillary sinus in the maxilla or the apices of mandibular premolars adjacent the mental foramen. Pulp chambers are identified within the dental crown, and the root canals will extend from the pulp chamber to its specific root apex. The periodontal ligament (PDL) space is differentiated from the pulp chamber as it follows the outline of roots and has a consistent narrow width (<1.0 mm) around the roots. It also will have a peripheral thin radiopaque lamina dura adjacent to it ( Fig. 3.8 ).

Identifying Pathosis
The recognition of disease requires an understanding of the pulpal, periodontal, or periapical changes that may arise in the presence of disease and how these changes can affect the identification of the normal anatomic outlines. Similarly, an understanding of systemic or local hard tissue pathologic processes that affect the jaws is also necessary to recognize these processes as they present. The more astute clinicians recognize these changes in their earliest stages of tissue alteration. It is also important to remember that pathologic processes in their earliest stages are often symptomatic before the development of prominent radiographic signs or clinical signs or symptoms ( Fig. 3.9 ).
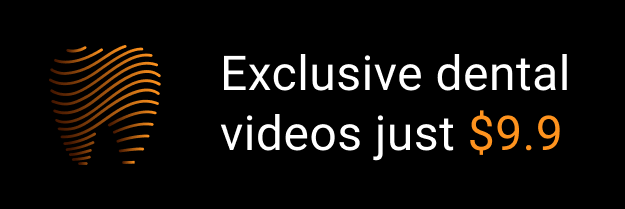