Introduction
The long duration of orthodontic treatment is a major concern for patients. A noninvasive method of accelerating tooth movement in a physiologic manner is needed. The aim of this study was to evaluate of the efficacy of low-intensity laser therapy in reducing orthodontic treatment duration and pain.
Methods
Twenty patients requiring extraction of first premolars were selected for this study. We used a randomly assigned incomplete block split-mouth design. Individual canine retraction by a nickel-titanium closed-coil spring was studied. The experimental side received infrared radiation from a semiconductor (aluminium gallium arsenide) diode laser with a wavelength of 810 nm. The laser regimen was applied on days 0, 3, 7, and 14 in the first month, and thereafter on every 15th day until complete canine retraction was achieved on the experimental side. Tooth movement was measured on progress models. Each patient’s pain response was ranked according to a visual analog scale.
Results
An average increase of 30% in the rate of tooth movement was observed with the low-intensity laser therapy. Pain scores on the experimental sides were significantly lower compared with the control sides.
Conclusions
Low-intensity laser therapy is a good option to reduce treatment duration and pain.
A major concern to orthodontic patients is treatment time. Generally, the time required for fixed appliance treatment is 20 to 30 months. Reducing the treatment time requires increasing the rate of orthodontic tooth movement. Many studies have examined different methods that can increase the rate of orthodontic tooth movement, including local injections of prostaglandins, 1, 25(OH) 2 D 3 (the active form of vitamin D 3 ), osteocalcin, and relaxin around the alveolar socket. Although these substances stimulate the rate of tooth movement, they also have the undesirable side effects of local pain and discomfort during the injections. Recently, electric stimulation and resonance vibration have been tried in animals, but these methods require an apparatus that is not routinely used in dental practice.
Ablative use of lasers has become common in dental practice. Low-intensity laser therapy has an energy output that is low enough so as not to cause the temperature of the treated tissues to rise above 36.5°C or normal body temperature. Low-intensity laser therapy in its initial days was applied only in medical sciences such as orthopedics, surgery, and medicine. It is used to accelerate the callus formation at fracture sites to facilitate wound healing. Saito and Shimizu found that low-intensity laser therapy can accelerate bone regeneration in the midpalatal suture during rapid palatal expansion and stimulate the synthesis of collagen, which is major matrix protein in bone. In the last decade, many histologic studies have attempted to determine the effect of low-intensity laser therapy on the histochemical pathways directly associated with orthodontic tooth movement. Increased osteoblastic and osteoclastic activity after low-level laser therapy was observed in vivo and in vitro. Few studies in the literature have examined the effect of low-intensity laser therapy on the rate of orthodontic tooth movement, and most were short-term animal studies.
Thus, the aims of this study were to evaluate the effect of low-intensity laser therapy on the rate of orthodontic tooth movement and also to study its analgesic effect during orthodontic tooth movement.
Material and methods
Our sample consisted of 20 healthy orthodontic patients (8 male, 12 female; ages, 12-23 years). Patients with a history of long-term medication were excluded because nonsteroidal anti-inflammatory drugs and hormone supplements are known to interfere with bone metabolism. Patients with unilateral chewing or parafunctional habits, skeletal crossbite, and occlusal interferences were also excluded. Periodontally compromised patients were excluded because poor bone quality can affect orthodontic tooth movement, and the development of mobility can lead to incorrect measurement of orthodontic tooth movement. Impacted canines and canines with dilacerated roots were excluded from the study because dilacerated roots make orthodontic tooth movement difficult with a greater possibility of root resorption.
The sample size was determined by power analysis based on the results of the pilot study that showed that the rate of tooth movement was twice that of the control side.
η={Z1−α/2√P(l−Pα)+Zl−β√PO(l−Pα)}2(Pα−PO)2PO=PopulationproportionPα=Sampleproportionα=Significancelevell−β=Power
η= { Z 1− α / 2 P ( l − P α ) + Z l − β P O ( l − P α ) } 2 ( P α − P O ) 2 P O = Population proportion P α = Sample proportion α=Significance level l−β= Power
With a permissible error of 5% by using a split-mouth design, a sample size of 20 was sufficient for the study to have 80% power and be clinically significant.
The study design was approved by the ethics committee of Government Dental College and Hospital, Nagpur, Maharashira, India, according to the guidelines of Health University.
Routine orthodontic diagnostic records were collected and analyzed for all subjects. The treatment plans for these patients included extraction of the maxillary or mandibular first premolars (or both) to meet the requirements of space for the retraction of anterior teeth. A randomly assigned incomplete block split-mouth design was used to prevent interindividual biologic variation. In 20 patients, the maxillary first premolars were extracted, but the mandibular first premolars were extracted in only 10 patients. In each patient, the extracted right and left quadrants were randomly divided into 2 groups. The patients were blinded about the experimental and control sides. Group 1 was the control side quadrant and did not receive low-intensity laser therapy. Group 2 was the experimental side quadrant and received laser therapy. Each group consisted of 30 quadrants.
On day 7 after the extractions, separators were placed mesially and distally to the first molars for band placement. After 2 days of separator placement, molar bands (0.180 × 0.005 in) were custom made. Triple molar tubes were welded on the buccal surfaces, and a lingual sheath was welded on the lingual surface of the maxillary molar bands. On the mandibular molar bands, double molar tubes were welded. Transpalatal arch 20-gauge (0.9 mm) stainless steel wire was adapted on the model. Maxillary bands with a transpalatal arch inserted in the lingual sheath were cemented with glass ionomer luting cement (D-tech, Pune, India). The transpalatal arch was placed for anchorage reinforcement and secured with an elastic module.
Preadjusted edgewise McLaughlin Bennett Trevisi brackets (Ortho Organizers, Carlsbad, Calif) of 0.022-in slot were bonded with Transbond XT (3M Unitek, Monrovia, Calif), and curing was done with a light-emitting diode (Dentsply International, York, Pa). Alignment and leveling were initiated with 0.016-in heat-activated nickel-titanium wire, and later sequences of wires were 16 × 22-in nickel-titanium, 17 × 25-in nickel-titanium, 17 × 25-in stainless steel, and 19 × 25-in nickel-titanium. After alignment and leveling, a final working wire was placed: 19 × 25-in stainless steel (Orthoforce; G&H Wire, Franklin, Ind).
After 21 days of 19 × 25-in stainless steel wire placement, individual canine retraction was started with a nickel-titanium closed-coil spring (G&H Wire). The incisors were consolidated by using 0.009-in steel ligature wires. The second premolar and the first molar were also consolidated to make a single anchorage unit. A constant force of 150 g was used for canine retraction on both the control and experimental sides. To maintain the force of 150 g in all patients in spite of the different widths of available extraction spaces, accurate spring selection was done by using the manufacturer’s guide. The kit of the nickel-titanium closed-coil spring system (G&H Wire) contains springs of 9 and 12 mm in length. They are again subdivided into feather light, extra light, light, medium, and heavy forces. The measuring gauge supplied by manufacturer with the spring kit has a hole to simulate the eyelet of the spring. This hole is slipped over the canine hook; the closest landmark to the molar hook then shows the recommended spring size to be selected to achieve a force of 150 g. The spring was positioned from the first molar tube hook to the power arm of the canine bracket and also secured with a ligature tie to the bracket. The exerted force value was confirmed with an orthodontic dynamometer. Patients were asked to report immediately if the spring dislodged or broke; it was then replaced.
Low-intensity laser therapy was started on the selected experimental side on the same day as placement of the coil spring.
In 2 patients, the nickel-titanium coil spring became detached. In both instances, the failures occurred unilaterally, from the canine hooks. They were replaced within 12 hours.
Informed consent was obtained from each patient or parent (for patients less than 18 years of age) for laser irradiation. The laser type used was a semiconductor (aluminium gallium arsenide) diode (model LA3D0001.1; LAMBDA S.p.A., Vicenza, Italy) emitting infrared radiation with a wavelength of 808 ± 10 nm operated according to the manufacturer’s recommendations. All safety precautions for the patient and the operator were followed. This medical equipment has a wide range of settings according to the required treatment.
For analgesic purposes, the settings were adjusted to a wavelength of 800 nm, a continuous wave mode, an output power of 0.7 mW, and an exposure time of 30 seconds. For bio-stimulation, the parameters were set at a wavelength of 800 nm, a continuous wave mode, an output power of 0.25 mW, and an exposure time of 10 seconds.
The hand piece has a cylindrical quartz tip with a surface area of 4 mm 2 from where the laser beam is emitted. The black color-coded needle (used for therapeutic purposes) was attached to the hand piece for low-intensity laser therapy. The routine method of sterilization and disinfection was followed. In particular, the hand piece body and the optic tips were sterilized by cold sterilization.
Protection glasses were worn by both the operator and the patient. These glasses, provided by the manufacturer, were in accordance with the European norm EN 207 and had a optical density of ≥5 at the wavelength of emission from the diode.
Low-intensity laser therapy was started on the day of placement of the nickel-titanium coil spring for analgesia. Two irradiations were done. One irradiation was done on the middle third of the canine root on the buccal side, and the second on the palatal side holding the laser tip in direct contact with the tissues. On day 3, low-intensity laser therapy was started for bio-stimulation. A total of 10 irradiations were done: 5 on the buccal side ( Fig 1 ) and 5 on the palatal side. To cover the entire periodontal fibers and alveolar process around the canines, the distribution and order were as follows.
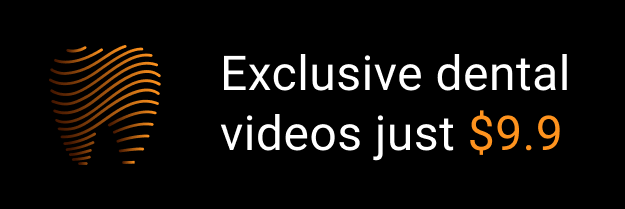