Abstract
Objectives
The application of an electric field has been shown to positively influence the bonding of dentin bonding systems (DBS) by improving adhesive impregnation into dentin. However, the mechanism responsible for this phenomenon has not been completely elucidated. The aim of this study was to clarify the effects of pH, matrix ionic strength, and applied voltage on the migration of commonly used DBS monomers in a model matrix (agarose gel).
Methods
Some common monomers examined were bis-GMA (2,2-bis[4-(2-hydroxy-3-methacryloyloxy propoxy) phenyl] propane); HEMA (2-hydroxyethyl methacrylate); 2-MP (bis[2-(methacryloyloxy) ethyl] phosphate); TCDM [di(hydroxyethyl methacrylate) ester of 5-(2,5,-dioxo tetrahydrofurfuryl)-3-methyl-3-cyclohexenyl-1,2-dicarboxylic acid]; and TEGDMA (triethylene glycol dimethacrylate). Agarose gels poured into a horizontal 10-well electrophoretic cell were used to mimic the collagen fibrils of the dentin organic matrix. The role of pH, matrix ionic strength, and voltage on monomer migration was assayed by modifying the experimental conditions.
Results
Results of experiments performed at pH 3.1, 6.3, 8.5, and 12.3; at low, medium, and high ionic strength; and at 50 and 100 V clearly showed that DBA monomer migration toward both the anode and the cathode can be affected by each of these parameters.
Significance
Migration of acrylic monomers toward the anode or cathode can be achieved as desired by selective choice of pH, ionic strength, and applied voltage. Additional studies are needed to evaluate the synergistic effects of DBS monomer blends on migration in an electric field.
1
Introduction
Dentin bonding systems (DBS) are used to couple relatively hydrophobic restorative materials to hydrophilic surfaces such as vital dentin. These blends of hydrophilic and hydrophobic monomers can be classified as etch-and-rinse or self-etch (or etch-and-dry) adhesives and can be used in multi-step processes (e.g., three-step etch-and-rinse or two-step self-etch) or in a simplified combined process (etching-primer–bonding) in clinical applications (i.e., two-step etch-and-rinse or one-step self-etch) . Because simplified DBS formulations involve mixing non-solvated adhesives with solvated primers (i.e., two-step etch-and-rinse) or with self-etching primers (i.e., one-step self-etch), the addition of solvents strongly increase the hydrophilicity of the resin mixture and of the bond .
Dentin bonding occurs through penetration of DBS monomers into the dentin substrate . Etch-and-rinse adhesives impregnate the delicate layer of demineralized collagen fibrils that is first exposed by the separate etching agent (which is rinsed away) , whereas self-etch DBS, because they are intrinsically acidic, simultaneously demineralize and impregnate the dentin . Because neat co-monomer blends are too viscous to infiltrate into wet demineralized dentin matrices, solvents are added to DBS blends to reduce their viscosity and favor the impregnation process .
DBS from many manufacturers have solvent concentrations of 8–49% . Volatile solvents, such as ethanol or acetone, are added to decrease viscosity, increase molecular mobility and improve polymerization in etch-and-rinse systems, whereas water is frequently added to self-etch adhesives for the same purpose and to allow for dissociation of weak acids .
Both etch-and-rinse and self-etch adhesives infiltrate the demineralized dentin structure passively via a diffusion mechanism in which the water within the insoluble collagen fibrils is replaced by solvent and DBS monomers. The hybrid layer resulting from the impregnation process, which can be likened to an in situ hybridization process, results in a structure in which collagen fibrils, hydroxyapatite, and the residual smear layer created by dentin preparation are mixed with DBS monomers and residual solvents . Several silver nitrate impregnation studies have demonstrated that incomplete resin infiltration into etched dentin frequently occurs because silver can be seem to electron microscopy to permeate hybrid layers even in the absence of a detectable interfacial gap, causing what is called “nanoleakage” . The more homogenous and compact the hybrid layer, the less silver uptake can be detected and the more stable and durable the bond; conversely, the more porous the hybrid layer, the higher the uptake of silver nanoleakage along the adhesive interface, resulting in reduced immediate bond strength and accelerated degradation .
The use of an electrical device (ElectroBond 3.0/2005, Seti, Rome, Italy) was recently reported to favor DBS application to dentin . The results of preliminary in vitro studies were promising. They confirmed that the application of DBS under the influence of an electric field increases the bond strength to dentin and reduces the amount of interfacial nanoleakage . While the use of ElectroBond is still not common in clinical dentistry, different prototypes have been tested in different labs with current intensities ranging from 25 to 175 μA . Iontophoresis was suggested to positively influence monomer impregnation and favor the dentin-water substitution rate, in a manner similar to be reported for various medicaments and local anesthetics . A recent study also suggested that the use of electric current improves wettability of dentin surface following application of phosphoric acid and a mild self-etch primer . However, despite the finding that application of DBS under the influence of an electric field increases the bond strength, the mechanisms underlying the proposed mechanism of action—the electric field forces monomers into the dentin substrate, favoring formation of the hybrid layer—remain unclear.
The aim of the present study was to better clarify the effects of pH, ionic strength of the matrix, and strength of applied voltage on dentin impregnation by common DBS monomers under the influence of an electric field. Agarose gel was used to simulate the demineralized dentin matrix. The tested null hypothesis was that monomer migration within organic matrices subjected to an electric field is not dependent on pH, ionic strength (buffer concentration), or electrical voltage.
2
Materials and methods
2.1
Reagents
Bis-GMA (2,2-bis[4-(2-hydroxy-3-methacryloyloxy propoxy) phenyl] propane), HEMA (2-hydroxyethyl methacrylate), 2-MP (bis[2-(methacryloyloxy) ethyl] phosphate, TCDM di(hydroxyethyl methacrylate) ester of 5-(2,5,-dioxo tetrahydrofurfuryl)-3-methyl-3-cyclohexenyl-1,2-dicarboxylic acid), and TEGDMA (triethylene glycol dimethacrylate) were purchased from Sigma Chemical (St. Louis, MO, USA) and used without further purification ( Table 1 ).
Low-electroendosmosis agarose gel D-1 (EEO = 0.09–0.13 by Weime method; sulfate < 0.14%) with a gelling temperature of 36–39 °C, a melting temperature of 87–89 °C, and a gel strength of 1.200 g/cm 2 was purchased from Shelton Scientific Inc (Shelton, CT, USA).
2.2
Experimental procedures
Agarose gel powder (0.5 g) was suspended in 100 mL of aqueous buffer at pH 3.1 (2.1% citric acid/1% sodium citrate), 6.3 (1% citric acid/2.9% sodium citrate), 8.5 (3.8% sodium tetraborate boric acid), or 12.3 (0.4% sodium hydroxide). Each buffer was precisely titrated to its nominal pH with HCl 4 N before use. Each agarose gel suspension was heated at full power in a 500-W microwave oven for 2 min to completely solubilize the agarose, poured into a horizontal 10-well electrophoretic cell (Owl EasyCast Horizontal System B1, Thermo Scientific, Portsmouth, NH, USA), and allowed to cool to room temperature. The final gel dimensions were 9 cm × 11 cm, and electrophoresis system buffer capacity was 600 mL. The tested monomers (bis-GMA, HEMA, 2-MP, TCDM, and TEGDMA) were dissolved in ethanol at 5% (w/w) concentration.
A voltage of 50 or 100 V was applied to the cell electrodes using a DC Power Supply (Apelex PS304 MinipacII, Massy, France), and both current and voltage were monitored during experiment. NaCl was added to the buffer used for electrophoresis to adjust their ionic strength to maintain the same electrical current/intensity ratio for all tests without monomer addition (data not shown). The buffers were adjusted so that the current at 100 V was 100 mA (highest ionic strength), 50 mA, or 25 mA (lowest ionic strength).
The dissolved monomers (10-μL aliquots) were injected into the wells of the gel, and voltage was applied for 30 min. After electric field application, the gel was carefully removed from the electrophoretic cell and placed onto a layer of polyethylene film on top of a silica thin-layer chromatography plate (Sigma Chemical) ( Fig. 1 ). The silica plate was used to enhance visualization of the monomers; most of the monomers do not fluoresce at 254 nm, and their optical absorbance at that wavelength was too low to provide clear images of their diffusion patterns without the silica plate underneath. The polyethylene film was used to avoid water migration from the gel to the silica. Images were obtained under an ultraviolet (UV) lamp at 254 nm (8 W; VL-4.LC, LTF Labortechnik, Wasserburg/B, Germany) in a darkroom using a Coolpix P1 8MP camera (Nikon, Tokyo, Japan).
In additional tests, the order of the monomers in the wells was changed to control for any effects of neighboring samples on monomer migration. The monomers were chosen to include hydrophilic neutral monomers commonly used in dental adhesives (i.e. Bis-GMA and TEGDMA), a neutral hydrophilic monomer used in adhesive blends (i.e. HEMA) and two acid hydrophilic monomers used in adhesives (2-MP and TCDM). If any of the neutral monomers migrated in the electric field, it would provide evidence of electroendosmosis (EEO) under those conditions. Only the ionic acidic monomers should migrate toward the anode if they were dissociated.
2
Materials and methods
2.1
Reagents
Bis-GMA (2,2-bis[4-(2-hydroxy-3-methacryloyloxy propoxy) phenyl] propane), HEMA (2-hydroxyethyl methacrylate), 2-MP (bis[2-(methacryloyloxy) ethyl] phosphate, TCDM di(hydroxyethyl methacrylate) ester of 5-(2,5,-dioxo tetrahydrofurfuryl)-3-methyl-3-cyclohexenyl-1,2-dicarboxylic acid), and TEGDMA (triethylene glycol dimethacrylate) were purchased from Sigma Chemical (St. Louis, MO, USA) and used without further purification ( Table 1 ).
Low-electroendosmosis agarose gel D-1 (EEO = 0.09–0.13 by Weime method; sulfate < 0.14%) with a gelling temperature of 36–39 °C, a melting temperature of 87–89 °C, and a gel strength of 1.200 g/cm 2 was purchased from Shelton Scientific Inc (Shelton, CT, USA).
2.2
Experimental procedures
Agarose gel powder (0.5 g) was suspended in 100 mL of aqueous buffer at pH 3.1 (2.1% citric acid/1% sodium citrate), 6.3 (1% citric acid/2.9% sodium citrate), 8.5 (3.8% sodium tetraborate boric acid), or 12.3 (0.4% sodium hydroxide). Each buffer was precisely titrated to its nominal pH with HCl 4 N before use. Each agarose gel suspension was heated at full power in a 500-W microwave oven for 2 min to completely solubilize the agarose, poured into a horizontal 10-well electrophoretic cell (Owl EasyCast Horizontal System B1, Thermo Scientific, Portsmouth, NH, USA), and allowed to cool to room temperature. The final gel dimensions were 9 cm × 11 cm, and electrophoresis system buffer capacity was 600 mL. The tested monomers (bis-GMA, HEMA, 2-MP, TCDM, and TEGDMA) were dissolved in ethanol at 5% (w/w) concentration.
A voltage of 50 or 100 V was applied to the cell electrodes using a DC Power Supply (Apelex PS304 MinipacII, Massy, France), and both current and voltage were monitored during experiment. NaCl was added to the buffer used for electrophoresis to adjust their ionic strength to maintain the same electrical current/intensity ratio for all tests without monomer addition (data not shown). The buffers were adjusted so that the current at 100 V was 100 mA (highest ionic strength), 50 mA, or 25 mA (lowest ionic strength).
The dissolved monomers (10-μL aliquots) were injected into the wells of the gel, and voltage was applied for 30 min. After electric field application, the gel was carefully removed from the electrophoretic cell and placed onto a layer of polyethylene film on top of a silica thin-layer chromatography plate (Sigma Chemical) ( Fig. 1 ). The silica plate was used to enhance visualization of the monomers; most of the monomers do not fluoresce at 254 nm, and their optical absorbance at that wavelength was too low to provide clear images of their diffusion patterns without the silica plate underneath. The polyethylene film was used to avoid water migration from the gel to the silica. Images were obtained under an ultraviolet (UV) lamp at 254 nm (8 W; VL-4.LC, LTF Labortechnik, Wasserburg/B, Germany) in a darkroom using a Coolpix P1 8MP camera (Nikon, Tokyo, Japan).
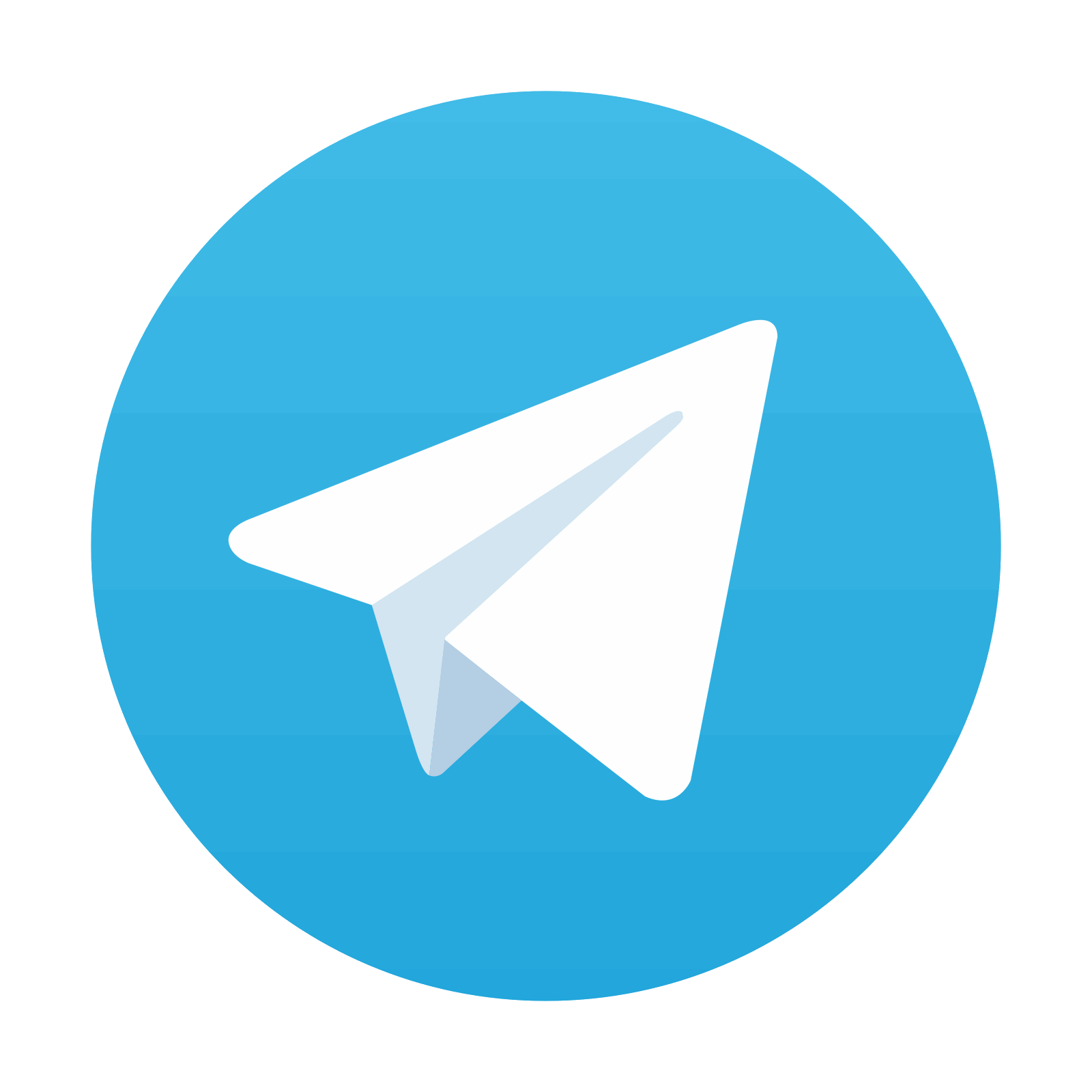
Stay updated, free dental videos. Join our Telegram channel

VIDEdental - Online dental courses
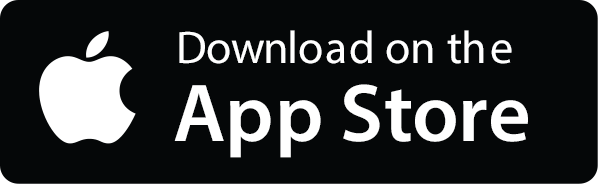
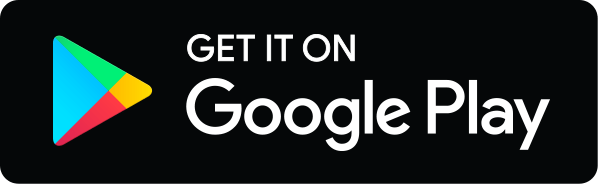