Abstract
Objectives
Leucite glass-ceramics with fine-grained leucite crystals promote improved mechanical strength and increased translucency. The objectives of the study were to optimize the microstructure of a fine-grained leucite glass-ceramic in order to increase its flexural strength and reliability as measured by its Weibull modulus.
Methods
Glass was prepared by a melt-derived method and ground into a powder (M1A). The glass crystallization kinetics were investigated using high temperature XRD and DSC. A series of two-step heat treatments with different nucleation/crystal growth temperatures and holds were carried out to establish the optimized crystallization heat treatment. Glass-ceramics were characterized using XRD, SEM and dilatometry. The glass-ceramic heat treated at the optimized crystallization parameters (M1A opt ) was both sintered (SM1A opt ) and heat extruded (EM1A opt ) into discs and tested using the biaxial flexural strength (BFS) test.
Results
High temperature XRD suggested leucite and sanidine crystallization at different temperatures. Optimized crystallization resulted in an even distribution of fine leucite crystals (0.15 (0.09) μm 2 ) in the glassy matrix, with no signs of microcracking. Glass-ceramic M1A opt showed BFS values of [mean (SD), MPa]: SM1A opt = 252.4 (38.7); and EM1A opt = 245.0 (24.3). Weibull results were: SM1A opt ; m = 8.7 (C.I. = 7.5–10.1) and EM1A opt ; m = 11.9 (C.I. = 9.3–15.1). Both experimental groups had a significantly higher BFS and characteristic strength than the IPS Empress Esthetic glass-ceramic, with a higher m value for the EM1A opt material ( p < 0.05).
Significance
A processable fine-grained leucite glass-ceramic with high flexural strength and improved reliability was the outcome of this study.
1
Introduction
Leucite glass-ceramics have been used in the construction of dental prostheses for over a decade . They were largely used for fusing to metallic substructures for high strength crown and bridge prostheses . Since the advent of adhesive dentistry, heat extrusion and CAD–CAM technology , this situation has changed. Leucite glass-ceramics are now used as all-ceramic materials that are etched and adhesively bonded by silane bonding agents and composite resins to etched tooth structure . Contemporary commercial leucite glass-ceramics however have a low reported flexural strength (120–140 MPa), and low toughness (1.3 MPa m 0.5 ) which is less than ideal for a restorative dental material. This is associated with the manufacturing process and the production of microstructures that lack homogeneity and often consist of large crystals and associated matrix microcracking . A crystallite size of less than 4 μm was established by Mackert et al. to avoid microcracking in leucite glass-ceramics. The inclusion of larger crystallite sizes in other glass-ceramic systems previously led to reductions in mechanical strength , where crystallite size dependant microcracking was accounted for by a Griffith flaw-energy balance criterion .
The relationship between the glass thermal properties and the high expansion leucite phase (22.3 × 10 −6 /°C) , with its cubic to tetragonal crystallographic transformation and the reversible 1.2% volume change are also important factors. Careful control of the difference in thermal expansion between the tetragonal leucite crystals and the glass matrix can be utilized to develop and maintain tangential compressive stresses around the crystals. These stresses are considered responsible for strengthening in leucite glass-ceramics .
A fine-grained microstructure is desirable to improve the mechanical strength and translucency in glass-ceramic composites. The current authors produced leucite glass-ceramics containing fine crystallites (<1 μm) with minimal matrix microcracking, which resulted in flexural strengths of 219–253 MPa . Optimization of these glass-ceramics may be possible to increase the leucite volume fraction or further reduce the crystallite size by changes to the processing and nucleation and growth parameters . The production of fine-grained leucite glass-ceramics may also reduce enamel wear and improve esthetics, due to the reduced abrasiveness and increased translucency provided by the fine crystallites. The intention was to create an esthetic restorative material that would be less prone to fracture and reduce enamel wear with the opposing dentition. The aims of the study were therefore to optimize the microstructure of a fine-grained leucite glass-ceramic in order to increase its flexural strength and reliability.
2
Materials and methods
2.1
Glass synthesis
A glass batch with composition (mol%: 74.6% SiO 2 , 10.7% Al 2 O 3 , 7.9% K 2 O, 2.1% CaO, 0.3% TiO 2 , 4.7% Na 2 O, 1.1% Li 2 O and 0.5% MgO) was heated in an electric kiln (Fredrickson Kiln Co., New York, USA) at 10 °C/min to 1316 °C (7 h hold). The frit was then cooled, ground and sieved to a maximum particle size of 125 μm. The glass powder (125 μm) was wet ground with 5 mm yttria stabilized zirconia (YTZ) grinding media for 120 min, followed by 120 min milling with 2 mm YTZ grinding media in an attritor mill (Model 1-S Lab Attritor, Union Process, Akron, USA). The resultant glass (M1A, D 50 = 1.79 μm) was used for the crystallization and flexural strength optimization study.
2.2
Differential scanning calorimetry analysis
Glass M1A (50 mg) frit was characterized by differential scanning calorimetry (DSC) using a Stanton Redcroft DSC 1500 (Rheometric Scientific, Epsom, UK) with matched pairs of platinum–rhodium alloy crucibles. Alumina was used as reference material. DSC measurements were performed at heating rates of 5, 10, 20, 25 and 40 °C/min within the temperature range of 25–1200 °C to evaluate the glass crystallization and activation energy of leucite crystallization.
2.3
High temperature X-ray diffraction
Glass M1A was investigated by high temperature X-ray diffraction (HTXRD) using an X’Pert Pro X-ray diffractometer (Panalytical, Almelo, The Netherlands), equipped with a high temperature furnace (Anton Parr HTK 16, Anton Parr, Graz, Austria). Flat plate / geometry and Ni-filtered Cu-Kα radiation ( λ 1 = 1.54059290 (50) Å and λ 2 = 1.54442740 (50) Å) was used . Glass powder was heated on a Pt strip in air at a rate of 1 °C/s. High temperature XRD measurements were taken at 20 °C temperature intervals from 500 to 1100 °C with a stabilizing time of 5 min before each measurement. Data was continuously collected from 10° to 80° (2 range) with a step size of 0.0167° and a step time of 26.67 s. Room temperature XRD of the crystallized glass-ceramics was also carried out with a step size of 0.0334° and a step time of 200.03 s. X’Pert Plus software (version 1.0, Philips Analytical, The Netherlands) was used to identify the crystalline phase using the ICDD PDF2 database (Newtown Square, PA, USA), where the diffraction patterns were compared with cubic leucite (ICDD: 01-076-2298), tetragonal leucite (ICDD: 00-038-1423) and sanidine (ICDD: 00-025-0618). Calibration was carried out with a NIST standard reference material 660a (lanthanum hexaboride). Crystal strain calculations were performed with the XRD data and Eq. (1) :
ε a = a − a 0 a 0 , ε c = c − c 0 c 0
where ε a and ε c are the strain of leucite in the a -axis and c -axis, a and c are the mean unit cell dimensions of tetragonal leucite for the tested glass-ceramics, a 0 and c 0 are the unit cell dimensions of the reference tetragonal leucite (ICDD: 00-038-1423).
2.4
Crystallization optimization study
Glass M1A powders (3.0 g) were mixed with 0.9 ml of modeling liquid (CH B: 24066, VITA, Zahnfabrik, Germany) and compacted in a rectangular steel mold with plunger under 3 × 10 5 Pa pressure for 60 s. After removal, the glass powder compacts were cut into sections (6 mm × 6 mm × 6 mm), placed on platinum foil and heat treated in a furnace (RHF 1600, Carbolite, Hope Valley, UK) at a ramp rate of 10 °C/min to explore the effects of temperature and hold on crystallization. In order to establish the optimal nucleation temperature, the glass compacts were heated from 23 °C to 600, 610, 620, 630, 640, 650, 660, 670, 680, 690 and 700 °C (1 h hold), then ramped to 1120 °C (1 h hold) before air quenching. Glass compacts were next heated from 23 °C to the optimal nucleation temperature for 0.5, 1, 2, 3, 4 and 5 h holds, then ramped to 1120 °C (1 h hold) to evaluate the nucleation duration.
Further experiments were carried out to explore the most favorable crystal growth temperatures and holds. The glass compacts were first heat treated using the optimized nucleation temperature and duration, followed by ramping to 1000, 1050, 1100 and 1120 °C (1 h holds). Various crystallization holds (0.5, 1, 2, 3, 4 and 5 h) at the optimized crystal growth temperature were also carried out to study the effects of holding time on crystal growth.
2.5
Secondary electron microscopy
Glass-ceramic specimens were embedded, polished and etched with 0.1% hydrofluoric acid for 60 s. The specimens were gold coated and viewed using a field emission scanning electron microscope (FEI Inspect F, Oxford Instruments, Oxfordshire, UK) in the secondary scanning imaging mode. Photomicrographs (2200×, area = 2210.4 μm 2 ) were used to quantify the leucite crystal size, number and area fraction using a light pen (270SD + INT-40, Trackballs, US), in conjunction with image analysis software (Sigma Scan Pro 5.0, Systat Software Inc., Chicago, USA). In order to compare leucite crystal sizes a one-way ANOVA on ranks was carried out and Dunn’s multiple comparison test ( p < 0.05).
2.6
Biaxial flexural strength (BFS) specimen preparation
Glass M1A was two-step heat treated using the optimized crystallization conditions, ground and sieved through a 125 μm sieve to produce glass-ceramic M1A opt powder. Disc specimens (30 per group) were fabricated by moistening 1.0 g of powder with 0.3 ml of modeling liquid (CH B: 24066, VITA) and compacting in a cylindrical steel mold with plunger (16 mm in diameter) under a hydraulic press. Glass-ceramic M1A opt powder (1.6 g) was dry pressed using a custom-made steel die (punch diameter 13.0 mm, Specac Ltd., Slough, UK) to produce powder ingots. Glass-ceramic powder disc compacts and ingots were sintered in a pre-heated (538 °C) dental porcelain furnace (Multimatt MCII, Dentsply, Konstanz, Germany) and sintered under vacuum at a rate of 38 °C/min to 1040 °C with 2 min hold. Disc specimens were wet ground to P1000 grade silicon carbide paper using a metalographic lapping machine (Struers, Knuth Rotor, Germany), followed by cleaning. One simulated stain firing in air from 538 °C to 780 °C without hold (ramp of 55 °C/min) and one simulated overglaze firing in air from 538 °C to 860 °C with a 0.3 min hold (ramp at 55 °C/min) were then carried out in a porcelain furnace.
A set of experiments were carried out to establish the heat extrusion cycle for glass-ceramic M1A opt . Perspex discs (14 mm × 2 mm) were sprued and invested using 200 g of IPS Empress Esthetic speed investment material (Lot: GL3038, Ivoclar-Vivadent, Schaan, Liechtenstein), vacuum mixed for 90 s with 32 ml of IPS Empress Esthetic investment liquid (Lot: GL3034, Ivoclar-Vivadent) and 22 ml distilled water. After 45 min, the investments were transferred to a preheated (850 °C) burnout furnace (5365, Kavo, Germany) and held for 45 min. Room temperature glass-ceramic ingots (M1A opt ) were heat extruded in an Optimal press furnace (Jeneric Pentron, Wallingford, CT, USA), at 1040 °C with a 15 min hold and 10 min press time. The investments were removed by sandblasting using 50 μm glass beads (Bracon Ltd., Etchingham, UK) at 1.5 × 10 5 Pa pressure. The specimens were wet ground to P1000 grade silicon carbide grinding paper on the compressive test surface only and the tensile test surface was left as sandblasted. Specimens were fired in a porcelain furnace (Multimatt MCII, Dentsply) using one simulated stain and one simulated overglaze firing cycles as described previously.
Glass-ceramic M1A 1 (2-step heat treated at 650 °C for 1 h and 1120 °C for 1 h) and heat extruded IPS Empress Esthetic glass-ceramic (Lot: H22624, ETC2, Ivoclar-Vivadent, Liechtenstein) used in a previous study were used as commercial and crystallization comparisons. The test surface of the IPS Empress Esthetic (EE) specimens were left as sandblasted (50 μm glass beads at 1.5 × 10 5 Pa pressure) and the compressive test surface wet ground to P1000 grade silicon carbide grinding paper. Specimens were then fired in a porcelain furnace (Multimatt MCII, Dentsply) according to the manufacturers’ recommended firing cycles (2 simulated stain and 2 simulated glaze firings).
2.7
Biaxial flexural strength testing
The biaxial flexural strength (BFS) of glass-ceramic groups (sintered M1A opt and heat extruded M1A opt ) was tested using a ball-on-ring test fixture. Disc specimens supported by a 10-mm diameter knife-edge were centrally loaded via a 4-mm diameter spherical ball indenter at a crosshead speed of 1-mm/min until specimen failure. The BFS was calculated using the Timoshenko and Woinowsky-Krieger equation :
σ max = P h 2 ( 1 + ν ) 0.485 × ln a h + 0.52 + 0.48
where σ max was the maximum tensile stress, P was the load measured at fracture, h was the specimen thickness, a was the radius of the knife-edge support and <SPAN role=presentation tabIndex=0 id=MathJax-Element-3-Frame class=MathJax style="POSITION: relative" data-mathml='v’>vv
v
was a Poisson’s ratio of 0.25.
2.8
Statistical analyses
The strength data was analyzed using a one-way ANOVA (Sigma Stat, version 2.03, SPSS Inc., Chicago, USA) and Tukey’s multiple comparison test ( p < 0.05). Weibull analysis of the different test groups was carried out using Weibullsmith software (Fulton Findings, USA). The biaxial flexural strength test values were ranked ascending. The double logarithm of 1/(1-median rank) was plotted vertically versus the logarithm of the actual data values and a straight line fitted through the points using the median rank regression methods. The equation of Weibull two parameter distribution function used was:
P f = 1 − exp − σ σ 0 m
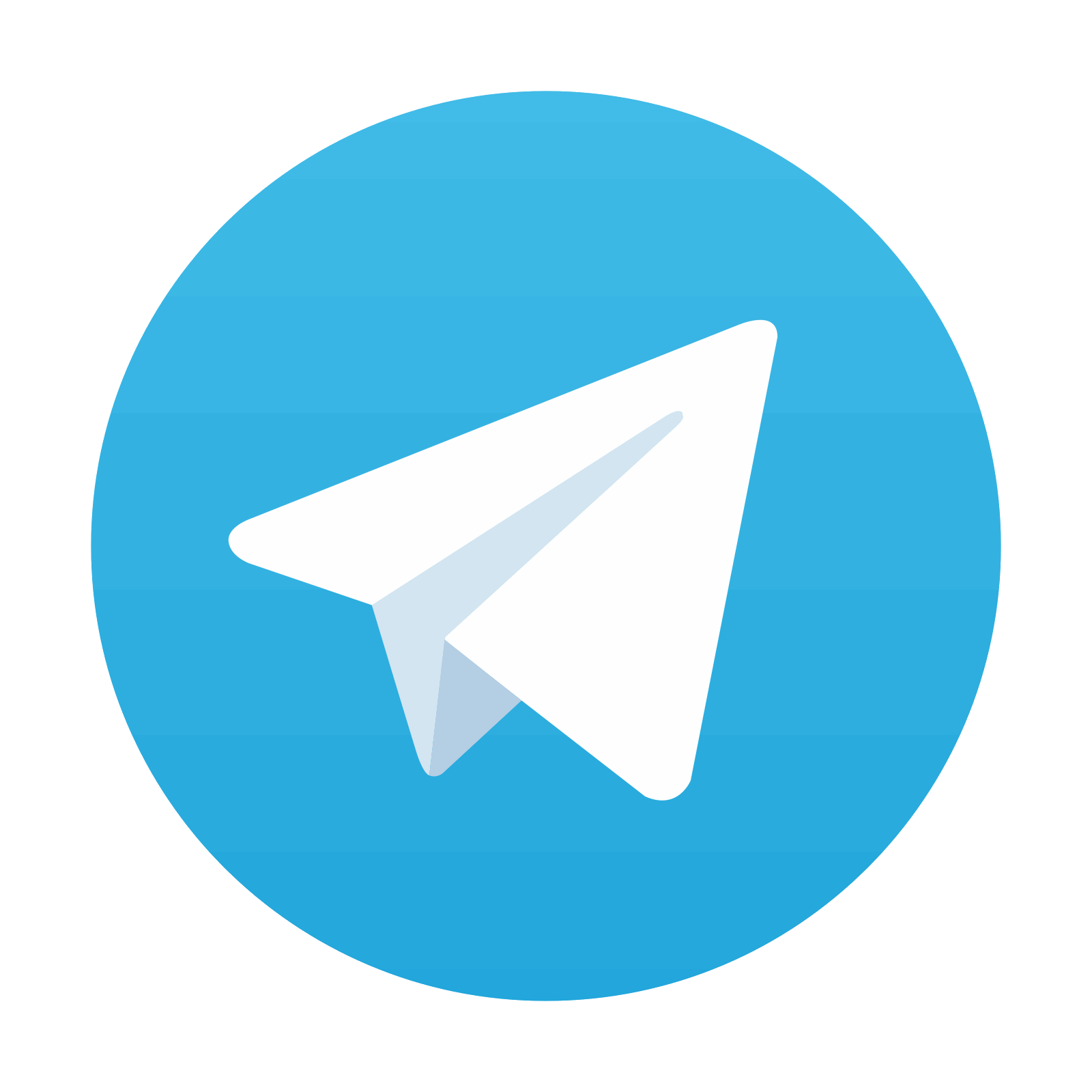
Stay updated, free dental videos. Join our Telegram channel

VIDEdental - Online dental courses
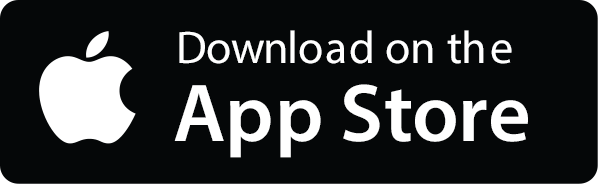
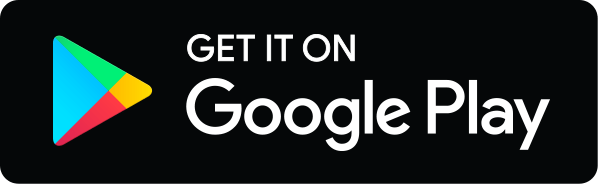