Highlights
- •
Alumina coating and glass infiltration can be used to effectively modify the intaglio (cementation) surface of zirconia-based restorations.
- •
Glass infiltration significantly improves the flexural strength of zirconia.
- •
Alumina coating has no substantial effect on the load-bearing capacity of zirconia.
- •
Monolithic zirconia mechanically outperforms its porcelain-veneered zirconia and lithium disilicate glass–ceramic counterparts.
Abstract
Objectives
To examine the effects of glass infiltration (GI) and alumina coating (AC) on the indentation flexural load and four-point bending strength of monolithic zirconia.
Methods
Plate-shaped (12 mm × 12 mm × 1.0 mm or 1.5 or 2.0 mm) and bar-shaped (4 mm × 3 mm × 25 mm) monolithic zirconia specimens were fabricated. In addition to monolithic zirconia (group Z), zirconia monoliths were glass-infiltrated or alumina-coated on their tensile surfaces to form groups ZGI and ZAC, respectively. They were also glass-infiltrated on their upper surfaces, and glass-infiltrated or alumina-coated on their lower (tensile) surfaces to make groups ZGI2 and ZAC2, respectively. For comparison, porcelain-veneered zirconia (group PVZ) and monolithic lithium disilicate glass–ceramic (group LiDi) specimens were also fabricated. The plate-shaped specimens were cemented onto a restorative composite base for Hertzian indentation using a tungsten carbide spherical indenter with a radius of 3.2 mm. Critical loads for indentation flexural fracture at the zirconia cementation surface were measured. Strengths of bar-shaped specimens were evaluated in four-point bending.
Results
Glass infiltration on zirconia tensile surfaces increased indentation flexural loads by 32% in Hertzian contact and flexural strength by 24% in four-point bending. Alumina coating showed no significant effect on resistance to flexural damage of zirconia. Monolithic zirconia outperformed porcelain-veneered zirconia and monolithic lithium disilicate glass–ceramics in terms of both indentation flexural load and flexural strength.
Significance
While both alumina coating and glass infiltration can be used to effectively modify the cementation surface of zirconia, glass infiltration can further increase the flexural fracture resistance of zirconia.
1
Introduction
Yttria-stabilized tetragonal zirconia polycrystals (Y-TZP) has high strength of 800–1200 MPa and fracture toughness of 4–6 MPa m 1/2 . It serves as an excellent material for all-ceramic restorations. However, zirconia is opaque and does not match the natural color of human teeth . Thus, zirconia restorations need to be veneered with esthetic porcelain to improve their esthetics. These bilayered structures often cause clinical complications in porcelain-veneered zirconia crowns and fixed partial dental prostheses (FDPs). This is because they are prone to chipping and delamination of the overlay porcelain by 25% after 31 months and 13% after 38 months in vivo . To overcome chipping complications in veneered zirconia structures, monolithic zirconia structures with improved esthetics have been developed . This was achieved by infiltrating glass into zirconia surfaces to provide shade options and better translucency .
Another limitation of zirconia crowns and FDPs is their insensitivity to the etching-silane bonding technique. To establish adequate zirconia-luting agent bonding strength, surface modifications of zirconia are required . Traditionally, airborne particle abrasion is used to modify the zirconia surface for mechanical retention . This process produces a protective layer of compressive stresses, which may impede fracture . It also introduces microcracks, which may accelerate failure . The formation of a compressively stressed layer in zirconia results from work hardening and volume expansion induced by a tetragonal–monoclinic phase transition . The microcrack generation during airborne particle abrasion is attributed to the mechanical impact of abrading particles on zirconia surfaces. Thus, airborne particle abrasion can either reduce or increase the flexural strength of zirconia, depending on the type and size of abrading particles, the air pressure applied and the surface conditions of zirconia. Given the high-cycle fatigue nature of mastication, surface cracks in a zirconia restoration can eventually extend beyond the compressive surface layer, diminishing the fatigue strength of the restoration. Strength reduction is a major cause for failures in ceramic restorations, due to the development of tensile stress-induced radial cracks at the cementation surface .
To improve the luting cement bonding strength, alumina coating and glass infiltration on zirconia cementation surfaces have been used. The non-invasive nanostructured alumina coating on zirconia surfaces can survive the thermal cycling, significantly improving the resin-bond strength to zirconia ceramics . The glass infiltration into zirconia surfaces has enabled the usage of the standard etching-silane technique to selectively remove the glass phase in the graded glass structure and create a three-dimensional surface morphology, increasing the resin bond strength . However, effects of these surface modifications on the fracture resistance of zirconia are not known.
Accordingly, the present study aims to investigate effects of zirconia surface modifications on its fracture resistance using Hertzian indentation and four-point bending techniques. Our research hypotheses are (1) glass infiltration and alumina coating on the cementation surface of zirconia monolith significantly increase its load bearing capacity; and (2) the load-bearing capacity of monolithic zirconia is significantly greater than commercial porcelain-veneered zirconia and monolithic lithium disilicate glass–ceramic structures.
2
Materials and methods
2.1
Specimen preparation
2.1.1
Fabrication of ceramic specimens
Five experimental zirconia groups and commercial ceramic compositions were utilized in this study, which are summarized in Table 1 .
Materials | Group abbreviations |
---|---|
Monolithic Y-TZP | Z |
Monolithic Y-TZP with glass infiltration (GI) on the cementation surface | ZGI |
Monolithic Y-TZP with glass infiltration on the cementation and occlusal surfaces | ZGI2 |
Monolithic Y-TZP with alumina coating (AC) on the cementation surface | ZAC |
Monolithic Y-TZP with alumina coating on the cementation surface and glass infiltration on the occlusal surface | ZAC2 |
Y-TZP veneered with overlay porcelain a | PVZ |
Monolithic lithium disilicate glass-ceramic b | LiDi |
Group Z were monolithic Y-TZP specimens. The material was made from 28 nm diameter powders of Y-TZP containing 5.18 wt% Y 2 O 3 (TZ-3Y-E grade, Tosoh, Tokyo, Japan) for green compacts of 80 mm × 64 mm × 15 mm using cold isostatic press at 200 MPa. The green compacts were cut into blocks of 16 mm × 16 mm × 3 mm using a low-speed saw (Isomet Low Speed Saw, Buehler, Lake Bluff, Illinois, USA) with a diamond blade. After cutting, the green blocks were polished with 600-grit silicon carbide grinding paper (CarbiMet™ 2, Buehler, Lake Bluff, Illinois, USA) to remove machining groves. The polished green blocks were then sintered at 1450 °C for 2 h in a box furnace (Lindberg/Blue M, Thermo Scientific Corp., Waltham, MA, USA) with a heating/cooling rate of 10 °C/min. During sintering at 1350–1450 °C, the Y-TZP material underwent a volumetric shrinkage of approximately 24%.
Group ZGI were the specimens of monolithic Y-TZP with glass infiltration (GI) on cementation surfaces. The polished Y-TZP green blocks were pre-sintered at 1350 °C for 1 h and cooled to room temperature at a heating/cooling rate of 10 °C/min to produce templates for glass infiltration. A uniform, powdered glass slurry was coated on cementation surfaces of the pre-sintered Y-TZP specimens and then dried in air. The glass consisted of 65.5 wt% SiO 2 , 11.7 wt% Al 2 O 3 , 10.0 wt% K 2 O, 7.3 wt% Na 2 O, 3.0 wt% CaO and 2.5 wt% Tb 4 O 7 . Glass infiltration and densification of the coated specimens were carried out simultaneously by heat treatment at 1450 °C for 2 h in the box furnace with a heating/cooling rate of 14 °C/min.
Group ZGI2 were the specimens of monolithic Y-TZP with glass infiltration on both cementation and occlusal surfaces using the same method for Group ZGI.
Group ZAC were the specimens of monolithic Y-TZP with alumina coating on cementation surfaces. This coating was applied according to the technique described by Kocjan et al. . The sintered zirconia specimens were immersed, with the cementation surface up, into deionized water at 90 °C for 30 s. Then, AlN powders of particle size of approximately 1.2 μm (Grade C, H.C. Starck, Berlin, Germany) were added to make a diluted solution with 3 wt% AlN, in which the hydrolysis reaction occurred for 7 min. The specimens were then removed from the solution, rinsed with deionized water, dried in air and heat-treated at 900 °C to convert the precipitated aluminum hydroxide (AlOOH) to transient δ-Al 2 O 3 .
Group ZAC2 were the specimens of monolithic Y-TZP with alumina coating on cementation surfaces and glass infiltration on occlusal surfaces using the same glass infiltration and alumina coating described above.
Plate-shaped specimens from groups Z, ZGI, ZGI2, ZAC and ZAC2 were made to a final dimension of 12 mm × 12 mm × 1.5 mm ( n = 10). Additional plates with the same lateral dimension of 12 mm × 12 mm but 1.0 and 2.0 mm thicknesses were also made for groups Z and ZGI ( n = 10).
Group PVZ were the specimens of monolithic zirconia veneered with porcelain. 18 plate-shaped specimens of monolithic zirconia of 12 mm × 12 mm × 0.5 mm were fabricated by a professional dental laboratory (Marotta Dental Studio, Farmingdale, New York, USA). Then, they were veneered with porcelain (VM9, Vita Zahnfabrik, Bad Sackingen, Germany) of 0.5, 1 and 1.5 mm thicknesses. Thus, three porcelain/zirconia bilayer groups with three thicknesses of 1, 1.5 or 2 mm ( n = 6) were obtained.
Group LiDi were the CAD/CAM-milled lithium disilicate glass–ceramic specimens. 18 plate-shaped specimens of 12 mm × 12 mm were divided into three groups ( n = 6) according to their final thicknesses of 1, 1.5 and 2 mm.
Finally, bar-shaped specimens of 3 mm × 4 mm × 25 mm from groups Z, ZGI, ZAC and LiDi were fabricated ( n = 10).
2.1.2
Fabrication of ceramic/cement/composite multilayer structures
For the Hertzian indentation test, ceramic specimens were cemented onto composite blocks of 12 mm × 12 mm × 4 mm (Z100, 3 M/ESPE Dental Products, St. Paul, Minnesota, USA). Prior to bonding, these blocks were stored in deionized water for 30 days to stabilize the water sorption-induced dimension change . The bonding surfaces of the composite blocks were roughened with 600-grit silicon carbide abrasive papers, then silane-treated (Multlink Automix, Ivoclar Vivadent AG, Schaan, Liechtenstein) and finally coated with a layer of cement before placing the ceramic specimens under a constant load of 750 g. The average cement thickness was controlled to be approximately 0.07 mm for all ceramic systems.
Specimens from groups Z, ZAC, ZAC2 and PVZ were sonically cleaned in 70% ethanol and air-dried prior to cementation. Once removing the excess cement, the cement was light cured (UltraLume 5LED, Ultradent Products, South Jordan, Utah, USA) for 40 s per surface. After curing, specimens were kept in deionized water at 37 °C for 7 days for cement hydration .
Specimens from groups ZGI and ZGI2, and group LiDi were etched with 4% hydrofluoric acid (Porcelain Etchant, Bisco Inc., Schaumburg, Illinois, USA) for 5 min and 20 s , respectively. Then, they were water-rinsed for 15 s and air-dried before the silane treatment and cement application as described above.
2.2
Mechanical testing
2.2.1
Hertzian indentation test
Fig. 1 is a schematic diagram of the Hertzian indentation test, which was designed to simulate a ceramic restoration (ceramic plate) cemented to a compliant tooth dentin support (resin-based composite) under occlusal loading. Each specimen was loaded on a universal testing machine (Instron 5566, Norwood, MA). A spherical tungsten carbide (WC) indenter of 3.18 mm radius was used to deliver the applied load. Each specimen was loaded monotonically at a 1 mm/min rate to avoid slow crack growth. Continuous data points were recorded during the test for load and extension at a time interval of 0.02 s until failure . Failure was defined as an initiation of cementation radial cracking accompanied by a small load drop from the force–displacement curve . All zirconia plates were cut by hand and underwent sintering-induced volume reductions of approximately 24%, inevitably causing some small variations in thickness amongst specimens. Additionally, the critical load is proportional to the square of a plate thickness . Thus, it was necessary to normalize the thickness to a nominal thickness for each specimen group ( i.e. 1.0, 1.5 or 2.0 mm) using the following equation:
P C = t nominal 2 × P measured t actual 2
where P C is the critical indentation flexural load (N), P measured is the measured load (N), t nominal is the nominal specimen thickness (mm) and t actual is the actual specimen thickness (mm).
2.2.2
Four-point flexural strength test
Groups Z, ZGI, ZAC and LiDi were also subject to four-point bending. For groups ZGI and ZAC, the glass-infiltrated and alumina-coated surfaces were loaded in tension. The loading rate was 1 mm/min and the fracture load was measured. The flexural strength, σ , was calculated using the following equation :
σ = 3 F L 4 w b 2
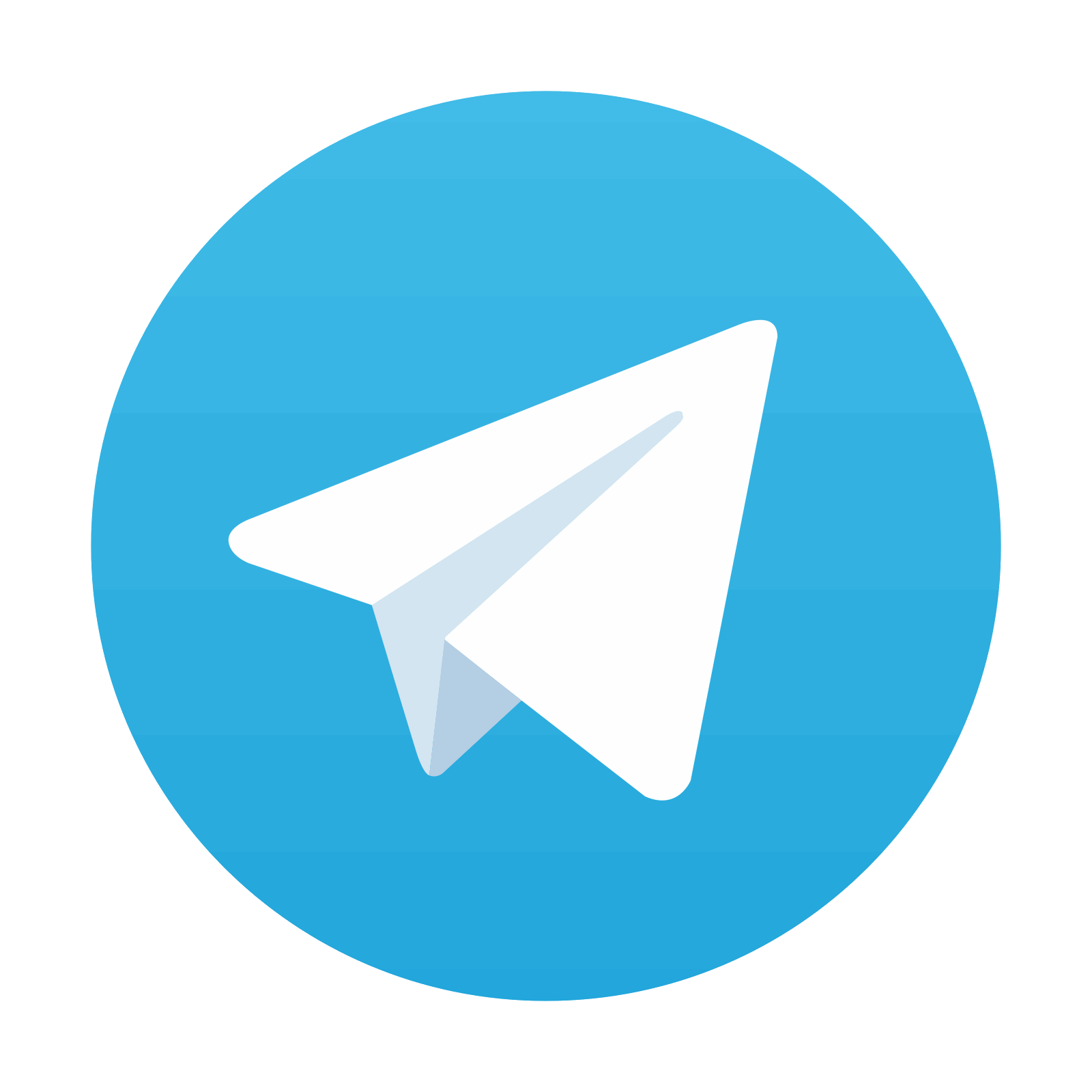
Stay updated, free dental videos. Join our Telegram channel

VIDEdental - Online dental courses
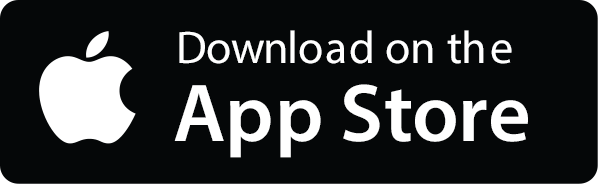
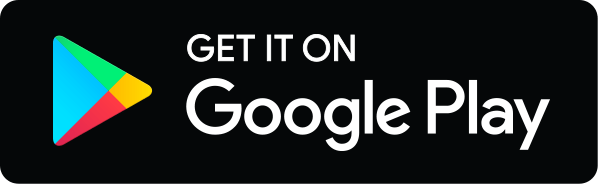