Highlights
- •
The composite showed enhanced cell proliferation rate when compared to Y-TZP.
- •
Addition of 12.5% of titania to Y-TZP resulted in formation of more mineralization nodules compared to the control.
- •
The composite showed no perceptible color differences when compared to Y-TZP.
- •
3Y-TZP/TiO 2 showed estimated failure probability 4.7% after 5 million cycles at a stress level of 50 MPa.
Abstract
Objective
The aims of this study were: 1) to evaluate the effect of sintering temperature on microstructure, density and flexural strength of a 3Y-TZP/TiO 2 composite containing 12.5 wt% of TiO 2 compared to 3Y-TZP specimens (control); 2) to compare 3Y-TZP with the experimental 3Y-TZP/TiO 2 composite, both sintered at 1400 °C, with respect to the following parameters: optical properties, characteristic strength, Weibull modulus, fatigue behavior, induction of osteoblasts proliferation and differentiation (mineralization nodules formation).
Methods
The 3Y-TZP and 3Y-TZP/TiO 2 powders were uniaxially pressed and sintered at 1200 °C, 1300 °C, 1400 °C or 1500 °C for one hour in a furnace. The microstructural analysis consisted of X-ray diffraction and scanning electron microscopy. The density was measured by the Archimedes’ principle and the flexural strength was obtained by the biaxial flexure test. The optical properties were measured using a spectrophotometer operating in the visible light wavelength range. The step-stress accelerated life testing was performed by the pneumatic mechanical cycler and the biological behavior achieved by using osteoblast-like cells (Osteo-1 cell line).
Results
Tetragonal zirconia was identified in all groups and cubic zirconia was identified only at 3Y-TZP group. The addition of TiO 2 decreased the values of density and flexural strength of the composite 3Y-TZP/TiO 2 in relation to 3Y-TZP regardless of the sintering temperature. The color difference between the two materials was not significant regarding L*a*b* parameters. The composite showed higher probability of failure, and induced higher proliferation and differentiation than control.
Significance
The composite developed have good aesthetic and biologics properties. However, its microstructure and mechanical properties need to be improved for future dental implant applications.
1
Introduction
The use of yttria-stabilized tetragonal zirconia polycrystal (Y-TZP) in dentistry has increased considerably in recent years. Properties, such as the whitish coloration, excellent biocompatibility and good mechanical properties, allow this ceramic to be applied in the fields of prosthodontics, orthodontics and implantology. Another factor that explains the great popularity of this material is the use of CAD-CAM technology, which facilitates the production of the zirconia restorations [ ].
Among all dental ceramics, 3Y-TZP (3 mol% of yttria) is the only one that possesses the toughening mechanism of displacive transformation. This phenomenon occurs when stresses are concentrated around existing defects in the material, triggering the transformation of the tetragonal grains into monoclinic grains. This transformation results in a volumetric expansion and also in many inter-related phenomena associated with the transformation cone formed at the crack tip [ ], that allowing 3Y-TZP to have a great ability to resist crack propagation and to exhibit higher fracture toughness compared to the veneering ceramics [ ].
In implantology, the gold standard material for rehabilitation is titanium (commercially pure or titanium alloy). High mechanical properties and good biocompatibility explain the success of this material in clinical studies with long follow-up periods [ ]. However, the grayish coloration of titanium is a major disadvantage in clinical rehabilitations with high esthetic demand. This situation is highly critical in the anterior dentition for patients with thin gingival biotype and with a high smile, because in these cases, blackened halos may appear near the perimplant regions. One option in these cases would be the use of 3Y-TZP implants, as their whitish shade may lead to better aesthetic results [ ].
On the other hand, 3Y-TZP is considered a relatively bioinert biomaterial [ ], which may impair osseointegration and increase the failure rate of the implant. To solve this problem, different ceramic composites were developed by adding to the matrix of the 3Y-TZP bioactive ceramics, such as calcium phosphates, bioactive glasses and titania [ ]. The objective of these additions is to develop a ceramic composite that is more bioactive than 3Y-TZP and that will lead to a faster and more reliable osseointegration process.
The highly bioactive behavior of titania or titanium dioxide (TiO 2 ) is associated with the formation of Ti−OH groups on its surface. These groups induce the adhesion of proteins, which in turn facilitate cellular adhesion and are also related to the nucleation of calcium phosphates when immersed into a solution of ionic composition similar to blood plasma (SBF) [ , , ]. In vitro studies associated titania with improved cell adhesion, spreading, proliferation and differentiation [ ]. In addition, animal study showed improved bone formation around implants with the presence of titania nanotubes in comparison to implants without this superficial alteration [ ].
The literature shows two different methodologies for obtaining 3Y-TZP/TiO 2 composites. One of them involves synthesis by means of the sol–gel route, using zirconium oxychloride, titanium chloride and yttrium chloride [ , ]. An alternative route involves mixing commercial powders of Y-TZP and titania in a ball mill [ ]. The sol-gel methodology results in more homogeneous materials; however it has several steps and consequently is very time-consuming. On the other hand, mixing commercial powders is faster, but generally results in less homogeneous powders. Additional advantage of commercial Y-TZP powders is that they have nanometric dimensions, contain small amounts of alumina (0.5 wt%) reducing low temperature degradation of 3Y-TZP and mainly exhibits suitable sintering properties.
Many studies in the literature have shown the great potential of 3Y-TZP/TiO 2 composite to be used as a biomaterial. This type of composite favors cell growth when compared to pure 3Y-TZP and has bioactivity, as it allows the formation of calcium phosphate on its surface. However, there is no consensus in the literature regarding which titania content should be added to 3Y-TZP powders and what is the most appropriate sintering temperature to obtain the maximum density of the composite [ , ]. In addition, 3Y-TZP/TiO 2 composites have not been characterized yet in terms of optical properties, fatigue behavior, and biological abilities, such as cell proliferation and differentiation.
The development of a novel ceramic composite for dental implant application with high osseointegration capacity requires a broad characterization, involving analysis of the microstructure, mechanical, optical and biological properties. Therefore, the general objective of the present work was to develop the 3Y-TZP/TiO 2 composite for dental implant application. The specific objectives were: 1) to evaluate the effect of the sintering temperature (1200 °C, 1300 °C, 1400 °C and 1500 °C) on the microstructure, density, and flexural strength of 3Y-TZP specimens (control) compared to a 3Y-TZP/TiO 2 composite containing 12.5 wt% of TiO 2 ; 2) to compare the control (3Y-TZP) with a Y-TZP/TiO 2 composite (12.5 wt%), both sintered at 1400 °C, with respect to the following parameters: optical properties, characteristic strength (σ o ), Weibull modulus (m), fatigue behavior (probability of failure estimated under fatigue at stresses of either 50 or 100 MPa, after missions of 1, 3 or 5 million cycles), induction of osteoblast proliferation and differentiation (mineralization nodules formation). The following hypotheses were tested: a) the sintering temperature would affect the properties of both the 3Y-TZP and the 3Y-TZP/TiO 2 composite and 2) the control and the composite would have similar behavior with respect to all parameters tested.
2
Materials and methods
2.1
Preparation of Y-TZP/TiO 2 composite
The starting materials chosen for this work were the commercial powders of tetragonal zirconia stabilized with 3 mol% yttria, Y-TZP (TZ-3YB-E, Tosoh, Japan) and TiO 2 (Sigma-Aldrich, US). The mixture of 3Y-TZP (87.5% by weight) and TiO 2 (12.5% by weight) was performed in a polymer flask with alumina balls and isopropyl alcohol using a ball mill for 10 h and then dried at 150 °C for six hours to evaporate the isopropyl alcohol. At the end, two compositions ( Table 1 ) were studied: the 3Y-TZP and the 3Y-TZP/TiO 2 composite (T12.5).
Groups | Composition (wt%) | |
---|---|---|
3Y-TZP (Tosoh, Japan) (3 mol% of yttria) | TiO 2 (Sigma Aldrich, US) | |
3Y-TZP | 100.0 | 0 |
T12.5 | 87.5 | 12.5 |
2.2
Processing of specimens
The 3Y-TZP and T12.5 powders were uniaxially pressed (50 MPa) into metal matrices and sintered at 1200 °C, 1300 °C, 1400 °C or 1500 °C for one hour in a furnace (Zircar, US). The specimens (n = 5 for each group and n total = 40) had their surface rectified in a semiautomatic polishing machine (Ecomet II, Buehler, US) with a diamond disk (Dia-Grid Diamond, 120 Grit Resin Bond, 12, Allied, US). The choice of a rough surface finish is related to an approximation with the surface applied to commercial 3Y-TZP implants, which are usually milled. The final dimensions of the specimens were approximately 12 mm in diameter and 1 mm in thickness for the mechanical testing and approximately 8 mm in diameter and 1 mm in thickness for the biological analyzes.
2.3
Microstructural analysis
The microstructural analysis consisted of X-ray diffraction (XRD, Rigaku, DMAX 2000, Japan) using CuK α 1 radiation (step size of 0.02 degree and acquisition time of 5 s) and scanning electron microscopy (SEM, Quanta 650 FEG, FEI, US). The specimens were polished and subjected to thermal etching (50 °C below the sintering temperature for 30 min) in a furnace (Zircar, US) to reveal inter-granular boundaries. Grain size of the 3Y-TZP and T12.5 was estimated by the linear intercept method with SEM images. The chemical mapping was obtained by dispersive energy spectroscopy (EDS, Quanta 650 FEG, FEI, US).
2.4
Density and flexural strength
The final density after sintering was determined using the Archimedes’ principle, with water as the immersion liquid. Theoretical density was calculated using the Rietveld refinement. For the biaxial flexural strength test, the specimens were fractured using a piston on three balls device in a universal testing machine (EMIC DL 200, Brazil). During the test, the specimens were immersed in water at 37 °C. For each group, five specimens were tested at a loading rate of 0.5 mm/min. The flexural strength was calculated according to Eq. (1) (ISO 6872/2008) [ ]:
σf=-0,2387FX-Yb2
where F is the fracture load, b is the specimen thickness and X and Y were determined by Eqs. (2) and (3) .
X=l+v.lnBC2+l-v2.BC2
Y=l+v1+lnAC2+1-v.AC2
where <SPAN role=presentation tabIndex=0 id=MathJax-Element-4-Frame class=MathJax style="POSITION: relative" data-mathml='v’>?v
v
is the Poisson’s ratio (0.31 for the 3Y-TZP group and 0.32 for the T12.5 group), A is the radius of the support circle (4 mm), B is the radius of the tip of the piston (0.85 mm) and C is the radius of the specimen (∼6 mm).
2.5
Optical properties and Weibull analysis
The optical properties were measured using CM 3700d spectrophotometer (Konica Minolta, Japan) operating in the wavelength ( λ ) range of visible light (360–740 nm, with 10 nm interval). The following parameters were standardized: observer function at 2°, illuminant D65 (daylight illuminant) and the specular component excluded were used. The specimens were placed on a white background in reflectance mode and a coupling agent was used to prevent light scattering. The color difference between the 3Y-TZP and the 3Y-TZP/TiO 2 composite was calculated using the CIEDE2000 (ΔE 00 ), using Eq. (4) [ ]:
ΔE00=ΔL’KLSL2+ΔC’KCSC2+ΔH’KHSH2+RTΔC’KCSCΔH’KHSH12
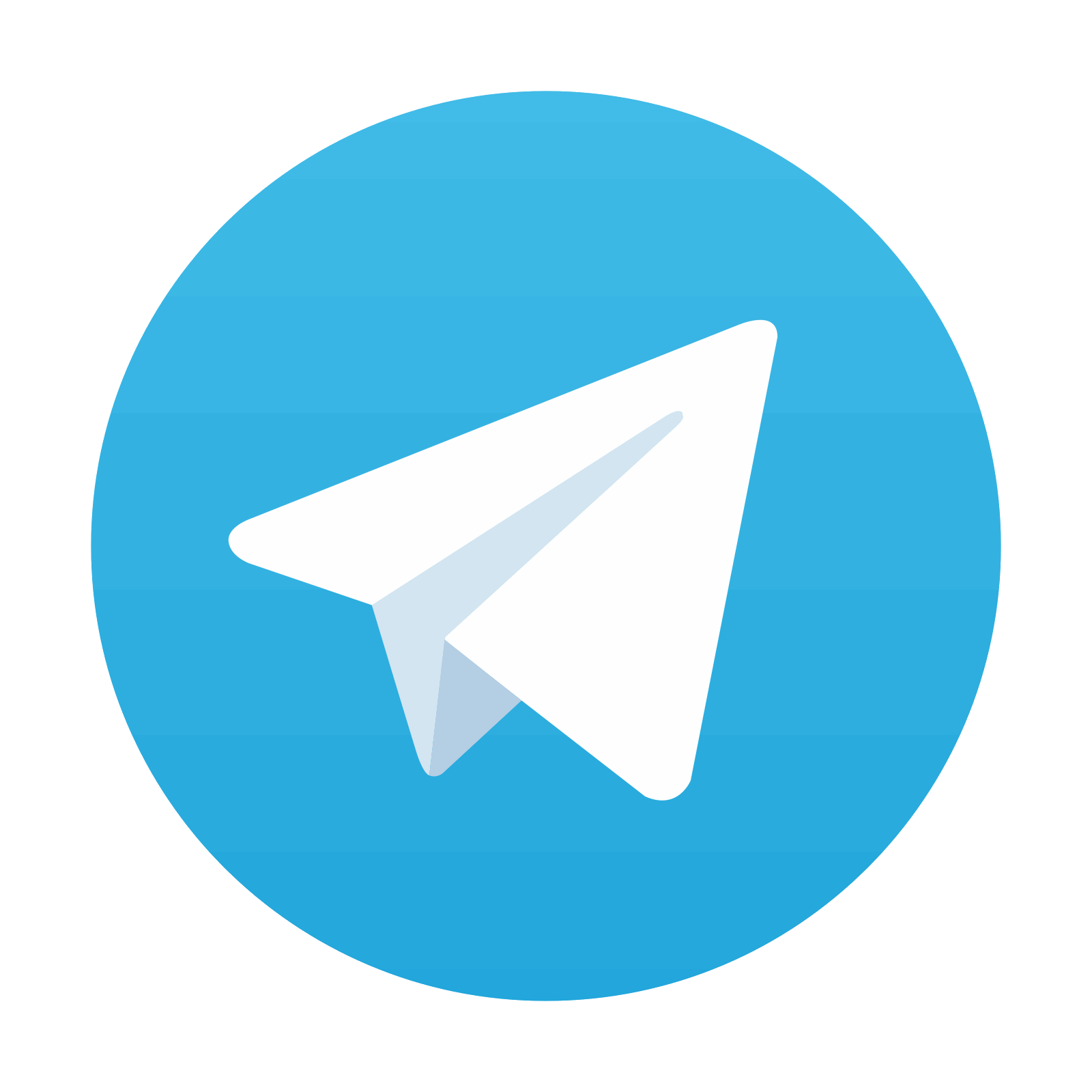
Stay updated, free dental videos. Join our Telegram channel

VIDEdental - Online dental courses
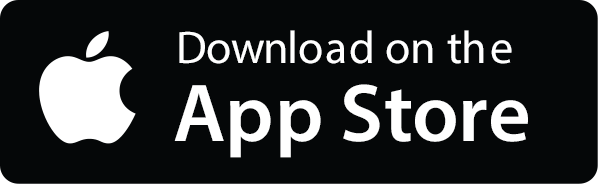
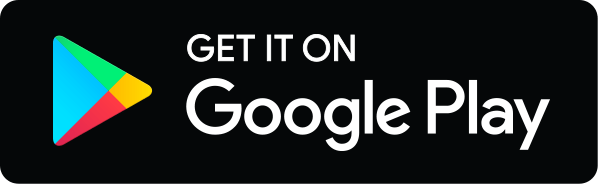
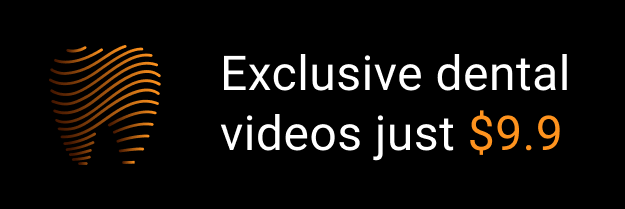