Introduction
Mini-implants are used to improve orthodontic anchorage, but optimal composition and surface characteristics have yet to be determined. We investigated the behavior of osteoblast-like cells on grade 4 commercially pure titanium and grade 5 titanium alloy with different surface treatments for mini-implants.
Methods
MC3T3 cells were plated on machined, acid-etched, or acid-etched grade 4 titanium enriched with calcium phosphate, or machined, anodized, or anodized and calcium phosphate-enriched grade 5 titanium disks. Surface and cell morphologies were assessed by scanning electron microscopy. Cell viability was measured by chemiluminescence, cytoskeletal organization was investigated by immunofluorescence, and real-time polymerase chain reaction for osteoblast-specific genes was performed to measure cell differentiation.
Results
Flattened shapes and strong stress fibers were observed on the machined surfaces; cells on the rough surfaces had a spindle shape, with lower cytoskeletal polarization. Cell proliferation was highest on smooth grade 4 titanium surfaces, whereas cells quickly reached a plateau on rough grade 4 titanium; no difference was observed after 72 hours in the grade 5 titanium groups. Calcium phosphate enrichment on grade 4 titanium significantly increased the messenger RNA levels for alkaline phosphatase and osteocalcin. Osteoblastic markers were higher on the grade 5 titanium machined surfaces than on the rough surfaces, and comparable with acid-etched grade 4 titanium.
Conclusions
Although the grade 4 titanium enriched with calcium phosphate had the highest level of differentiation in vitro, the grade 5 titanium machined surfaces supported cell proliferation and matrix synthesis, and induced high expression of early differentiation markers. Increased mechanical resistance of grade 5 titanium makes it a potential candidate for orthodontic mini-implants.
Mini-implants are provisional endosseous devices used to provide anchorage for orthodontic treatment. Commercially pure grade 4 titanium is widely used as an implantable material because of its high biocompatibility and lack of inflammatory response. Mini-implants, however, must be small to avoid the need of pilot drilling and cumbersome surgery, and to be easier for the patient to tolerate. The small size of mini-implants requires mechanical characteristics that must be considered when designing a mini-implant. To this purpose, stronger grade 5 titanium alloys containing aluminum and vanadium have been proposed, thus enabling the creation of smaller, self-drilling mini-implants. Furthermore, surface treatment can affect the proliferation and differentiation of bone cells, and osteoblastic cells proliferate more rapidly on smoother surfaces, although this is usually associated with a lower level of differentiation, and rough surfaces promote the expression of higher levels of osteoblastic markers, both in cell lines and primary cells. In-vivo experiments have shown that implant roughness promotes a higher bone-to-implant contact surface, thus providing greater removal torque. Several surface treatments have been proposed to improve the expression of differentiation markers and promote implant integration in bone. These include sandblasting and acid etching, which rely on the use of grit agents and acids to increase surface roughness, or electrochemical treatments that induce the formation of oxide layers of desired thickness and composition. Moreover, enrichment protocols have been proposed to deposit calcium phosphate crystals on the surface and promote their integration with the surrounding mineralized tissues. Recently, hydrophilic surfaces have been introduced to optimize cell-material interactions. Since most of the current, vast literature is focused on dental implants, the optimal surface treatment for mini-implants is still controversial. Although the purpose of a tooth-supporting implant is to reach the highest degree of osseointegration, it is not clear what extent of integration a provisional endosseous device must achieve, especially if this is conflicting with the ideal mechanical properties of the material. The aim of this study was to investigate, within the litmits of in-vitro settings, the performance of grade 5 titanium after anodization with or without calcium phosphate, by analyzing cell morphology, cytoskeletal organization, and cell growth and differentiation of murine osteoblastic cell line MC3T3, taking a new paradigm to evaluate the results and considering that the specific use of these materials will be different from dental implants. As a comparison, standard dental implant grade 4 machined or roughened titanium surfaces were considered.
Material and methods
Two groups of titanium samples were used in this study: commercially pure grade 4 titanium and grade 5 titanium alloy. They were provided by HDC (Saracedo, Vicenza, Italy) as sterile disks 10 mm in diameter and 1.5 mm in thickness. Each titanium test group comprised 1 smooth machined surface and 2 roughened surfaces. Rough grade 4 disks were obtained by either acid etching with hydrochloric acid or acid etching with the same procedure followed by calcium phosphate enrichment according to a proprietary procedure. The grade 5 titanium disks were treated with an electrochemical anodization procedure or the anodization procedure plus calcium phosphate enrichment. This alternative treatment for grade 5 titanium alloy was chosen because this material responds to etching differently from grade 4 titanium, and using the same etching protocol was therefore not possible.
The MC3T3 cell line is a murine osteoblastic cell line obtained from calvaria of C57/BL6 mice, broadly used as a model of osteoblasts for bone biology and biomaterial studies. It was obtained from the American Type Culture Collection (LGC Standards, Sesto S. Giovanni, Milan, Italy) and cultured in Dulbecco modified minimal essential medium (Euroclone, Milan, Italy) with 10% fetal bovine serum (Euroclone), 1% penicillin and streptomycin (Penstrep; Euroclone), and 1% glutamine (Euroclone) at 37°C in a modified atmosphere (5% carbon dioxide, 95% air). For the experiments, the cells were harvested at 70% to 90% confluence by using trypsin-EDTA 0.25% (Sigma Chemicals, St Louis, Mo) and seeded onto the titanium disks in 24-well plates (Euroclone) in culture medium enriched with 250 mmol per liter to promote the expression of the differentiation genes.
The cells were seeded on the titanium surfaces at a density of 1 × 10 4 cells per well in triplicate. The cells were analyzed after 24 hours of culture. They were fixed with a 2.5% glutaraldehyde solution in 0.1 mol per liter of sodium cacodylate buffer (pH, 7.3) for 30 minutes at 4°C. They were then dehydrated through a series of alcohols and then critical-point dried with liquid carbon dioxide (CPD 030; BalTec, Walluf, Germany). The specimens were then sputter coated with a gold-palladium layer (Plano GmbH, Wetzlar, Germany) by using a coating device (SCD 040; Balzers Union Ltd., Balzers, Liechtenstein). The samples were studied by using a scanning electron microscope at an accelerating voltage of 10 kV (DSM 950; Zeiss, Jena, Germany).
To evaluate cell viability, a bioluminescence assay was used (CellTiter Glo, Promega, Milan, Italy). This assay quantifies the adenosine triphosphate in cell lysate, thus inferring the number of metabolically active cells.
MC3T3 cells were seeded at a density of 3 × 10 4 cells per well in minimal essential medium 10% fetal bovine serum in 24-well plates containing the tested titanium disks of grade 4 and 5. Twenty-four or 48 hours after plating, 100 μL of lysis reagent was added to the wells, and the samples were treated according to the manufacturer’s recommendations. Samples were read with a Glomax 20/20 Luminometer (Promega) equipped with double injectors.
The cells were seeded at the concentration of 2 × 10 4 cells per well in complete medium on titanium disks in 24 well plates and were fixed after 24 hours with 4% paraformaldehyde for 10 minutes followed by 3 rinses with phosphate buffer solution (Sigma Chemicals). The cells were then permeabilized with 0.1% Triton-X100 (Sigma Chemicals) for 5 minutes followed by 3 rinses with phosphate buffer solution. The fixed slides were then blocked in 1% bovine serum albumin/phosphate buffer solution for 20 minutes at room temperature. Staining was then performed on each sample disk: the cells were incubated with a rabbit monoclonal anti-mouse vinculin antibody (V4139, Sigma Chemicals) for 60 minutes at room temperature, followed by 3 washes in sterile phosphate buffer solution. The cells were labeled with fluorescein isothiocyanate anti-rabbit IgG goat antibody (ab6717, Abcam, Cambridge, United Kingdom). Actin filaments were labeled with rhodamine phalloidin (FAK100; Chemicon, Temecula, Calif) for 1 hour in the dark followed by 3 rinses with phosphate buffer solution. Nuclear counterstaining was performed by incubation with 4′,6-diamidino-2-phenylindole (D1306, molecular probes; Invitrogen, San Giuliano, Milan, Italy) for 5 minutes followed by 3 rinses with phosphate buffer solution. The treated disks were then transferred to microscope slides and mounted under glass coverslips by using an antifade-mounting medium (P7481, molecular probes; Invitrogen) for photo-bleaching reduction. The samples were examined with a microscope (Eclipse 90i; Nikon, Tokyo, Japan) equipped for fluorescence analysis.
To count cells, MC3T3 cells were seeded on the titanium samples; after 24 hours, they were fixed and stained with 4′,6-diamidino-2-phenylindole as described above. Two independent and blinded operators (C.G. and M.P.), previously calibrated, counted the numbers of cells in a series of 20 fields per surface at 60 times magnification. The average number per field was then calculated.
To evaluate the gene expression specific to the ostegenic activity of osteoblasts, 1 × 10 5 MC3T3 cells were seeded in 24 well plates containing the titanium disks with minimal essential medium 10% fetal bovine serum supplemented with 250 μmol per liter of ascorbic acid (Sigma Chemicals). Seventy-two hours after plating, total cellular RNA was extracted using the TriZOL reagent (Invitrogen), and 2 μg of total RNA was reverse-transcribed to complementary DNA by using the high capacity complementary DNA reverse transcription kit (Applied Biosystems, Foster City, Calif). TaqMan quantitative reverse transcriptase-polymerase chain reaction was performed as previously described by using the following primer probe sets from Applied Biosystems: alkaline phosphatase (Mm00475834_m1); collagen type I (Mm00801666_g1); osteoprotegerin (Mm00435451_m1); osteocalcin (for 5′-GCTGCGCTCTGTCTCTCTGA-3′; rev 5′-TGCTTGGACATGAAGGCTTTG-3′; probe 5′-FAM-AAGCCCAGCGGCC-NFQ-3′); housekeeping gene, mouse ribosomal protein S2, and ChoB (for 5′-CCCAGGATGGCGACGAT-3′; rev 5′-CCGAATGCTGTAATGGCGTAT-3′; probe, 5′-FAMTCCAGAGCAGGATCC-NFQ-3′).
Statistical analysis
The data were analyzed by using Prism 4 (GraphPad, La Jolla, Calif). All values are reported as the means ± standard deviations of the 3 repeated experiments in triplicate. Analysis of variance (ANOVA) and the Brunner-Langer test were used to determine the statistical significance of the differences between the groups. P values less than 0.05 were considered significant.
Results
To observe the texture and the topography of the tested disks, we examined them with scanning electron microscopy. Both machined grades 4 and 5 disks exhibited relatively smooth surface patterns ( Fig 1 , A and D ). Nevertheless, at higher magnification, the grade 4 surfaces had many parallel grooves, also characterized by some shallow irregularities ( Fig 1 , A ), whereas the machined grade 5 titanium disks ( Fig 1 , D ) overall showed fewer irregularities.
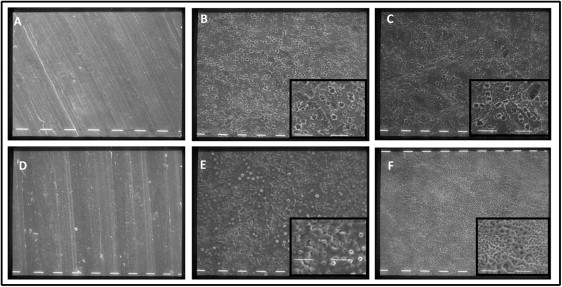
The acid-etched and acid-etched plus calcium phosphate grade 4 surfaces ( Fig 1 , B and C ) had a microrough topography. Micropits with jagged edges, the result of the acid-etching process, were visible on the surface. These had variable sizes (1-4 μm) and were distributed unevenly on the surface. Although the acid-etched, calcium phosphate treated disks showed similar topographic features as did the acid-etched surfaces, observation at a higher magnification showed fewer micropits with different shapes and sizes ( Fig 1 , C ).
Small, rounded formations were present on the grade 5 electrochemically treated samples; at higher magnification, these appeared as surface swellings with a central pore ( Fig 1 , E ). The disks prepared with electrochemical treatment and calcium phosphate enrichment had a finer texture, organized as a porous microstructured network, with irregular and, in some regions, larger pores ( Fig 1 , F ).
To better understand the complex cell and material interactions, the morphology of murine osteoblastic MC3T3 cells on the materials was investigated with scanning electron microscopy after 2 days of culture. Cells on the machined grade 4 titanium appeared flattened and projected multiple long and thin membrane filopodes ( Fig 2 , A ). On the acid-etched surfaces, the cells exhibited a polygonal morphology with well-formed cytoplasmic extensions ( Fig 2 , B and C ). They had a high degree of contact with the underlying surface, and the topographic features of the substrate were visible through the cell body at high magnification ( Fig 2 , B , inset). Cells on machined grade 5 disks had different morphologies: they were either polygonal with visible processes or flat and spread over the surface with fewer and blunter processes than those observed in the cells on the machined grade 4 titanium ( Fig 2 , D ). The cells on rough grade 5 titanium displayed a spread cell body, closely anchored to the surface but with fewer projections ( Fig 2 , E ).
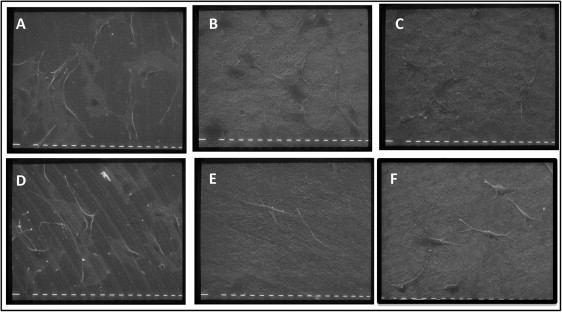
Cells in contact with both calcium phosphate enriched surfaces appeared less spread than in the other groups, showing an elongated, spindled, and bipolar morphology, and displayed large lamellipodia with which they took contact with the microroughness of the substrate ( Fig 2 , F ).
To visualize the cytoskeletal architecture and the distribution of vinculin, a cytoskeletal protein required for focal adhesion assembly, fluorescence microscopy was performed on the titanium samples after 24 hours of culture ( Fig 3 ).
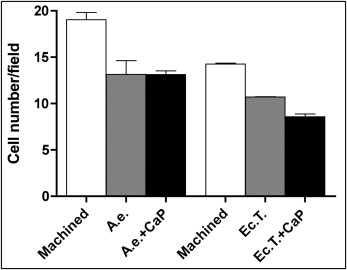
Higher cell densities were observed on the smooth grade 4 samples than on the rough grade 4 surfaces ( Fig 3 ). Cells in contact with both grades 4 and 5 machined surfaces possessed visible stress fibers, which are bundles of actin filaments oriented along the main cellular axis according to the vectors of intracellular force ( Fig 4 , G and J ). Focal adhesions were distributed at the cell pheriphery as bright spots, and colocalized with actin filaments ( Figs 4 , M and P , and 5 , A and B ).
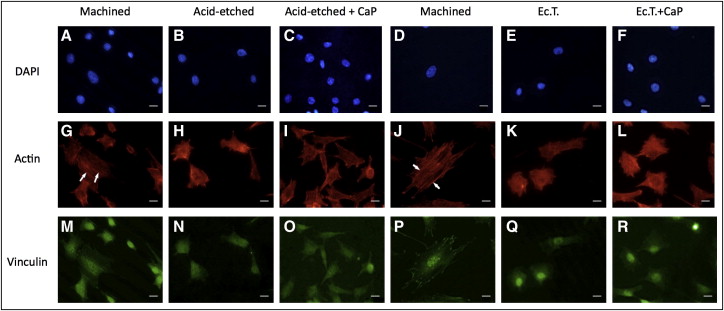
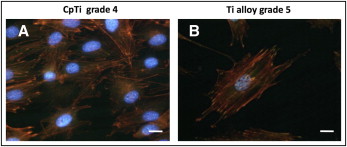
No relevant differences in cell morphology were observed among the rough surfaces: star-shaped cells were frequent, but fewer stress fibers were visible. On the grade 4 rough surfaces, the vinculin-associated fluorescence was weaker and appeared grainy and dispersed throughout the cell ( Fig 4 , N and O ), whereas vinculin in cells on the rough grade 5 surfaces was mainly localized in and around the nucleus ( Fig 4 , Q and R ). Calcium phosphate enrichment on the grade 5 surfaces induced the formation of short extroflections, in agreement with the observations from the scanning electron microscopy.
Osteoblast proliferation values on grades 4 and 5 titanium surfaces were measured at 24, 48, and 72 hours with a bioluminescence assay ( Fig 6 ). Cell numbers constantly increased on machined grade 4 titanium surfaces throughout the experiment ( Fig 6 , A ). Cells cultured on rough grade 4 surfaces, however, reached a growth plateau after 48 hours, and no further growth was observed after this time. At each time point tested, cell viability on rough grade 4 surfaces was significantly lower than on the machined titanium, and calcium phosphate enrichment did not significantly affect growth kinetics ( Fig 6 , A ).
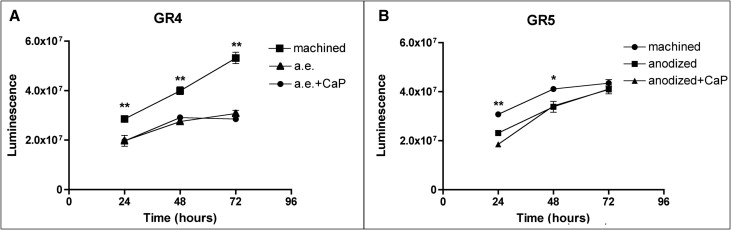
Differences in cell growth on grade 5 titanium disks were less visible ( Fig 6 , B ). Cell numbers on both rough surfaces were significantly lower than on the machined surfaces at 24 and 48 hours ( P <0.001 and P <0.05, respectively), but no difference was observed after 72 hours of culture.
Moreover, the machined surface of grade 4 titanium appeared to promote higher cell proliferation than did the machined grade 5 at all time points ( Fig 6 , B ; P <0.001).
We then investigated the expression of messenger RNA for collagen type 1, alkaline phosphatase, osteoprotegerin, and osteocalcin in MC3T3 cells, after the addition of ascorbic acid to the culture medium for 3 and 6 days ( Fig 7 ).
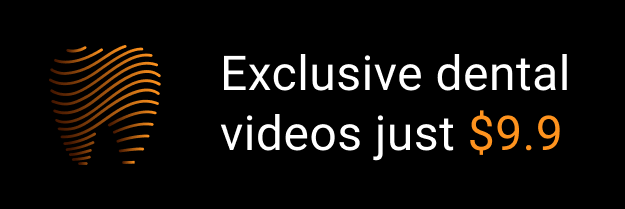