Introduction
This study aimed to investigate the effect of print orientation and ultraviolet (UV) light curing duration on the dimensional accuracy of a clear aligner design fabricated directly using 3-dimensional (3D) printing.
Methods
A master clear aligner design file was 3D printed on a stereolithography printer using 3 different build angles with respect to the build platform: parallel (Horizontal), perpendicular (Vertical), and 45° (45-Degree) (n = 10/group). The 45° orientation then was used to print aligners for 3 postprint processing treatment groups: 0 minutes of UV light and heat exposure (No Cure); 20 minutes of UV light exposure at 80 o C (20 Minute), and 40 minutes of UV light exposure at 80 o C (40 Minute) (n = 10/group). Each part was digitally scanned and superimposed with the input file for 3D deviation analysis. A generalized linear mixed model and post-hoc Tukey contrasts were applied for statistical analysis.
Results
Difficulties were encountered in optical scanning of 3D-printed aligners, resulting in the exclusion of some samples and the No Cure group from the analysis. The average positive and negative deviations were not statistically significantly different among the print orientations, and postprint processing conditions were analyzed and fell within limits of clinical acceptability (0.250 mm). Color deviation maps illustrated localized areas of dimensional deviation that may affect the clinical utility of the printed aligner design.
Conclusions
The print orientation and postprint curing duration have little effect on the overall accuracy of the 3D-printed aligner design under the conditions investigated. However, the potential effects of location-specific deviations on the clinical utility of 3D-printed aligners should be considered in future studies.
Highlights
- •
Optical scanning of 3-dimensionally printed clear aligners presents challenges.
- •
Print orientation did not significantly affect overall dimensional accuracy.
- •
Overall deviations do not represent location-specific deviations affecting clinical use.
The influx of digital technology and computer-aided design and computer-aided manufacturing into the orthodontic space enables increased use of positioner-like appliances for major tooth movement. Applying computer-aided design and computer-aided manufacturing technology, the workflow for clear aligner therapy traditionally uses a single intraoral impression or digital scan to generate multiple digital setups. These setups are then 3-dimensionally (3D) printed as a series of dental models onto which clear, plastic aligners can be manufactured through thermoforming.
Currently, the research has focused primarily on the accuracy of intraoral scanning, 3D-printed models, and fabrication of clear aligners on 3D-printed models using the traditional workflow. However, resins are emerging that may be suitable for direct fabrication of clear aligners through 3D printing, obviating the need for the 3D-printed intermediate models and thermoforming steps associated with the traditional workflow. If accurate, these resins could dramatically improve the efficiency of the process, enabling more rapid delivery of aligners to the patients and generating a higher yield for the practice while reducing waste and cost.
Although the accuracy of 3D-printed orthodontic models has been investigated in the literature, key differences exist in the geometry of models and aligners; specifically, the geometry of an aligner design file is more complex than a 3D-printed model. Each printed aligner design has a shell-like morphology, presenting an intaglio (inside) surface that contacts the teeth and a cameo (outside) surface that contacts the soft tissue and opposing dentition. The complexity of the aligner design must be realized for its potential clinical ramifications, as unwanted deviations in the dimensional accuracy of the printed aligner relative to the digitally designed aligner can result in potential clinical sequelae such as undesired tooth movement.
With the emergence of technologies presenting the potential to directly 3D print aligner designs, key questions remain regarding the impact of tunable printing parameters, such as build angle and postprint heat and ultraviolet (UV) light exposure duration, on the dimensional accuracy of appliances directly fabricated using 3D printing of clear materials marketed for long-term intraoral use. This study aimed to investigate the impact of (1) print orientation (part 1) and (2) postprint curing conditions (part 2) on the dimensional accuracy of a passive clear aligner design fabricated directly using a stereolithography (STL)-format 3D printer. The null hypotheses were that the orientation of the aligner during printing will not affect the dimensional accuracy of the 3D-printed aligner and that the duration of heat and UV light exposure will not affect the dimensional accuracy of the 3D-printed aligner.
Material and methods
A master maxillary passive clear aligner STL file of 0.500-mm thickness was designed using 3D modeling software (Meshmixer, version 3.2; Autodesk, San Rafael, Calif) and a surface scan of an ideal maxillary typodont as the reference anatomy ( Fig 1 ). The master maxillary clear aligner design file was prepared for printing in each of 3 orientations using print preparation software (PreForm Software, version 2.19.3; Formlabs, Somerville, Mass): (1) occlusal plane parallel to the print platform (Horizontal), (2) occlusal plane perpendicular to the print platform (Vertical), and (3) occlusal plane offset at a 45° angle to the platform (45-Degree). Support structures were generated automatically by the software for each orientation and edited as needed to avoid the inclusion of supports on essential areas of the aligners, such as the intaglio surface ( Fig 2 ). Thirty copies of the master clear aligner STL design file were printed in 10 separate print jobs on a Form 2 printer (Formlabs) using a clear, Class IIa biocompatible resin marketed for direct fabrication of long-term orthodontic appliances, including retainers and splints (Dental LT Clear Resin [FLDLCL01]; Formlabs). Each print job included 1 aligner at each of the investigated orientations. The aligners were randomly arranged on the build platform in each print job to vary the location of the part of a given orientation across print jobs. The aligners were processed after printing in accordance with the manufacturer’s instructions. Briefly, the aligners were removed from the build platform and rinsed in isopropyl alcohol (IPA; >96%) for 2 minutes in an ultrasonic bath. They then were transferred to a second IPA bath and sonicated for 3 minutes. A compressed air hose was used to accelerate the drying of the aligner surfaces. After at least 30 minutes of additional drying, the aligners then were placed in a heated curing unit (Form Cure; Formlabs) for 20 minutes at 80 ° C in accordance with the manufacturer’s instructions. Flush cutters (Formlabs) were used to remove the supports from each aligner.


After processing in accordance with the manufacturer’s instructions, each aligner was individually sprayed with Spotcheck SKD-S2 Aerosol Developer (Magnaflux, Glenview, Illinois) to facilitate scanning, and the spray coating was thinned with compressed air. The aligners were scanned with an intraoral scanner (iTero Element Flex; Align Technology, Inc, San Jose, Calif) to produce a digital representation of the surfaces of each 3D-printed aligner. The digital file for each printed aligner then was superimposed on the master STL file using an automated best-fit algorithm (Geomagic Control, version 2015.1.1; 3D Systems, Rock Hill, SC). Briefly, the automated best-fit alignment algorithm applied an initial gross alignment phase using a low number of comparison points between the surfaces of the 2 objects, followed by a fine adjustment phase to optimize the alignment using a higher number of comparison points. The comparison points in each phase were determined automatically by the software. A 0.250-mm tolerance was set in the software to analyze the differences between the surfaces of the master digital file and each superimposed aligner scan. Any points in the scanned file deviating more than 0.250-mm in the positive or negative directions were considered to be beyond the upper and lower bounds, respectively. Reports for each superimposition were generated, and included average positive deviations, average negative deviations, standard deviation, and percentage points out of bounds.
Thirty copies of the same master clear aligner design file from part 1 were printed at a 45° angle to the platform. Five print jobs of 6 identically orientated aligners each were completed on a Form 2 printer. The aligners were randomly arranged in each print job to vary the location of the parts on the build platform across prints. The aligners from each print job were randomly assigned to 3 groups, with each group receiving 2 aligners from each print job. The 3 resultant groups comprised a total of 10 aligners each. The groups were classified according to the duration of exposure to heat and UV light for postprint processing: (1) no heat or UV light applied (No Cure), (2) 20 minutes of UV light at 80 ° C (20 Minute); and (3) 40 minutes of UV light at 80 ° C (40 Minute). After the print was completed, the aligners were removed from the build platform and processed as in part 1, except for the postprint UV curing conditions. Specifically, the aligners were placed in the Form Cure unit for varying durations of UV light and heat exposure in accordance with the respective group conditions. Flush cutters were used to remove the supports from each aligner. Scanning and superimposition analysis was completed as described in part 1.
Statistical analysis
A generalized linear mixed model and post-hoc Tukey contrasts were applied to compare the response variables associated with each orientation and duration. The level of significance for all analyses was set at P <0.05.
Results
Errors were encountered in the scanning of some aligners during the image processing and compilation stage, which resulted in segments of the arch being omitted from the digital representation. Rescanning was not able to overcome the issue in these instances, and incomplete scan files were excluded from the study. The number of successfully scanned aligners was 8, 10, and 3 for the 45-Degree, Horizontal, and Vertical groups, respectively. General linear mixed models indicated no statistically significant differences in the average positive deviations, average negative deviations, and percent of points out of bounds among the groups investigated ( P >0.05) ( Table I ). The average positive and negative deviations for all 3 treatment groups fell within the reported limits of clinical acceptability (0.250 mm). , A general linear mixed model indicated a statistically significant difference in the average standard deviation of all 3 orientations ( P <0.05). Post-hoc Tukey contrasts yielded that the standard deviation observed with the 45-Degree group was significantly lower than the Horizontal ( P <0.05) and Vertical ( P <0.005) groups, which were not significantly different from one another. Although the standard deviation was significantly lower for the 45-Degree group, the standard deviations for all treatment groups were below reported limits of clinical acceptability (0.250 mm).
Deviation | 45° (n = 8) | Horizontal (n = 10) | Vertical (n = 3) | GLMM | Tukey post-hoc | ||
---|---|---|---|---|---|---|---|
P | 45 to H | 45 to V | H to V | ||||
Positive deviation, mm | 0.135 ± 0.009 | 0.139 ± 0.014 | 0.153 ± 0.014 | 0.143 | |||
Negative deviation, mm | −0.079 ± 0.014 | −0.093 ± 0.018 | −0.102 ± 0.020 | 0.102 | |||
Standard deviation, mm | 0.133 ± 0.012 | 0.152 ± 0.018 | 0.167 ± 0.011 | 0.008∗∗ | 0.020∗ | 0.003∗∗ | 0.322 |
Out of bounds, % | 8.61 ± 2.31 | 11.7 ± 3.27 | 12.8 ± 3.77 | 0.063 |
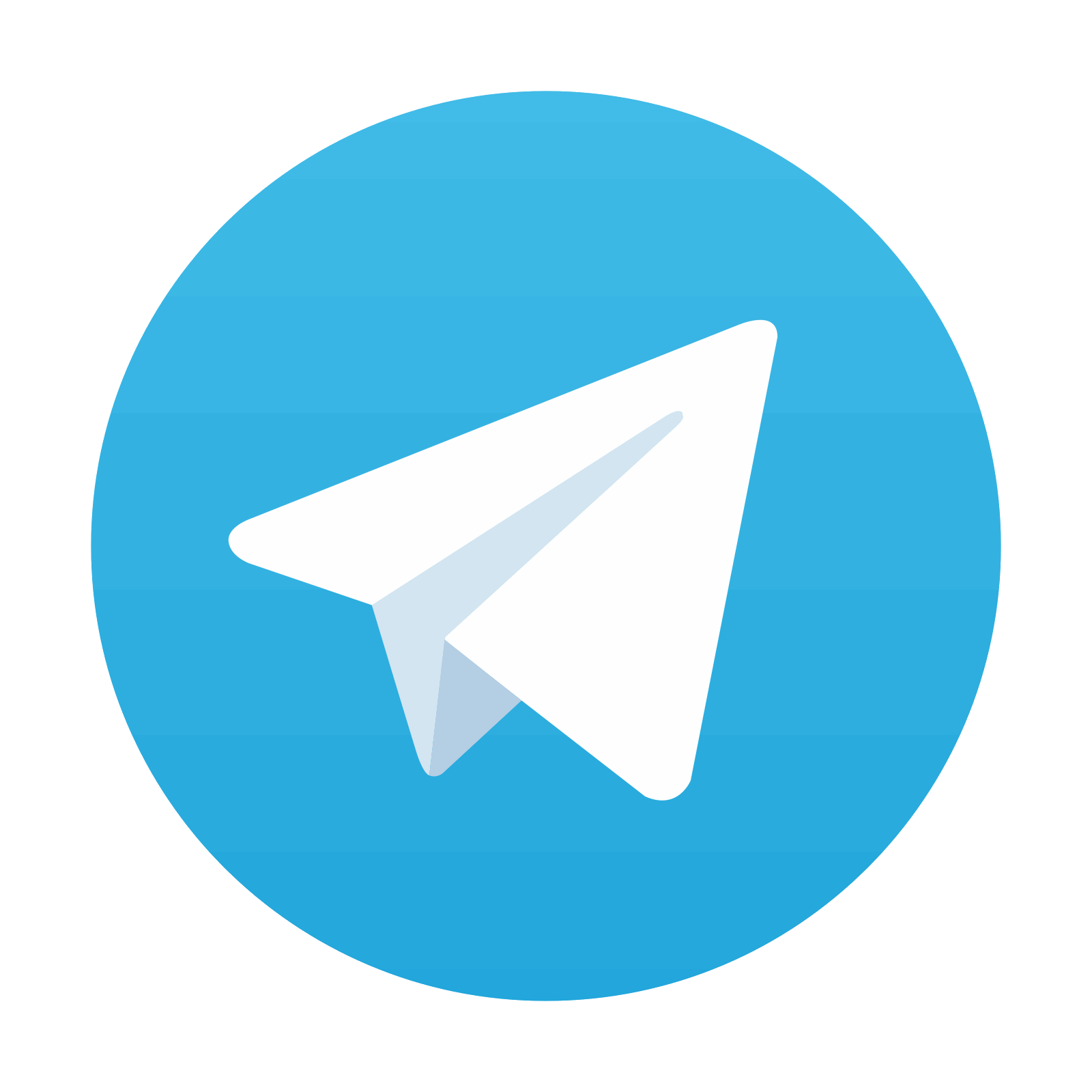
Stay updated, free dental videos. Join our Telegram channel

VIDEdental - Online dental courses
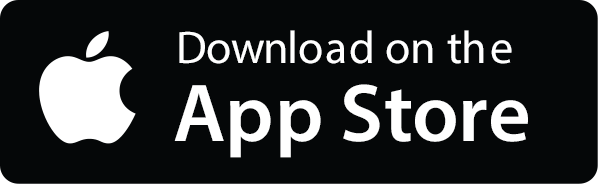
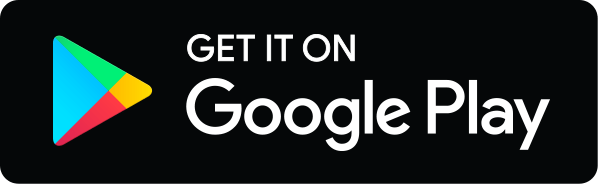
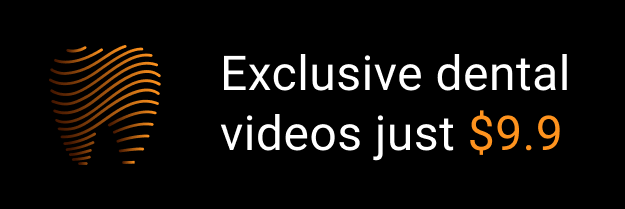