Introduction
Periodontal ligament cells (PDLC) respond to the application of mechanical forces by releasing various molecules that participate in bone remodeling. Whether these cellular reactions take place at the same rate in adolescent and adult patients is not known. In this study, we aimed to evaluate differences in genetic expression, if any, between the release of various inflammatory mediators from PDLC in adolescent and adult patients before and after the application of orthodontic forces.
Methods
Forty subjects with bimaxillary dentoalveolar protrusion requiring extraction of first premolars for orthodontic treatment were selected and divided into 2 groups. Group A included 20 adolescents (aged 12-20 years), and group B included 20 adults (aged 35-50 years). Then, 35-50 g of force were applied to the maxillary first premolars, and teeth were extracted at different periods: pretreatment (control group), 7 days, 14 days, and 28 days (experimental group). The periodontal ligament was scraped from the middle third of the root, and the beta-galactosidase assay was performed in the control group. RNA extraction, DNase treatment, quantitative polymerase chain reaction, and complementary DNA synthesis were performed in the experimental group.
Results
Adult PDLC exhibited senescent changes through increased beta-galactosidase activity. The increase in the inflammatory response and bone resorption in adult patients was evident by increased prostaglandin E2, IL1B , and acid phosphatase mRNA expression levels. Controlled bone formation response by adolescent PDLC was evident from increased ALP and BGLAP mRNA levels and a balanced receptor activator of nuclear factor kappa-Β ligand/ OPG ratio.
Conclusions
The study could identify the reasons behind the differential response of adolescent and adult PDLC to orthodontic mechanics.
Highlights
- •
The response of adolescent and adult periodontal ligament cells were evaluated.
- •
Cells from adult patients exhibited senescent changes.
- •
Adult cells expressed an increase in prostaglandin E2, IL1B , and acid phosphatase mRNA.
- •
Adolescent cells showed increased ALP and a balanced RANKL / OPG ratio.
- •
The observed increase in levels of BGLAP mRNA at day 28 in adolescent cells.
Mechanical loading with orthodontic force application initiates periodontal ligament (PDL) remodeling and differentiation facilitating tooth movement. The most abundant cells in the PDL are fibroblasts, along with bone cells and odontoblasts, which are highly responsive to these mechanical stresses. Many researchers have tried to elucidate the molecular mechanisms that translate these forces into cellular responses and has been successful to a great extent. It is clear from the existing literature that PDL fibroblasts are capable of osteoblastic differentiation in response to several stimuli, including mechanical cell deformation, and because of this ability, they are important for the maintenance of tissue homeostasis, repair, and remodeling. ,
Cells continually experience stress and damage from exogenous and endogenous sources, and their responses range from complete recovery to cell death. Proliferating cells can initiate an additional response by adopting a state of permanent cell-cycle arrest that is termed cellular senescence. Understanding the causes and consequences of cellular senescence has provided novel insights into how cells react to stress, especially genotoxic stress, and how this cellular response can affect complex organismal processes such as the development of cancer and aging. Although it is known that aging affects PDL homeostasis, the role of cellular senescence on the activation of signaling pathways in periodontal ligament cells (PDLCs) in response to mechanical stimulation has not been studied yet.
It is understood that the PDLCs release prostaglandins initially in response to mechanical force application, after which cytokines such as interleukins are released, facilitating signal transduction. The role PDLCs play in bone remodeling responses is portrayed through various studies demonstrating the release of enzymes (eg, acid phosphatase [ACP] and ALP ), bone remodeling cytokines (eg, RANKL and OPG ), as well as proosteoblastic proteins such as osteocalcin or bone γ-carboxyglutamic acid containing protein ( BGLAP ). The sequence of events portrayed above is reported in the healthy adolescent periodontium, but the information from adult patients regarding the same is lacking in the literature. What is not known is whether cellular reactions take place in the same rate in adolescent and adult patients as cellular renewal happens every 60 days as per the research on chick embryo by Weissman-Shomer and Fry. It is also reported that the Hayflick limit of cultured human fibroblasts is approximately 50 population doublings after which they undergo senescence (a loss of replicative capacity). The present study is aimed to find the difference in genetic expression through mRNA evaluation, if any, between the release of cytokines, enzymes as well as 1 protein from PDLC of adolescent and adult patients before and after subjecting to orthodontic mechanical forces.
Material and methods
Forty subjects with bimaxillary dentoalveolar protrusion who required the extraction of all first premolars for their orthodontic treatment and who were ready to give consent for using their extracted teeth for the study purpose were selected from among those who attended the outpatient clinic of Department of Orthodontics and Dentofacial Orthopaedics, Sri Sankara Dental College Varkala, Trivandrum, Kerala, India. Patients were excluded if they had any systemic diseases or had consumed drugs for any disease condition, had periodontally compromised and restored teeth, had teeth subjected to any sort of trauma or fractured, or any history of orthodontic treatment. Ethical approval of the study was obtained from the Institutional Ethical Committee of Sri Sankara Dental College vide reference number IEC/007/2015 on December 19, 2016. On the basis of chronological age, the subjects were divided into 2 groups of 20: group A, adolescents (range, 12-20 years; mean age, 16.4 ± 2.78 years), and group B, adults (range, 35-50 years; mean age, 40.9 ± 3.99 years). These groups were further divided into subgroups on a random basis (5 each; control, 7 days, 14 days, and 28 days) based on the timing of extraction of first premolars after orthodontic force application. A power analysis established by G∗Power software (version 3.1.3; Franz Faul University, Kiel, Germany), based on an equal ratio among groups and a group size of 20 subjects, would give 91% power to detect significant differences with a 1.2 effect size “f” and at the α error probability = 0.01 (critical F, 5.2922140; noncentrality parameter, λ = 28.800000). So, for the 2 groups, a total of 40 patients (20 males, 20 females) were included. All patients underwent routine periodontal evaluation with gingival index, plaque index, bleeding on probing, and probing pocket depth and found to be healthy and able to receive orthodontic forces. The same parameters were looked for at every visit (7 days, 14 days, and 28 days) to check the level of oral hygiene maintenance and were found to be satisfactory (data not shown). The aim and design of the study were explained to each patient before the start, and each agreed to participate by signing an institutional review board–approved informed consent.
The treatment in these patients was initiated with a 0.022 × 0.028-in slot Roth prescription straight wire appliance. Then, a 35-50 g of force (measured through Dontirx gauge) was applied to all maxillary first premolars using a custom-designed spring promoting tipping toward the buccal side ( Fig 1 ). The scheduled teeth extraction was carried out in an atraumatic manner at different periods, such as pretreatment (for the control group), 7 days, 14 days, and 28 days (experimental group) in both groups, followed by an immediate wash in Tris saline buffer until blood-free. The experimental side (pressure side on the labial aspect) was scored in the enamel for proper identification. The periodontal ligament was scraped from the middle third of the root of the extracted teeth, from both the control and experimental group specimens using curettage with a scalpel blade. Both experimental and control group specimens were stored in a frozen environment (−20°C) after periodontal tissue weighing and homogenization in the Eppendorf tubes containing RNA later solution, except for those for beta-galactosidase [GLb] assay.

The GLb assay is a sandwich enzyme immunoassay for in vitro quantitative measurement of GLb in human serum, plasma, tissue homogenates, cell lysates, and other biological fluids. The GLb assay has become 1 of the most commonly used markers of cell aging in vitro. The GLb Assay Kit (no. SEA196Hu; Cloud-Clone Corp, Wuhan, China) was obtained, which consists of a microplate precoated with an antibody specific to GLb. The control group and experimental group specimens from adolescent and adult subjects were rinsed in ice-cold phosphate-buffered saline to remove excess blood thoroughly and weighed before homogenization. Next, the tissues were minced to small pieces and homogenized them in freshly prepared lysis buffer (w:v = 1:20-1:50 [eg, 1 mL lysis buffer is added in 20-50 mg tissue sample]) with a glass homogenizer on ice. Then, the homogenates were centrifuged for 5 minutes at 10,000 × g, and the supernatant was collected to perform the assay immediately. Specimens were then added to the appropriate microplate wells with a biotin-conjugated antibody specific to GLb. Then, avidin conjugated to horseradish peroxidase is added to each microplate well and incubated. After 3,3′,5,5′-tetramethylbenzidine substrate solution is added, only those wells that contain GLb, biotin-conjugated antibody, and enzyme-conjugated avidin will exhibit a change in color. The enzyme-substrate reaction was terminated by the addition of a sulfuric acid solution, and the color change is measured spectrophotometrically at a wavelength of 450 nm ± 10 nm. The concentration of GLb in the samples is then determined by comparing the optical density of the samples with the standard curve.
The samples stored at −20°C were centrifuged at 1000 revolutions per minute (rpm) for 5 minutes, and the RNA later solution was removed, then the tissue was pressed gently in a sterile Whatman filter paper to remove the traces of solution before processing. The tissue was then powdered in liquid nitrogen using sterile mortar and pestle. To the powdered sample, 1 mL of Trizol Reagent (Takara Bio, Shiga, Japan) was added mixed by grinding, transferred to a 2 mL microcentrifuge tube, and incubated at room temperature for 15-20 minutes. It was then centrifuged at 10,000 rpm for 5 minutes, and the supernatant was transferred to a fresh sterile microcentrifuge tube (Eppendorf India Private Limited, Chennai, India). An equal volume of Chloroform: Isoamyl alcohol (24:1) was added, mixed gently, and incubated in ice for 2 minutes. After centrifuging the above at 12,000 rpm for 5 minutes, the supernatant was transferred to a fresh vial, which was then incubated in ice for 2 minutes after adding 500 μl of isopropanol. The RNA was pelleted down by centrifuging the above for 10 minutes at 10,000 rpm, and the pellet was vortexed gently with 300 μl of 70% of ethanol. The extracted RNA was treated with DNase enzyme to remove any traces of DNA contamination. One microliter of DNase was added to the above RNA and incubated for 1 hour at 37°C, after which the temperature was raised to 70°C for 5 minutes to inactivate the enzyme. The RNA was stored at −20°C for later use. The concentration and purity of RNA were assessed using a spectrophotometer (Sartorius India Pvt. Ltd., Bangalore, India). A 1 μL aliquot of RNA was pipetted onto the apparatus pedestal. RNA with an absorbance ratio at 260 and 280 nm between 1.8 and 2.2 will be deemed indicative of pure RNA. The ratio obtained for the samples collected was approximately 2, indicating pure RNA.
After quantification, the RNA was reverse transcribed using Oligo dT (Sigma Aldrich, Saint Louis, Mo). One-hundred nanograms of RNA were aliquoted to a fresh sterile microcentrifuge tube, and 2 μl of oligo dideoxy thymine was added and incubated at 70°C for 5 minutes and immediately transferred to ice. To this 2 μl of dNTPs, 1 μl of reverse transcriptase enzyme (New England BioLabs, Beverly, Mass) and 2 μl of 10X reverse transcriptase buffer was added and made up to the volume to 25 μl using RNase free water. This mixture was incubated at 42°C for 90 minutes, and the reaction was terminated by incubating at 70°C for 15 minutes.
The primers for Relative quantification analysis were designed using Primer Express software of Applied Biosystems (Foster City, Calif). The melting temperature was calculated and adjusted, and the synthesized primers were purified by high-performance liquid chromatography. Beta-actin was taken as the housekeeping gene. The sequences of primers were mentioned in Table I . A gradient polymerase chain reaction was performed to standardize the optimum annealing temperature of the designed primer using 30 ng of synthesized cDNA, keeping the temperature range between 50°C-60°C. The optimum temperature was confirmed at 60°C for all the primers.
Gene symbol | Sequence | Length | Tm, °C | GC content, % |
---|---|---|---|---|
PGE2 | FP 5′ CCACCTCATTCTCCTGGCTA 3′ | 20 | 50.3 | 56 |
RP 5′ CGACAACAGAGGACTGAACG 3′ | 20 | 53.8 | 55 | |
IL1B | FP 5′ ACAGATGAAGTGCTCCTTCCA 3′ | 23 | 55.3 | 48 |
RP 5′ GTCGGAGATTCGTAGCTGGAT 3′ | 26 | 54.8 | 38 | |
ALP | FP 5′ CCCCCCGTGGCAACTCTATCTT 3′ | 22 | 54.8 | 50 |
RP 5′ GTAGTTCTGCTCGTGGACGCCG 3′ | 23 | 55.3 | 48 | |
ACP | FP 5′ CTTTCTACCGCCTGCACTTC 3′ | 20 | 53.8 | 55 |
RP 5′ GCTGTTTCTTGAGCCAGGAC 3′ | 20 | 53.8 | 55 | |
RANKL | FP 5′ CATCGGGTTCCCATAAAG 3′ | 18 | 48.0 | 50 |
RP 5′ GAAGCAAATGTTGGCGTA 3′ | 18 | 45.8 | 44 | |
OPG | FP 5′ ACAGTTTGCCTGGGACCAAA 3′ | 20 | 51.8 | 50 |
RP 5′ TCACAGAGGTCAATGTCTTGGA 3′ | 22 | 53.0 | 45 | |
BGLAP | FP 5′ GCAGCGAGGTAGTGAAGAGA 3′ | 20 | 53.8 | 55 |
RP 5′ GGAGGTCAGGGCAAGG 3′ | 16 | 51.1 | 69 |
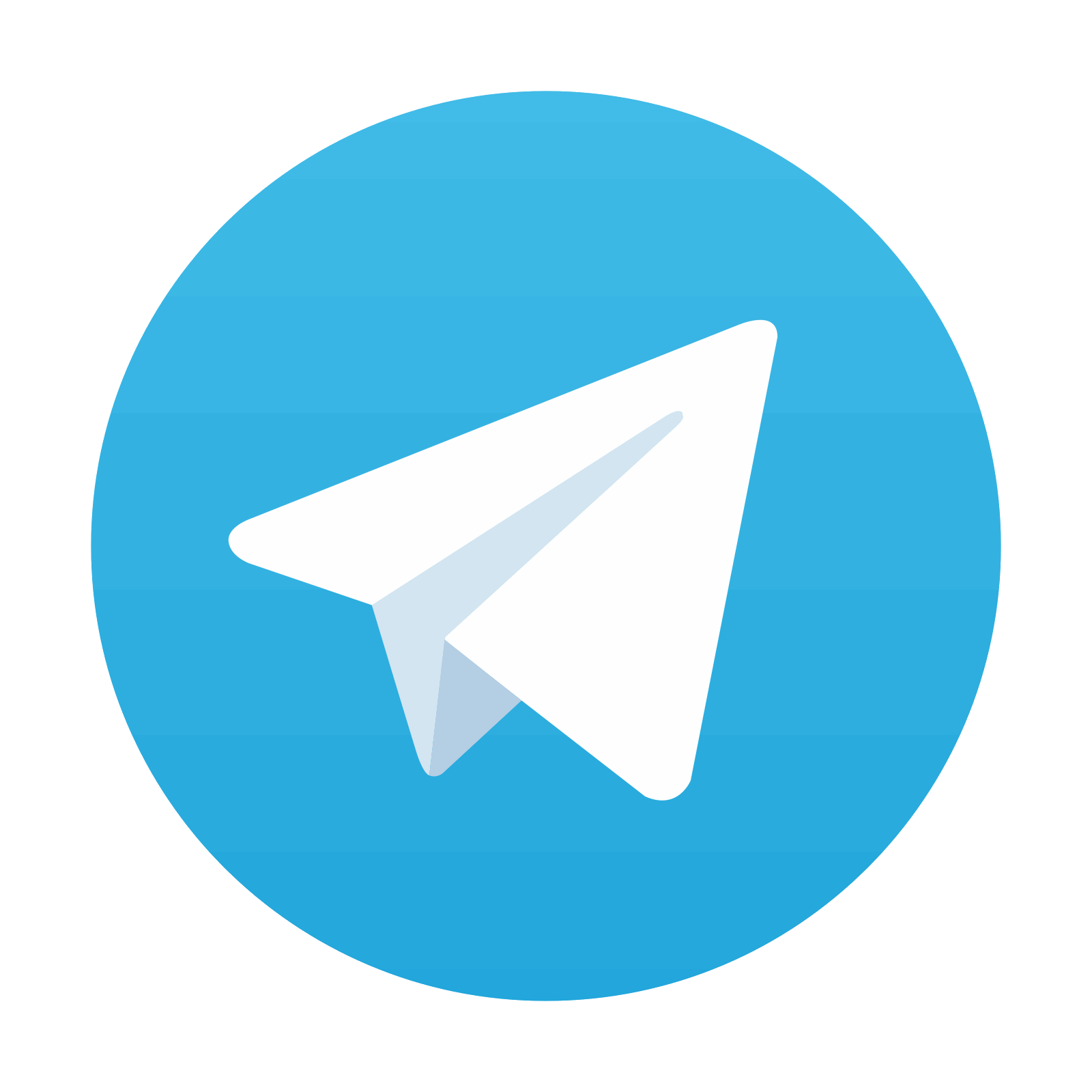
Stay updated, free dental videos. Join our Telegram channel

VIDEdental - Online dental courses
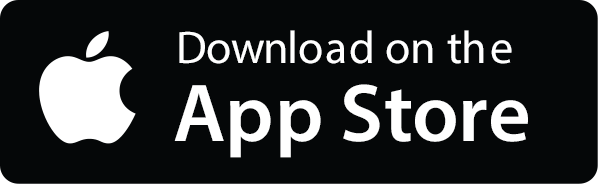
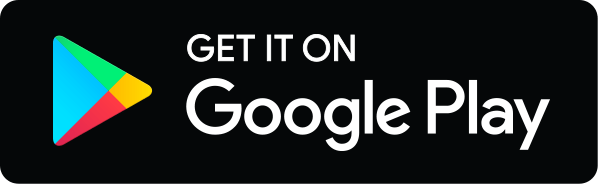
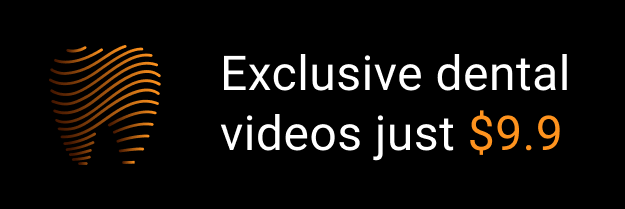