Introduction
Although the detrimental health effects of cigarettes are well known, many people, including some orthodontic patients, continue to smoke. Nicotine can affect bone resorption and apposition. Therefore, the aim of this study was to investigate the effect of nicotine on orthodontic tooth movement.
Methods
Thirty-two adult male Sprague-Dawley rats were randomly divided into 3 experimental groups and 1 control group. With the rats under general anesthesia, 5-mm nickel-titanium closed-coil springs were placed between the maxillary right first molars and first incisors. Injections of nicotine were administered daily for 13 days: group A, 0.5 mg per kilogram; group B, 0.75 mg per kilogram; and group C, 1 mg per kilogram. Group D, the control group, received injections of 0.1 mL of normal saline solution. At the end of the experimental period, the rats were killed, and interproximal tooth movements were measured.
Results
The mean amounts of tooth movement were 0.47 mm in group A, 0.62 mm in group B, 0.78 mm in group C, and 0.21 mm in group D. Statistical analysis with analyis of variance (ANOVA) test showed significant differences between all groups. The most movement occurred in group C, and group D had the least movement.
Conclusions
Nicotine accelerates orthodontic tooth movement, and this effect is dose-dependent. Nicotine’s role in accelerating tooth movement might be related to its effect of accelerating bone resorption.
Cigarette smoking is a major cause of many fatal diseases and premature deaths in many countries. Smoking increases the progression of atherosclerosis and cardiovascular diseases and is highly associated with diabetes, thyroid abnormalities, and gastrointestinal diseases. It is an important risk factor for lung, prostate, and pancreatic cancers. It also has detrimental effects on other people as passive smokers. In addition, smoking has injurious intraoral effects; it increases the progression of periodontal disease and is the major cause of oral cancer. Investigations of American students have shown that 8.1% of middle school students and 22.3% of high school students smoke cigarettes, and about 20% of American adults are smokers. About 15% of all orthodontic patients are adults, and the rest are adolescents and children. Therefore, it seems that the rate of smoking among orthodontic patients is not negligible. Regarding these investigations and considering the effects of smoking on decreasing health-related quality of life, orthodontists can play a critical role in encouraging their patients to stop cigarette smoking, a major preventable cause of disease and death.
Nicotine is an alkaloid from the oxygen-free alkaloid group, which is the major psychoactive component of cigarette smoke that is highly addictive if inhaled via binding to nicotinic cholinergic receptors in the brain and releasing neurotransmitters such as dopamine and by causing neuroadaptation (tolerance). Nicotine also can result in dependence and withdrawal syndrome.
Many studies have investigated nicotine`s cellular and molecular effects. Investigations of nicotine’s effect on cultured cells suggest that it can influence bone resorption and apposition. Nicotine causes an increase in the expression of the cyclooxygenase-2 (COX-2) gene and prostaglandin E2 (PGE2) release in human gingival fibroblasts in a time and dose-dependent manner. COX is the main enzyme for converting arachidonic acid to prostaglandins, which are important factors in bone resorption. Therefore, nicotine administration might increase orthodontic tooth movement. In preosteoblastic cells, nicotine promotes the cell cycle and inhibits differentiation related to p53 regulation. In rats, daily administration of nicotine adversely affects the periodontal tissues in a dose-dependent manner.
Several human and animal studies have focused on the effects of medications and hormonal changes on orthodontic tooth movement. Regarding these effects on bone and gingival and periodontal tissues, we expected nicotine to affect orthodontic tooth movement because, based on Wolff’s theory, orthodontic tooth movement can be considered a kind of bone remodeling.
Considering the data about the relatively remarkable prevalence of smoking among orthodontic patients, and the cellular and molecular effects of nicotine, we need more studies to investigate the effects of cigarette components on orthodontic tooth movement. Because of the detrimental effects of nicotine, it is not ethical to perform such studies on human subjects. Therefore, the purpose of this study was to investigate the effect of nicotine on orthodontic tooth movement in rats and learn whether different doses of nicotine have different effects on this phenomenon.
Material and methods
Thirty-two adult male Sprague-Dawley rats weighing 250 ± 20 g were selected from the animal-keeping center of the pharmacy faculty at Tehran University of Medical Sciences in Iran. The rats were kept in plastic cages under a standard 12-hour light and 12-hour dark photoperiod at a temperature of 21°C ± 2°C and humidity of 55%. They were fed standard rat food (0.8%-1.2% calcium and 0.7%-0.9% phosphorus, combined with 3060-kg units of vitamin D) and sufficient water. All experiments were performed according to the guidelines of the US National Institutes of Health (publication 85-23, revised 1985).
At the beginning of the study, the animals were weighed. To ensure the rats’ health and to calculate the dosage of solution for injection, they were also weighed daily during the study.
The rats were anesthetized by intraperitoneal injection of a mixture of 0.9 mg per kilogram of xylazin HCl and 70 mg per kilogram of ketamine to their left groin.
The rats were randomly divided into 4 equal groups: 3 experimental groups and 1 control group. An identical orthodontic force was applied for all groups. The first group (A) underwent orthodontic force application and daily injections of 0.5 mg per kilogram of nicotine (nicotine tartrate salt, Merck, Darmstadt, Germany, dissolved in normal saline solution). For the second group (B), orthodontic forces were applied, and daily injections of 0.75 mg per kilogram of nicotine were given. The third group (C) had orthodontic force applications and daily injections of 1 mg per kilogram of nicotine. For the last group (D), the control group, we applied the same orthodontic forces and gave daily injections of 0.1 mL of normal saline solution. All injections were given intraperitoneally in rats’ left groin for 13 days.
The force application system consisted of a 5-mm nickel-titanium closed-coil spring (Hitek, 0.006 × 0.022 in, 3M Unitek, Monrovia, Calif) placed between the maxillary right first molar and the right incisor with a ligature wire (0.001-in stainless steel, Dentaurum, Ispringen, Germany). The first molar in rats has a slight bulge on the mesiopalatal surface; passing a ligature wire under the bulge increases the retention significantly. Due to a lack of undercuts in the incisor area, a cervical groove was prepared in the gingival third of each tooth by using an 0.8-mm diamond bur. The teeth were etched with 37% phosphoric acid for 40 seconds and washed with sterilized water. Then, for each central incisor, the ligature wire was placed on the groove and fixed by resin composite (self-cured, Degufill, Degussa, Frankfurt, Germany). A 60-g force was produced for each specimen by a 2-mm activation of the spring via elongation of the spring up to 7 mm.
Fourteen days after appliance placement, the animals were anesthasised with ether. In each rat, the amount of orthodontic tooth movement between the maxillary right first molar and incisor was measured by using an interproximal feeler gauge (Mitutoyo co, Kawasaki-shi, Japan). This gauge has several sheets of different thicknesses: the thickest sheet is 0.63 mm, and the thinnest one is 0.04 mm. Measurements were performed before untying the orthodontic appliance to prevent possible errors resulting from relapse.
An operator made all measurements and then was blinded, and all measurements were repeated. The mean value was used as the final measurement.
Analysis of variance (ANOVA) and the Tukey test were used to determine whether there were significant statistical differences between the groups ( P <0.05).
Results
After force application, we measured the mount of tooth movement in all specimens ( Table ). One-way ANOVA showed significant differences in the amounts of tooth movement between all groups ( P <0.001). The Tukey test showed that the amounts of tooth movement in groups A, B, and C, which had received nicotine, were significantly higher than in the control group (D) ( P <0.001). In addition, it showed that the amount of tooth movement in group C (mean, 0.78 mm) was significantly higher than in group B (mean, 0.62 mm) ( P <0.001); in group B, the amount of tooth movement was significantly higher than in group A (mean, 0.47 mm) ( P <0.001). The control group (D), which had received no nicotine, showed the least amount of tooth movement (mean, 0.21 mm); it was significantly less than in the nicotine groups ( P <0.001).
Group | Mean movement (mm) | SD | Maximum movement (mm) | Minimum movement (mm) |
---|---|---|---|---|
A (0.5 mg/kg nicotine) | 0.47 | 0.66 | 0.55 | 0.38 |
B (0.75 mg/kg nicotine) | 0.62 | 0.33 | 0.68 | 0.58 |
C (1 mg/kg nicotine) | 0.78 | 0.47 | 0.85 | 0.73 |
D (1 mL normal saline solution) | 0.21 | 0.43 | 0.28 | 0.17 |
Results
After force application, we measured the mount of tooth movement in all specimens ( Table ). One-way ANOVA showed significant differences in the amounts of tooth movement between all groups ( P <0.001). The Tukey test showed that the amounts of tooth movement in groups A, B, and C, which had received nicotine, were significantly higher than in the control group (D) ( P <0.001). In addition, it showed that the amount of tooth movement in group C (mean, 0.78 mm) was significantly higher than in group B (mean, 0.62 mm) ( P <0.001); in group B, the amount of tooth movement was significantly higher than in group A (mean, 0.47 mm) ( P <0.001). The control group (D), which had received no nicotine, showed the least amount of tooth movement (mean, 0.21 mm); it was significantly less than in the nicotine groups ( P <0.001).
Group | Mean movement (mm) | SD | Maximum movement (mm) | Minimum movement (mm) |
---|---|---|---|---|
A (0.5 mg/kg nicotine) | 0.47 | 0.66 | 0.55 | 0.38 |
B (0.75 mg/kg nicotine) | 0.62 | 0.33 | 0.68 | 0.58 |
C (1 mg/kg nicotine) | 0.78 | 0.47 | 0.85 | 0.73 |
D (1 mL normal saline solution) | 0.21 | 0.43 | 0.28 | 0.17 |
Discussion
Orthodontic tooth movement is based on bone remodeling, a complicated process. This process is influenced by numerous local and systemic factors. The rate of smoking among orthodontic patients is remarkable. Nicotine is one of the most psychoactive components of cigarette smoke and seems to influence bone resorption and apposition. Therefore, it is necessary to investigate the effects of nicotine on orthodontic tooth movements.
In this study, we used closed-coil springs to produce the orthodontic forces. In a clinical study, Samuels et al compared space closure by nickel-titanium closed-coil springs with space closure by elastic modules. They concluded that space closure by nickel-titanium closed-coil springs is more consistent than by elastic modules.
We chose the nicotine doses based on the study of Nociti et al about the effect of nicotine on rats’ periodontal tissues. Intravenous injection of nicotine is a logical way to simulate smoking; Rose et al showed that the plasma nicotine level after smoking the usual brand of cigarettes is almost as same as this level after intravenous nicotine administration.
The results of our study showed that administration of nicotine increases the orthodontic tooth movement rate in rats significantly, and the orthodontic tooth movement rate also has a dose-dependent response to daily administration of nicotine. This significant difference might be explained by the following reasons.
Long-term tobacco smoking a risk factor for osteoporosis and can lead to bisphosphonate treatment, which can decrease tooth movement rapidly and interfere with desirable orthodontic results. Therefore, long-term smoking might decrease tooth movement because of other medications that are used, even though 1 isolate, nicotine, can increase tooth movement. Although several studies have investigated the effects of nicotine on osteoclasts and osteoblasts, differences in cell culture conditions, different stages of cell differentiation, different cell types, and different doses of nicotine have led to variable results in these studies. These results were taken from in-vitro studies that were different from in-vivo conditions. In spite of the differences in results, these studies are unanimous that nicotine can affect bone metabolism and might be a reason for acceleration of orthodontic tooth movements.
Henemyre et al investigated the effect of nicotine on porcine osteoclast function measured by resorption of calcium and phosphate. They concluded that nicotine leads to increases in the number and resorptive functions of osteoclasts. This effect might help to accelerate orthodontic tooth movement.
Lahmouzi et al, via ultrastructural analysis, investigated the effect of nicotine on rats’ gingival fibroblasts cultured in vitro. They concluded that nicotine might directly affect some functions of rats’ gingival fibroblasts, increase apoptosis in these cells, and lead to mitochondrial dilation and vacuolation, which are necrotic signs. They also showed that alterations in the doses of nicotine can change the survival rate of these cells; this might be related to our finding that increased dosages of nicotine led to increased rates of tooth movement. Furthermore, another in-vitro investigation showed that nicotine can change some cellular functions in human gingival fibroblasts and periodontal ligament fibroblasts. These authors also concluded that increasing the dose of nicotine can enhance some of its effects; this helps us to explain our result about the effect of increasing the nicotine dosage. Although these studies were in vitro, it is possible that -nicotine might have effects on these cells in vivo that led to acceleration in orthodontic tooth movement.
It was reported that nicotine can lead to up-regulation of PGE2 in human neutrophils and monocytes. Chang et al reported that stimulation of quiescent human gingival fibroblasts with nicotine resulted in COX-2 mRNA and protein induction in these cells. It is well known that cyclooxygenase is one of the most important enzymes that contributes to the conversion of arachidonic acid into prostaglandins and thromboxanes, and prostaglandins (especially PGE-2) are important mediators in bone remodeling and orthodontic tooth movement. Therefore, the acceleration of orthodontic tooth movement caused by nicotine might be mediated via increased production of PGE-2. Chang et al also showed that the higher the amount of nicotine, the greater the production of PGE-2; this might be a reason for an increase in the rate of tooth movement with an increase in the dosage of nicotine.
Hapidin et al investigated the effects of nicotine on bone-resorbing cytokines and bone histomorphometric parameters in male rats. They concluded that nicotine administration significantly increased the serum IL-1 and IL-6 levels and significantly decreased trabecular bone volume, trabecular thickness, mineralizing surface, mineral appositional rate, and bone formation rate. It also caused an increase in the osteoclast surface. These are all resoptive response features that might explain the acceleration of orthodontic tooth movement caused by nicotine. Unfortunately, they used only 1 dose of nicotine; therefore, it is impossible to predict whether the effects would be altered by increasing the doses of nicotine.
Several studies showed that nicotine has direct effects on the small blood vessels in causing vasoconstriction and systemic venoconstriction, which lead to hypoxia. Based on the pressure-tension theory, hypoxia in the pressure side leads to chemical mediator production that leads to osteoclast differentiation and bone resorption. The hypoxia caused by nicotine might contribute to the production of chemical mediators in the pressure side and accelerate bone resorption and tooth movement.
Although our results have shown acceleration of orthodontic tooth movement with nicotine, all of its detrimental effects on health and highly addictive features, and the dangerous consequences of smoking, absolutely do not rationalize smoking. Orthodontists, like other health care professionals, should try to encourage their patients to stop smoking.
Nicotine replacement therapies such as nicotine gums and patches are available; they have been shown to be effective for reducing smoking in several studies. Although nicotine is addictive when inhaled in tobacco smoke, it rarely results in addiction if it is delivered orally or through the skin ; this might be because of its slower delivery to brain. Because of the effectiveness of nicotine replacement therapies and since our results showed the accelerated effects of nicotine on orthodontic tooth movement, we suggest that orthodontists can advise their patients to use nicotine replacement therapies to help them stop smoking. Their consumption should be monitored by a physician, so that it will not interfere with orthodontic treatment.
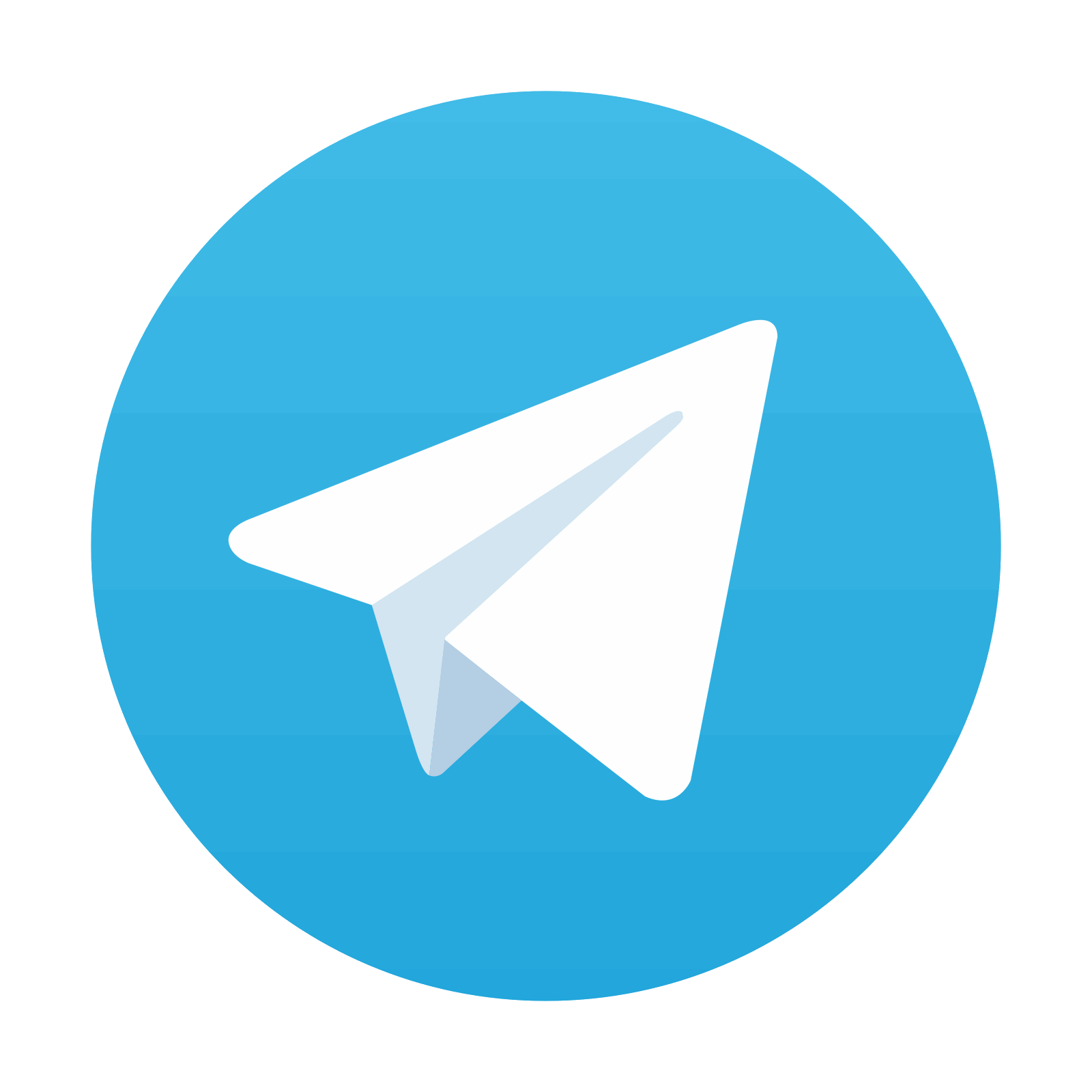
Stay updated, free dental videos. Join our Telegram channel

VIDEdental - Online dental courses
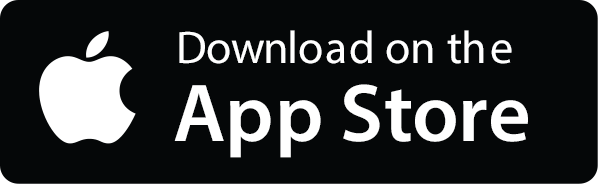
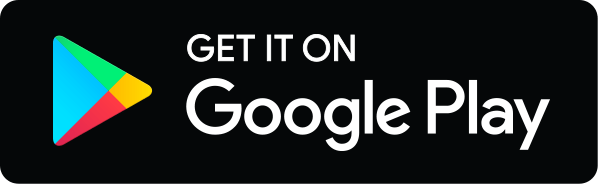