Introduction
Posttreatment relapse is a major challenging clinical issue. The objective of this study was to evaluate the effect of local administration of simvastatin on posttreatment relapse.
Methods
Orthodontic tooth movement was induced in 10 white New Zealand rabbits. After 21 days of active tooth movement, the orthodontic appliances were removed, and the experimental teeth were allowed to relapse for 21 days. During the relapse phase, 1 mandibular quadrant received local simvastatin administration, and the other received the control vehicle solution on a weekly basis. Three-dimensional models of the experimental teeth were created to allow the measurement of experimental tooth movement and posttreatment relapse. The animals were killed at the end of the relapse phase for histomorphometric analysis of alveolar bone remodeling.
Results
The mean relapse percentages were 75.83% in the quadrant receiving the control vehicle solution and 62.01% in the quadrant receiving simvastatin. Neither the relapse magnitude nor the relapse percentage showed a significant difference between the 2 quadrants. Histomorphometric analyses showed that local simvastatin administration yielded a significant reduction in the area of active bone-resorptive lacunae and a significant increase in newly formed bone area.
Conclusions
Although local administration of simvastatin aids in bone remodeling associated with posttreatment relapse by reducing the area of active bone resorption and upregulating bone formation, it did not significantly minimize posttreatment relapse.
Highlights
- •
Local simvastatin administration reduces the area of active bone resorption.
- •
Local simvastatin administration upregulates bone formation.
- •
Local simvastatin administration does not inhibit postorthodontic relapse.
Relapse is a physiologic response of the supporting tissues to force application and has been a major challenging clinical issue with respect to the goals of successful orthodontic treatment. The classic approaches to orthodontic retention primarily involve the use of fixed or removable retainers whose caveats include reliance on patient compliance and a long duration of retention to ensure stability.
Various systemically and locally administered agents have been reported to reduce the amount of relapse in animal models, including bisphosphonate, osteoprotegerin, relaxin, and bone morphogenetic proteins. Although the mechanisms of action are different, relapse is decreased by modification of the remodeling process of the dental supporting tissues. These findings prompted the possibility of safe pharmacotherapeutic strategies designed to manipulate alveolar bone remodeling to minimize postorthodontic relapse.
A hypolipidemic agent, simvastatin, has recently been expected to serve as a therapeutic agent for osteoporosis. These drugs, known as statins, represent a new approach for treating osteoporosis; one that emphasizes building new bone to replace bone that has deteriorated. Of 30,000 natural compounds, Mundy et al identified statins as the only natural product that specifically increases bone morphogenetic protein-2 (BMP-2) gene expression in osteoblasts and increases levels of bone formation proteins in these cells. There was also a striking increase in osteoblast cell numbers after statin application, since BMP-2 is one of the most potent growth factors targeting bone formation in vivo, which stimulates osteoblastic differentiation and proliferation.
Inhibition of the 3-hydroxy-3-methyl-glutaryl-coenzyme-A reductase enzyme and the subsequent blockage of the mevalonate pathway is probably the most important mechanism of inhibition of bone resorption by simvastatin. Yazawa et al showed that relatively low concentrations of simvastatin promote cell proliferation and osteoblastic differentiation. Lee et al observed increased bone formation and higher maximum force to fracture after local application of statins in rat mandibles. Ozec et al showed that local application of simvastatin significantly increased the density of bony defects in rat mandibles.
Statins, including simvastatin, showed biologically significant antioxidant, anti-inflammatory, and anabolic effects on osteoblastic bone formation that could prove beneficial in orthodontics. Han et al showed that the systemic administration of simvastatin could minimize postorthodontic relapse by inhibiting the bone-resorbing activity of osteoclasts and stimulating bone formation. Moreover, the impressive long-term safety profile of simvastatin renders it a suitable agent for use in orthodontic therapy.
In this study, we used an experimental rabbit model to explore the effect of local administration of simvastatin on postorthodontic relapse. It was hypothesized that the osteoinductive effects of simvastatin on the dental supporting tissues as well as the inhibition of alveolar bone resorption might minimize postorthodontic relapse. The null hypothesis was that the local administration of simvastatin has no effect on either postorthodontic relapse or associated alveolar bone remodeling.
Material and methods
This study was conducted in accordance with the ARRIVE guidelines for animal studies and after approval from the ethics committee, Faculty of Dentistry, Alexandria University in Egypt.
Calculation of the required sample size was performed using the formula for studies comparing paired continuous data. Based on the results of a previous study yielding a standard deviation of 0.548 mm, we determined that a conservative sample of 9 rabbits would be sufficient to detect an effect size of a 0.7-mm difference in a split-mouth study with a power of 90% and a significance level of 0.05. Since we expected that some animals might not tolerate the experimental procedures and survive until the end of the experimental period, the sample size was increased to a total of 10 rabbits to allow for a 10% expected attrition.
Ten 16-week-old healthy male white New Zealand rabbits ( Oryctolagus cuniculus ) were included in the study. They had a body weight between 2.5 and 3.5 kg with normal development of dentition and mandibular incisors, premolars, and molars. All experimental procedures were performed under general anesthesia by intramuscular injection of ketamine (ketamine alfasan 10%; Alfasan Group of Companies, Woerden, The Netherlands) and xylazine (xyla-ject injectable solution; Adwia Pharmaceuticals, 10th of Ramadan City, Egypt).
The rabbits were kept under standardized light and dark cycles at the animal house of Alexandria Medical Research Institute. They had free access to a soft standard diet to minimize the incidence of appliance breakage.
A prospective randomized split-mouth experimental trial was implemented in each rabbit. The appliance used to produce tooth movement was similar to the model described by Pithon and Ruellas. All rabbits received an orthodontic appliance consisting of a 13-mm nickel-titanium closed-coil spring (Jiscop, Gyeonggi-do, Korea) stretched between the mandibular first premolars and incisors bilaterally. The activation force of the spring was measured with a force gauge (Correx; Haag-Streit, Koniz, Switzerland) so that the spring provided a force of 100 cN. A ligature wire was used to encircle the mandibular second premolar, first molar, and second molar and tied to stabilize them in position and minimize the influence of interseptal fibers. The appliance was left in place for 21 days to achieve appreciable tooth movement ( Fig 1 ).

At the end of active tooth movement, the orthodontic appliances were removed, and the experimental teeth were allowed to relapse for 21 days. Each mandibular quadrant was randomly assigned to 1 of 2 experimental groups. In the control group (A), the teeth were allowed to relapse with a local injection of control vehicle solution on days 21, 28, and 35, and in the simvastatin group (B), the teeth were allowed to relapse with a local injection of simvastatin on days 21, 28, and 35. The assignment of each mandibular quadrant to an experimental group was performed using a computer-generated list of random numbers. The investigator (M.M.) generating the list was blinded to the treatment groups.
Simvastatin powder (PHR1438; Sigma-Aldrich, St Louis, Mo) was prepared at a concentration of 0.5 mg per 480 μl of solution. Pluronic F-127 (Sigma-Aldrich) acted as the carrier for the simvastatin in group B. The pluronic control vehicle solution without simvastatin was administrated in group A.
Two routes of local administration were implemented in each quadrant ( Fig 2 ). Intraligamentous injection was performed using an intraligamentous injector (Saniject; Saniswiss, Geneva, Switzerland) into the distal periodontal space of the mandibular first premolar delivering 180 μl of solution. Submucosal injection was performed using a 0.5-ml insulin syringe with a 31-gauge ultrafine needle (Insumed 31G × 8 mm; Pic Solution, Artsana, Grandate, Italy) close to the distal surface of the mandibular first premolar delivering 300 μl of solution. The solutions were injected by the principal investigator (H.A.) blinded to the contents of the syringes.

Impressions of the experimental teeth were made on days 21 and 42 using injection-type silicone vinyl polysiloxane impression material (Express; 3M ESPE Dental Products, St Paul, Minn) loaded into previously fabricated custom trays. The impressions were then poured using an improved die stone (Elite Rock Dental Stone; Zhermack, Badia Polesine, Italy).
Casts of the experimental teeth were scanned using a 3-dimensional scanner (InEos X5; Sirona Dental Systems, Bensheim, Germany) to create 3-dimensional models in .stl file format. Using Viewbox software (Viewbox for Windows, version 4.0.1.7; dHal Software, Kifissia, Greece), the models were oriented according to the mandibular occlusal plane determined by the cusp tips of the mandibular molars and second premolar. Two planes were drawn perpendicular to the mandibular occlusal plane. The first plane was drawn touching the most distal contact area of the distal surface of the mandibular first premolar. A second plane was drawn touching the most mesial contact area of the mesial surface of the mandibular second premolar. The amount of tooth movement was determined by measuring the linear distance (parallel to the mandibular occlusal reference plane) between the 2 constructed planes ( Fig 3 ). Measurements were performed using the ruler tool of the Viewbox software. This method, similar to that proposed by Vieira et al with modifications, was used to minimize measurement errors from tipping and extrusion movements on the mandibular first premolar. Magnitudes of tooth movement, postorthodontic relapse, and relapse percentage were calculated.

Measurements were performed in a blinded fashion by the principal investigator. The intraexaminer errors for tooth movement measurements were assessed by repeating the measurements of 30 randomly selected 3-dimensional models, 2 weeks apart, by the same investigator. Intraclass correlation coefficients were calculated to assess intrarater reliability. The Bland-Altman analysis was conducted to quantify the level of the agreement between the 2 repeated measurements.
At the end of the postorthodontic relapse phase, 3 animals were randomly selected for histologic analyses. After they were killed, their mandibles were dissected, cut into halves, fixed, and decalcified. Parasagittal serial sections of 6-μm thickness were obtained, and 5 randomly selected sections per specimen were processed. The sections were stained with hematoxylin and eosin. Sections in the interdental area between the mandibular first and second premolars were evaluated under a light microscope (Primo Star; Carl Zeiss, Oberkochen, Germany) equipped with a 5-megapixel digital camera, and images of representative areas were captured and described. Histologic sections including the coronal and middle thirds of the root of the mandibular first premolar and the adjacent alveolar bone were examined. Histomorphometric analysis was performed on the former tension sides (changed into compression sides during relapse) along the distal aspect of the root of the mandibular first premolar.
After image scale calibration using Image J software (Image J software for Windows, version 1.50i; National Institutes of Health, Bethesda, Md), a square grid overlay with a side length of 1 mm was superimposed over the histologic sections, with the 2 opposite sides of the square perpendicular to the root surface of the mandibular first premolar. Histomorphometric parameters, including osteoclastic count, osteoblastic count, newly formed bone area, and area of active bone-resorptive lacunae, were evaluated in the designated square area according to a previously described method by Igarashi et al. Newly formed bone area was evaluated using a previous method reported by Garrett.
Histologic analysis was performed in a blinded manner by the principal investigator. Histomorphometric parameters were remeasured by the same investigator 2 weeks later on 15 randomly selected slides. Intraclass correlation coefficients were calculated to assess intrarater reliability.
Statistical analysis
The Statistical Package for the Social Sciences software (version 23; IBM, Armonk, NY) was used for statistical analysis. Kolmogorov-Smirnov and Shapiro-Wilk tests were conducted to assess the normality assumption. Once verified, paired-samples t tests were conducted to compare mean values between the groups. Otherwise, Wilcoxon Signed Ranks test was used.
Results
Two experimental rabbits failed to survive and were excluded from the study. Since the percentage of attrition was higher than estimated, 1 rabbit fulfilling the eligibility criteria was procured, and the same experimental procedures were performed to satisfy the required sample size of 9 rabbits per group. The rest of the animals tolerated the experimental procedures well, with no discernible effects on overall health.
Mean values and standard deviations calculated for the magnitudes of tooth movement and postorthodontic relapse as well as relapse percentages in groups A and B are summarized in Table I .
Magnitude of movement (mm) ∗ | Magnitude of relapse (mm) ∗ | Relapse percentage † | |
---|---|---|---|
Group A | 1.53 ± 0.34 | 1.15 ± 0.39 | 75.83 ± 20.33 |
Group B | 1.55 ± 0.36 | 1.01 ± 0.54 | 62.01 ± 28.94 |
∗ Data presented as means and standard deviations.
† Data presented as means and standard deviations of corresponding tooth movement.
In group A, the magnitude of tooth movement was 1.53 ± 0.34 mm, and the magnitude of postorthodontic relapse was 1.15 ± 0.39 mm, yielding a relapse percentage of 75.83% ± 20.33% of the achieved tooth movement. In group B, the magnitude of tooth movement was 1.55 ± 0.36 mm, and the magnitude of postorthodontic relapse was 1.01 ± 0.54 mm, yielding a relapse percentage of 62.01% ± 28.94% of the achieved tooth movement.
Results of Kolmogorov-Smirnov and Shapiro-Wilk normality tests for magnitudes of tooth movement, postorthodontic relapse, and relapse percentage confirmed the assumption of normality.
Results of paired-samples t tests, given in Table II , showed no significant difference between magnitudes of tooth movement in groups A and B ( P = 0.883).
Paired differences | Significance (2 tailed) | |||||
---|---|---|---|---|---|---|
Mean | SD | SE of mean | 95% CI of the difference | |||
Lower | Upper | |||||
Pair 1 | ||||||
Movement magnitude A-movement magnitude B | −0.0200 | 0.394 | 0.131 | −0.323 | 0.283 | 0.883 |
Pair 2 | ||||||
Relapse magnitude A-relapse magnitude B | 0.141 | 0.790 | 0.263 | −0.466 | 0.749 | 0.607 |
Pair 3 | ||||||
Relapse percentage A-relapse percentage B | 13.819 | 37.650 | 12.550 | −15.122 | 42.760 | 0.303 |
When the relapse magnitudes between the 2 groups were compared, the results of paired-samples t test showed no significant difference between magnitudes of postorthodontic relapse in groups A and B ( P = 0.607). Similarly, no significant difference was reported in the percentage of postorthodontic relapse between groups A and B ( P = 0.303).
The estimated intraclass correlation coefficient for the repeated measurements of tooth movement was 0.994, showing strong evidence for the reliability of these measurements. Bland-Altman analysis was performed with a resulting coefficient of repeatability of 0.01 mm, showing a high level of agreement for most of the measurements, excluding fixed or systematic bias.
Histologic sections in control group A along the distal aspect of the root of the mandibular first premolar showed slowly maturing bone with few osteoblasts. In some areas, bone appeared less dense and scalloped with few osteoclasts in resorption bays denoting some bone loss. In other areas, resorption bays were observed without osteoclasts, whereas successive parallel dark lines were noted, indicating bone apposition. Dense periodontal fibers showed regular distribution at some areas and irregular arrangement at others. Extravasated blood vessels were observed at random areas of the alveolar bone ( Figs 4-6 ).



Histologic sections in simvastatin group B along the distal aspect of the root of the mandibular first premolar showed an increase in the amount and maturation of alveolar bone. Extensive accretion of alveolar bone was observed on hematoxylin and eosin-stained reversal lines. Newly formed alveolar bone showed many appositional lines parallel to each other including signs of a normal remodeling pattern. The alveolar bone surface appeared relatively smooth with small resorption lacunae and an increased number of osteoblasts bordering the alveolar bone surface. Relatively few osteoclasts were observed along the alveolar bone surface. Extravasated blood vessels were also noted at random areas of the alveolar bone. Compared with group A, bundles of principal fibers and fibroblasts showed a more regular arrangement pattern without inflammatory infiltrate or hyaline zones.
Mean values and standard deviations were calculated for the histomorphometric parameters analyzed in the interdental area; along the distal aspect of the root of the mandibular first premolar in groups A and B ( Table III ).
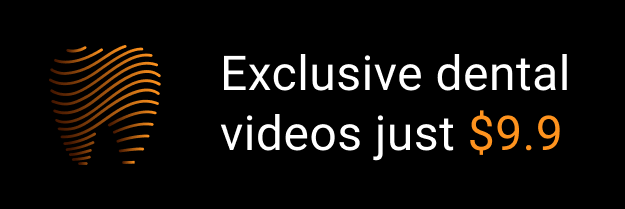