Highlights
- •
HF followed by silane and self-etching ceramic primer promoted higher flexure strength.
- •
Sandblasting with Al 2 O 3 and silicatization produced damages to all ceramics evaluated.
- •
Self-etching ceramic primer showed lower roughness values.
Abstract
Objective
To investigate the influence of different surface treatments on biaxial flexure strength, roughness, and surface topography of lithium silicate/disilicate-based ceramics.
Methods
225 discs (∅: 12 mm; 1.2 mm – ISO 6872) were made from three ceramics: IPS e.max CAD (LD – Ivoclar Vivadent), Suprinity (LSS – Vita) and Celtra Duo (LSC – Dentsply). The samples were randomly divided into 5 groups (n = 15): no treatment (C); 10% hydrofluoric acid + silane (HF); sandblasting Al 2 O 3 + silane (SB); silicatization + silane (SC); and self-etching ceramic primer (SEP). After surface treatment, a resin cement layer was applied to the disc surface (RelyX U200, 3M ESPE), mechanical cycled (1.2 × 10 6 cycles, 50 N, 3.8 Hz) and submitted to biaxial flexural strength test (1 mm/min, 1000 Kgf). Roughness, EDS and SEM were also performed. Data were analyzed by one-way ANOVA, Tukey test (5%) and Weibull.
Results
ANOVA revealed that the “surface treatment” factor was significant for all ceramics (p < 0.05). The groups LD-HF (289.30 ± 40) LD-SEP (298. 87 ± 53.29), LSC-HF (195.51 ± 42.12), LSS-HF (269.58 ± 27.07) and LSS-SEP (207.45 ± 28.63) presented significantly higher biaxial flexure strength than respective control groups, except for the LSC-SEP (165.41 ± 33.86), which was statistically similar to the control. The Weibull modulus was significantly higher for the LD-SB, LSC-SC groups. Additionally, the LD-SB, LSC-SC and LSS-HF groups showed higher roughness compared to the other treatments.
Significance
HF etching followed by silanization and self-etching ceramic primer are the most suitable surface treatments for lithium silicate/disilicate-based glass-ceramics.
1
Introduction
In order to achieve better prosthetic results, Dentistry has evolved to the use of restorations produced by the CAD/CAM system (computer-aided design/computer-aided manufacturing), which has become a widespread technique in recent decades [ ]. This technique has the advantage of allowing the manufacture of indirect restorations in a single session [ ], excellent precision and adaptation of restorations [ ], besides to using industrially manufactured ceramic blocks, which have less internal defects, thus improving the mechanical strength of restorations [ ]. Materials with different compositions and microstructures are available for CAD/CAM, such as glass-ceramics based on lithium disilicate (LD) and lithium silicate (LS), which has recently been available on the market [ ]. According to the manufacturers, zirconium oxides were added to the composition of lithium silicate in order to improve the mechanical properties of glass-ceramics [ , ]. However, according to recent studies, these ceramics cannot be considered as reinforced by zirconia, since it is only found dissolved in the glass phase, not as crystal particulate [ ]. For this reason, they will be consider in the present study as lithium silicate-based glass-ceramics.
Only two LS commercials brand are currently available on the market: Celtra Duo (Dentsply) and Suprinity (VITA Zahnfabrik). Both materials are mainly composed of two crystalline phases: lithium metasilicate (Li 2 SiO 3 ) and lithium orthophosphate (Li 3 PO 4 ), immersed in a glassy matrix [ , ]. An important difference between these materials is the crystallization state in which they are available for milling. Suprinity is available in pre-crystallized state, so a crystallization firing after milling is necessary for achieving its ideal color and density [ ]. Celtra Duo, on the other hand, is available in fully crystallized state, thus the restoration can be finished after milling and polishing, which reduces the treatment time, or the firing step can also be carried out in order to increase the mechanical strength of the material [ ]. in vitro studies describes a flexure strength of LS similar [ ] or lower than LD [ ]. In addition, an excellent clinical performance of LS-based partial restorations was observed in follow-up periods of 1–3 years [ , ], as well as for LD-based full crowns, which have a longer follow-up, reaching 12 years [ ]. Despite these results, fracture has been one of the main causes of failure reported in these studies [ ].
Thus, the surface treatment of ceramics is one of the factors that might influence the mechanical performance of the restorative material [ , ]. Surface treatment before cementation seeks to modify the ceramic surface to create micromechanical retentions, increase in roughness, as well as promoting a chemical adhesion between ceramic and resin cement [ ]. Hydrofluoric acid (HF) etching associated with silane has been the most indicated treatment for LS and LD [ , ] ceramics. However, due to the risk of excessive degradation of the glass-ceramic matrix [ ] and the toxic potential of HF [ , ], other surface treatments have been studied such as: self-etching ceramic primer (SEP, Monobond Etch & Prime, Ivoclar Vivadent) [ , ], sandblasting with particles of aluminum oxide (Al 2 O 3 ) [ ] and silicatization associated with silanization [ ]. The SEP combines the etching and silanization steps and has shown effective adhesion between these ceramics and the resin cement [ ]. On the other hand, sandblasting with Al 2 O 3 particles produces surface roughness that promotes mechanical retention to resin cement [ ], being a method used in laboratories to abrade [ ] and cleaning [ ] the internal surface of the ceramic. Silicatization (sandblasting of Al 2 O 3 particles coated with silica), in its turn, promotes the formation of roughness and the deposition of silica on the ceramic surface, making it chemically reactive for the adhesion of resin cement through silane [ ]. Nonetheless, the adhesion promoted by these last two treatments has shown controversial results in the literature [ , , ].
There are still no studies in the literature that have evaluated the effect of different surface treatments on the mechanical properties of cemented LS. In a previous study with a different type of glass-ceramic (leucite glass-ceramic), the presence of the cementing agent significantly increased the mechanical resistance of the material [ ]. Therefore, the objective of this study was to verify the effect of different surface treatments on the biaxial flexure strength, roughness and microstructure of LS and LD-based ceramics after cementation and mechanical cycling. The tested hypotheses were: (1) the surface treatment, regardless of the type, influences the flexural strength of the LS and LD ceramics; (2) sandblasting promotes greater surface roughness compared to other treatments.
2
Material and methods
2.1
Materials
The materials used in this study, as well as their respective brands, manufacturers, batches and composition are shown in Table 1 .
Brand | Type of material | Manufacture | Batch | Composition |
---|---|---|---|---|
Celtra Duo – HT / A3 | Lithium silicate | Dentsply | 18027977 | SiO 2 ; Al 2 O 3 ; Li 2 O; K 2 O; ZrO 2 ; Al, among others |
Suprinity – HT / A3 | Lithium silicate | VITA Zahnfabrik | 40020 | ZrO 2 , SiO 2 , LiO 2 , La 2 O 3 , among others |
IPS e.max CAD – HT / A3 | Lithium disilicate | Ivoclar Vivadent | W04573 | SiO 2 Li 2 O, K 2 O, MgO, Al 2 O 3 , P 2 O 5 and other oxides |
RelyX Ceramic Primer | Silane | 3M ESPE | N822741 | Ethyl alcohol, water and 3-trimethoxysilylpropyl methacrylate |
Aluminum oxide | Aluminiun oxide 50 μm in powder | Bio-art | 52160 | Al 2 O 3 |
Condac Porcelain | 10% Hydrofluoric acid | FGM | 171116 | Hydrofluoric acid, water, thickener, surfactant and dye |
Rocatec Soft 30 μm | Aluminum oxide silica-coated | 3M ESPE | 1803100248 | Al 2 O 3, SiO 2 |
Monobond Etch & Prime | Self-etching ceramic primer | Ivoclar Vivadent | V50443 | Ammonium polyfluoride alcohol, silane methacrylate and dye |
Cement RelyX U200 | Self-adhesive resin cement | 3M ESPE | 660958 | Base paste: methacrylate monomers containing phosphoric acid groups, methacrylate monomers, silaneted fillers, initiator componentes, stabilizers, rheological additives. Catalyst parte: mathacrylate monomers, alcaline (basic) fillers, silanated fillers, initiator componentes, stabiliazers, pigments, rheological additives |
2.2
Sample preparation
Two hundred and twenty-five discs (∅: 12 mm; thickness: 1.2 ± 0.2 mm – ISO 6872 [ ]) were made from ceramics: lithium disilicate (LD, IPS e.max CAD, Ivoclar Vivadent, Schaan, Liechtenstein) and two lithium silicate-based glass-ceramics (LSS, Vita Suprinity – Vita Zahnfabrik, H. Rauter GmbH & Co., Bad Säckingen, Germany; and LSC, Celtra Duo – Dentsply Sirona, Hanau, Wolfgang, Germany). Subsequently, the samples were regularized with grit SiC abrasive papers with 240, 400, 600 and 1200# granulations, in a polishing machine (Sander PL02, Metalprisma, São Paulo, SP, Brazil). All samples underwent to a crystallization cycle carried out in a specific oven (Programat EP5000, Ivoclar Vivadent, Schaan, Liechtenstein), according to the manufacturer’s recommendations. The samples of each type of ceramic were randomly divided into five groups (n = 15), according to the factor “surface treatment” (5 levels).
2.3
Surface treatments
All samples were submitted to an ultrasonic cleaning with distilled water for 8 min (Cristófoli Biosafety Equipment LTDA, Paraná, Brazil) and samples of each ceramic were randomly divided according to the following groups:
- •
Control (C): without treatment.
- •
10% Hydrofluoric acid (HF) + Silane: 10% hydrofluoric acid etching (FGM, Joinville, SC, Brazil) for 20 s followed by washing with air–water spray (40 s) and air-dried. Afterwards, using a microbrush, a layer of Silane (RelyX Ceramic Primer, 3M ESPE, St Paul, MN, USA) was applied, reacting for 2 min.
- •
50 μm aluminum oxide (SB): sandblasting with 50 μm Al 2 O 3 (Bio-art, São Carlos, SP, Brazil) using a microblaster (Dento-PrepTM, RØNVIG, Daugaard, Denmark). The distance between the ceramic surface and the tip of the microblaster was standardized at 10 mm and the inclination at 45°. The pressure applied was 2.8 bar/10 s. Then, using a microbrush, a layer of Silane was applied, reacting for 2 min.
- •
Silicatization (SC): sandblasting with silica coated Al 2 O 3 particles (Rocatec® 30 μm/3 M ESPE, USA), using a microblaster (Dento-PrepTM, RØNVIG). The distance between the ceramic surface and the tip of the microblaster was standardized at 10 mm and the inclination at 45°. The pressure applied was 2.8 bar/10 s. Then, using a microbrush, a layer of Silane was applied, reacting for 2 min.
- •
Self-etching ceramic primer (SEP): with the aid of a microbrush (Vigodent, Rio de Janeiro, RJ, Brazil), Monobond Etch & Prime (Ivoclar Vivadent, Schaan, Liechtenstein) was actively applied to the ceramic surfaces for 20 s, then reacting for another 40 s. After this period, the ceramic surface was washed with air-water spray (60 s) and air-dried.
2.4
Bonding cementation
After the surface treatments of the ceramics, a layer of RelyX U200 self-adhesive resin cement (3M ESPE, St Paul, MN, USA) was applied, following the manufacturer’s recommendations, with a cement spatula #24 for 10 s. Immediately after, the cement was applied in the center of the treated surface of each ceramic unit. Subsequently, a glass cover was placed on the cement in order to spread it over the entire surface of the ceramic disk. With the aid of an adapted parallelometer (BioArt, São Carlos, SP, Brazil) a 750 g load was applied to the glass cover, ensuring uniform flow and a thin cement thickness. After 1 min, the glass cover was removed, sliding it over the surface of the ceramic disk and the cement was photopolymerized for 40 s (Valo, 1400 mW/cm 2 , Ultradent, Salt Lake City, USA). After cemented, all samples were stored in distilled water at 37 °C for 24 h.
2.5
Mechanical fatigue
All samples were subjected to mechanical cycling in a mechanical fatigue simulator (ERIOS, Model: ER-11000, São Paulo, SP, Brazil) for 1.2 × 10 6 cycles with a constant load of 50 N and frequency of 3.8 Hz. The load was applied in the center of the samples by a stainless steel piston with rounded tip and with a radius of curvature of 1.5 mm. The treated face was positioned downwards (tension area), the load being applied to the surface that did not receive any treatments.
2.6
Biaxial flexure strength
The final dimensions of each specimen were measured with a digital caliper (Absolute, Mitutoyo, São Paulo, Brazil) and after they were subjected to biaxial flexure strength test in a universal testing machine (EMIC, DL-1000, São José dos Campos, SP, Brazil). A tungsten flat piston (ISO 6872 [ ], Ø = 1.6 mm) attached to the load cell, applied an increasing load in the center of the opposite face to the treated one (compression area), at a speed of 1 mm/min and 1000 Kgf load cell until reaching a catastrophic fracture. After fracture, for the calculation of the biaxial flexure strength (MPa) of each sample, the data obtained (N) were placed in the equation, according to the ISO 6872 [ ] standard:
S=-0,2387P(X-Y)/b2
Where S is the maximum tension force, in MPa; P is the total load required to cause the fracture, in N; X and Y are parameters related to the elastic properties of the material (Poisson’s ratio and elastic module) and b is the thickness of the sample at the origin of the fracture, in mm.
2.7
Optical profilometry
Additional samples (∅: 12 mm; thickness: 1.2 mm) from each group (n = 3) were made and their surfaces were analyzed using a digital optical profilometer (Wyko, Model NT 1100, Veeco, Tucson, USA) connected to a computer with imaging software (Vision 32, Veeco, USA), in order to perform surface micrographs (qualitative analysis of three-dimensional geometry – 3D) and measurement of surface roughness. For this, 12 readings were performed on each sample and an arithmetic mean (Ra) of the surface roughness was obtained using the proper system software.
2.8
Scanning Electron Microscopy (SEM)
The same samples analyzed in optical profilometry (n = 3) were used and their surfaces were analyzed using the Tescan Scanning Electron Microscope (Model MIRA 3, Kohoutovice, Czech Republic).
2.9
Energy-dispersive spectroscopy (EDS)
The samples used in the previous analyses (n = 3) were submitted to the analysis of chemical elements present in the ceramic material by means of Energy-dispersive X-ray spectroscopy (EDS). The EDS spectrometer works coupled to the scanning electron microscope (SEM) using the Oxford Instruments system (Model X-MAXN) with AZtec Live software. The readings of each sample were performed at a working distance of 12 mm and 20 kV of acceleration voltage. No conductive coverage was applied to the samples. For each measured area of the sample, the main elements were analyzed with real time for 100 s.
2.10
Statistical analysis
The power of the study was calculated using the OpenEpi website ( www.openepi.com ), by comparing the higher and the lower means and standard deviations of the experimental groups, for each ceramic type, considering a 95% confidence interval and the sample size per group of 15. Shapiro-Wilk test was performed to evaluate de normality. The results of this test indicated that the data of the groups follow a normal distribution ( P > 0.05).
The numerical values obtained through biaxial flexure strength test and optical profilometry were subjected to analysis of variance (one-way ANOVA) and Tukey’s multiple comparison test (5%) for each type of ceramic. Statistical analysis was performed using program STATISTIX (Analytical Software Inc., version 8.0, 2003). The level of significance adopted was the conventional value of 5%.
Weibull analysis measures the reliability of the biaxial flexure strength of the material by Weibull modulus (m) and characteristic strength (σ 0 ). Weibull modulus indicates the structural homogeneity of the material considering strength distribution. Characteristic strength expresses the resistance at a failure probability of approximately 63.3%. Weibull modulus and characteristic strength with a confidence interval of 95%, were calculated by lnσ c –ln [ln 1/(1-F(σ c )] diagram (according to ENV 843-5):
lnln11-Fσc=mlnσc-mlnσo
Statistical analysis was performed at Minitab software (version 17, 2013, Minitab, State College, PA). The level of significance was 5%.
3
Results
The power of 100% was obtained for LD and LSS ceramics and 97.58% for LSC.
3.1
Biaxial flexure strength
The one-way ANOVA revealed that surface treatment significantly influenced the biaxial flexure strength for all evaluated ceramics: LD (p < 0.00001), LSC (p = 0.0002) and LSS (p < 0.00001) ( Table 2 ). For LD ceramics, the SEP (298.87 ± 53.29 A ) and HF (289.30 ± 40.11 A ) groups promoted an increase in flexural strength, while SC (189.19 ± 31.49 C ) generated a lower resistance flexural strength compared to the control group (236.21 ± 34.63 B ). For the LSC ceramic, only the HF (195.51 ± 42.12 A ) was statistically superior to the control group (139.24 ± 36.06 B ). Finally, for LSS ceramics, HF (269.58 ± 27.07 A ) and SEP (207.45 ± 28.63 B ) also significantly increased flexural strength compared to the control group (194.81 ± 22.81 C ). The SB treatment (142.91 ± 13.57 D ) caused a significant reduction in flexural strength compared to the control group ( Table 3 ).
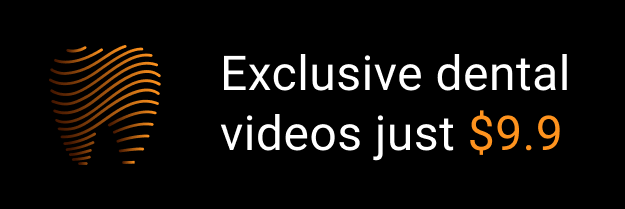