Introduction
Anchorage, which is defined as resistance against undesired tooth movements, is one of the most important factors in success of orthodontic treatment. In recent years, mini-implants have been used instead of uncomfortable headgears and Nance appliances. The pullout test is the most common method for measuring the anchorage capacity of mini-implants. The aim of this study was to investigate the effects of cortical bone thickness and cortical layer bone density on pullout strength of mini-implants.
Methods
Mini-implants were placed in polyurethane foam blocks representing 3 different cortical thicknesses (1 mm, 2 mm, and 3 mm) and 3 different cortical bone densities (grade 40, grade 45, and grade 50). Pullout tests were performed with 5 mm/min loading rate. Load vs displacement values were recorded during the tests.
Results
Comparing cortical thicknesses on the same bone densities, statistically significant differences were found between 1 mm, 2 mm, and 3 mm thicknesses. Likewise, comparing bone densities on the same cortical thicknesses, statistically significant differences were found among all groups. Spearman rank tests showed that both cortical thickness and cortical bone density are correlated with pullout strength (rs, 0.548; P <0.001 and rs, 0.691; P <0.001, respectively).
Conclusions
Although both factors are positively correlated with pullout strength, the effect of cortical bone density was the dominant factor affecting primary stability.
Highlights
- •
Mini-implants were placed in blocks simulating cortical bone.
- •
Effects of bone thickness and density on pullout strength were assessed.
- •
Both cortical layer density and thickness were positively correlated with pullout strength.
- •
Density has a greater impact on pullout strength than thickness.
- •
1-mm cortical thickness is the threshold value for pullout strength.
Traumatic or developmental disorders may occur in the human jaw system. A study among American people showed that only 35% of adults have well-aligned incisors. Anchorage is used during the treatments of such common disorders. Anchorage is defined as resistance against unwanted tooth movement. Intraoral and extraoral devices were developed for orthodontic anchorage. Nance appliances and headgears are used, but because of social and esthetic reasons, these methods are not preferred. Mini-implants were invented to overcome such problems. A recent study showed that mini-implants have a 90% success rate. Mini-implants have some important advantages, such as increased patient comfort, short healing time, higher hygiene standards, and lower treatment costs. ,
In the literature, there are many studies about the success rate of mini-implants and factors affecting it. Park et al showed that the success rate of mini-implants was 91.6% and that implanting in the maxilla or mandible and implanting side significantly affected the success rate. Motoyoshi et al calculated the success rate of mini-implants as 80% of patients who have <1-mm cortical shell thickness and 91.5% of patients who have cortical shell thickness >1 mm. Maxilla and mandible bones have different physical properties. Deguchi et al found that cortical shell thickness is greater at the mandible than at the maxilla. Miyamoto et al made cortical shell thickness measurements and found that the thickness at the mandible ranged between 0.79 mm and 3.21 mm and the thickness at the maxilla bone ranged between 0.92 mm and 2.54 mm. Pullout is one of the most common ways for evaluating anchorage performance of implants. A study in fresh-frozen human cadavers showed that bone mineral density is an important factor on implant pullout strength. A study in bovine pelvic bones indicated that there is positive correlation between pullout strength and bone mineral density. Huja et al claimed that cortical bone thickness has an important effect on pullout strength in dogs.
Specimens taken from human and animal cadavers were used in biomechanical experiments because their features are close to in vivo tissues. However, using synthetic materials in tests have become important recently because of ethical issues and difficulties of providing and conserving cadaver specimens. In the literature, there are studies comparing the quality of the synthetic bone specimens in pullout tests. , , The aim of this study was to compare the effect of cortical shell thickness and cortical shell density on pullout performance of mini-implants.
Material and methods
Mini-implants (Tasarýmmed, Istanbul, Turkey) of 1.6 mm in diameter and 8 mm in length were used for pullout tests, as shown in Figure 1 . The main properties of mini-implants were listed as self-tapping, self-drilling, anodize surface, and manufactured from TiAl6V4-ELI grade 23 titanium alloy.

Polyurethane (PU) foam blocks were used for bone models in pullout tests according to American Society for Testing and Materials (ASTM) F 543. PU foam blocks were prepared according to ASTM F1839-09 Standard Specification for Rigid Polyurethane Foam for Use as a Standard Material for Testing Orthopedic Devices and Instruments. Rigid PU foam blocks were divided into groups according to their densities and cortical thicknesses. Density ranges for determining the block grades were specified according to ASTM F1839-09 standards. PU foam blocks were produced from polyol and poly-isocyanate with closed-die casting method. Chemicals were poured into a mold and mixed until a homogeneous mixture was obtained, and then the mold was closed ( Fig 2 ). If the process does not occur in a closed mold, volume of composed foam keeps growing until the process is over. In a known volume, by using determined amounts of polyol and poly-isocyanate, PU foam blocks with stated grades were produced. To simulate bone with cortical and trabecular tissues, 2-layered PU foam blocks were produced with a 2-phased molding method. First, high grade PU foam blocks used as cortical layer were obtained and cut into 8-mm-thick slices ( Fig 2 ). After that, these slices were placed into a mold again ( Fig 2 ). After the second phase of molding, 2 layered PU foam blocks with an 8-mm-thick cortical shell were obtained. To make the cortical bone shell thinner, a milling procedure was applied.

Consisting of 3 different cortical shell thicknesses and 3 different cortical bone densities, in total, 9 different rigid PU foam blocks were produced ( Table I ). In all 9 groups of 2-layered PU foam blocks, the trabecular part was grade 25 PU foam and naturally bonded with the cortical shell. The cortical shell thickness groups were chosen as 1, 2, and 3 mm. The selection of the cortical layer thickness was based on the studies about mean thickness of the maxilla and mandibula. Miyamoto et al reported that the mean maxilla cortical thickness was 1.49 ± 0.34 mm (n = 98, range 0.92-2.54), and the mean mandible cortical thickness was 2.22 ± 0.47 mm (n = 127, range 0.79-3.21). Based on these parameters, the cortical layer thicknesses chosen for this study were 1, 2, and 3 mm. The density groups of cortical shells were determined as grade 40, grade 45, and grade 50, which were specified between the density values of grade 40 and grade 50. In all 9 groups of 2-layered PU foam blocks, the trabecular part was grade 25 PU foam and naturally bonded with cortical shell ( Fig 3 ).
Foam block code | Specification cortical thickness, cortical grade |
---|---|
40-1 | 1-mm thick, grade 40 cortical layer |
40-2 | 2-mm thick, grade 40 cortical layer |
40-3 | 3-mm thick, grade 40 cortical layer |
45-1 | 1-mm thick, grade 45 cortical layer |
45-2 | 2-mm thick, grade 45 cortical layer |
45-3 | 3-mm thick, grade 45 cortical layer |
50-1 | 1-mm thick, grade 50 cortical layer |
50-2 | 2-mm thick, grade 50 cortical layer |
50-3 | 3-mm thick, grade 50 cortical layer |

The mini-implants were manually inserted close to the center line of PU foam block without any pilot drilling. Mini-implants on each block were inserted on the same line, and care was taken for enough gap between the mini-implants to prevent pullout process effects on the other mini-implants. After insertion, all samples were placed on the loading frame (Instron 5944, 2kN; Instron, Norwood, Mass), as shown in Figure 4 . A total of 90 pullout tests were performed that consist of 10 samples for each group. Load vs displacement values were recorded during the tests.

After all pullout tests were conducted, PU foam blocks used during the tests were examined, and samples from the trabecular layer of each block were taken. Trabecular density of the samples was determined.
Statistical analysis
Data analysis was performed using SPSS statistical software for Windows (version 11.5; IBM, Armonk, NY). The Shapiro-Wilk test was used to determine if the distributions of continuous variables were normal. We used nonparametric Mann-Whitney U test to compare differences in groups. Spearman correlation coefficients were used to determine correlations between the variable pairs. A 2-way analysis of variance (ANOVA) was conducted with bone thickness and density as discriminating variables.
Taguchi method uses a loss function to calculate the deviation between the experimental and desired values, then loss function is converted into signal/noise (S/N) ratio. There are 3 major categories of the Taguchi method in the analysis of S/N ratio: (1) lower is better, (2) higher is better, and (3) nominal is better. Because the highest pullout value was desired, the higher is better category was chosen. ANOVA is a statistical method used to determine the individual interactions of all the parameters. Using ANOVA together with the Taguchi method gives better results for an evaluation of the effects of the parameters. P values were used to determine whether the differences were statistically significant. A P value of <0.05 was considered statistically significant.
Results
Mean and standard deviation values of pullout strength of the specimens in each group were given in Table II . Trabecular density of the samples was determined and given in Table II . Trabecular bone density was kept as the same bone density for all groups. The mean pullout strengths of the grade 40 bone density group were 203 ± 5 N in 1-mm cortical thickness, 230 ± 13 N in 2-mm cortical thickness, and 215 ± 11 N in 3-mm cortical thickness. There were statistically significant differences between pullout strength of 1-, 2-, and 3-mm cortical thickness in grade 40 bone density group ( Table III ).
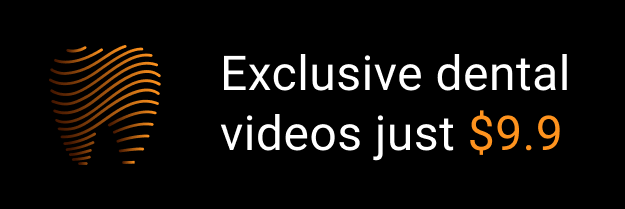