Introduction
Histamine (H 1 ) receptor antagonists are widely used drugs for treatment of allergic conditions. Although histamine was shown to be involved in bone remodeling, the aim of this study was to determine the effects of cetirizine, an H 1 receptor antagonist, on bone modeling processes during orthodontic tooth movement.
Methods
We used 3 groups of Wistar rats: control group (n = 16), appliance-only group (n = 16) and cetirizine group (n = 16). Each animal of the last 2 groups was fitted with a superelastic closed-coil spring appliance and treated daily with saline solution or cetirizine. Tooth movement was measured weekly from day 0 to day 42. Gene expression levels for bone turnover markers cathepsin K and osteocalcin were determined by means of real-time polymerase chain reaction. Histologic samples were analyzed by using histomorphometry.
Results
Cetirizine decreased the amount of tooth movement from day 28 onward ( P <0.01), and it also decreased osteoclast volume density ( P <0.001). An increase in alveolar bone volume density was observed in the cetirizine group ( P <0.01) compared with the appliance-only group. No statistically significant differences were observed in osteoclast activity, osteoblast volume density, and osteoblast activity between the cetirizine and the appliance-only groups.
Conclusions
Cetirizine influences bone modeling, mainly by inhibiting bone resorption. Therefore, H 1 receptor antagonists could interfere with orthodontic treatment.
Antihistamines are widely prescribed drugs. Histamine (H 1 ) receptor antagonists are mostly used in the treatment of allergic rhinitis and chronic idiopathic urticaria. These chronic diseases are common in the general population; allergic rhinitis affects up to 60 million people in the United States, whereas chronic idiopathic urticaria affects up to 3% of the population at some time in their lives. Therefore, it is highly possible that some orthodontic patients are prescribed H 1 receptor antagonists during their orthodontic therapy.
Histamine is involved in bone remodeling—osteoclasts first resorb bone, and then osteoblasts deposit bone at the same location—and in bone modeling, the process in which the shape of the bone is changed. Both osteoclasts and osteoblasts were found to express H 1 receptors. The role of histamine in bone remodeling is more pronounced in situations of high bone turnover but has little or no impact on basal bone remodeling. Histamine promotes the formation of osteoclasts in vitro indirectly via H 1 receptors on osteoblasts. H 1 receptors are also expressed on mature osteoclasts. In-vitro studies agree with in-vivo studies. In a study of postmenopausal women with pollen allergy, it was observed that H 1 receptor antagonists have beneficial effects on the risk of bone fractures. In a synchronized resorption rat model, it was shown that mepyramine, an H 1 receptor antagonist, transiently inhibits bone resorption. Although the effect of histamine on bone resorption has been well studied, less is known about histamine involvement in bone formation. Mice lacking the capacity to synthesize histamine exhibited increased cortical bone thickness and mineral content, because of increased bone formation and reduced bone resorption. Histamine deficiency also increases bone formation, partly by increasing serum calcitriol levels, which consequently stimulate osteoblast activity. These findings contrast with those from an in-vitro study, in which it was found that histamine stimulates the maturation and the differentiation of osteoblasts.
Bone modeling is the key process in orthodontic tooth movement. It starts 20 to 30 days after force application to the tooth when sterile necrosis is removed. At the pressure side, osteoclasts degrade the alveolar bone to create space for the moving tooth. At the tension side, new bone is formed by activated osteoblasts that produce new extracellular matrix, which subsequently mineralizes. During orthodontic tooth movement, different mediators, which induce the bone-modeling processes, are produced by the periodontal ligament (PDL) and bone cells ; histamine is one of them. It has already been shown that cetirizine, an H 1 receptor antagonist, affects orthodontic tooth movement in rats. H 1 receptor gene expression level increased on days 14 and 42 after force application to the teeth.
The purpose of this study was to further evaluate the effects of cetirizine, an H 1 receptor antagonist, on bone resorption and bone formation during orthodontic tooth movement in rats.
Material and methods
All animal procedures and the study protocol were approved by the Veterinary Administration of the Republic of Slovenia (number 323-02-234/2005/2) and are in compliance with the guiding principles in the “Care and Use of Animals.” The study was performed with 48 male Wistar rats (320-340 g, 13-14 weeks old). The animals were housed in groups of 4 in polycarbonate cages under normal laboratory conditions at constant temperature (24°C-25°C) and humidity, with a 12-hour circadian cycle. They were fed with soaked standard laboratory rat chow diet (Krka, Novo mesto, Slovenia) and water ad libitum. The rat chow was soaked in water to facilitate food intake that was impaired by the orthodontic appliance. General anesthesia was used for placing the orthodontic appliance. To ensure general anesthesia, a mixture of 3 anesthetics was used. The anesthetics were injected intraperitoneally: ketamine at 50 mg per kilogram −1 of body weight (Bioketan, Vetoquinol Biowet, Gorzów Wielkopolski, Poland), medetomidin hydrochloride at 67 μg per kilogram −1 of body weight (Domitor, Pfizer, Brooklyn, NY), and thiopental at 3 mg per kilogram −1 of body weight (Tiopental, Pliva, Zagreb, Croatia).
The orthodontic appliance consisted of a superelastic closed-coil spring (25 cN; wire diameter, 0.15 mm; GAC International, Bohemia, NY), placed between the maxillary left first molar and the incisors. The superelasticity of the coil spring ensured a constant force (25 cN) during the whole time of coil-spring activation. The closed-coil spring was attached to the maxillary left first molar with a stainless steel ligature wire (diameter, 0.25 mm; Dentaurum, Ispringen, Germany) and to the incisors by a surgical steel wire (4-0, multifilament, W310 , Ethicon; Johnson & Johnson, New Brunswick, NJ). To improve the fixation of the appliance, a 0.5-mm hole was made by using a hard metal burr (HM 1, 204, 005, Meisinger, Neuss, Germany). The hole was drilled from the distal surfaces of the incisors, through the teeth, and perpendicular to the longitudinal axis of the incisor at the gingival level. The steel wire was inserted through the hole and bonded to the proximal surface of the right incisor.
The animals were divided into 3 groups, 1 control and 2 appliance groups (appliance-only and cetirizine). In the control group (n = 16), no orthodontic appliance was placed, and each animal received 0.1 mL of saline solution daily. In the appliance-only group (n = 16), each animal was fitted with an orthodontic appliance and received 0.1 mL of saline solution daily. In the cetirizine group (n = 16), each animal was fitted with an orthodontic appliance and received 3 mg of kilogram −1 of cetirizine (Krka) daily. All animals received saline solution or cetirizine at approximately the same time for 42 days. The orthodontic appliance was placed under general anesthesia at the beginning of the study and replaced to the correct position every 7 days to ensure its proper activation, and consequently a constant force was exerted on the teeth. On day 42, all animals were killed.
The distance between the most mesial point of the maxillary left first molar and the most palatal point of the ipsilateral incisor at the gingival level was measured in all animals. Measurements were made weekly with a digitronic caliper with an accuracy of ± 0.01 mm (Wilson & Wolpert, Utrecht, The Netherlands) while the animals were anesthetized. All measurements were made twice by 2 investigators (A.M., Š.S.) independently within a few minutes. Tooth movement was calculated by subtracting the distance between the teeth on each day of measurement (days 7, 14, 21, 28, 35, and 42) from the distance between the teeth measured the previous week.
Cathepsin K gene expression level was used to determine osteoclast activity, and osteocalcin gene expression level was used to determine osteoblast activity. On day 42, 8 animals from each of the 3 groups were killed with an intraperitoneal injection of anesthetics and carbon dioxide. Tissue samples of alveolar bone, with all 3 molars and their PDLs, were excised and frozen in liquid nitrogen and mechanically powdered. The total ribonucleic acid (RNA) content from 120 ± 30 mg of each powdered sample was isolated by using TRIzol reagent (Invitrogen, Carlsbad, Calif), according to the manufacturer’s instructions. RNA concentrations and quality were determined by the Agilent 2100 Bioanalyzer with an RNA microfluidics chip (Agilent, Santa Clara, Calif).
To prepare primary cDNA, 10 ng of total RNA was used along with a high-capacity cDNA archive kit, with the addition of RNase inhibitor (Applied Biosystems, Foster City, Calif). The reaction was conducted according to the manufacturer’s recommendations at a final volume of 100 μL. It proceeded at 25°C for 10 minutes and 37°C for 2 hours, and was stopped by inactivation at 99°C for 5 minutes. Oligonucleotides for cathepsin K and osteocalcin were chosen from predesigned assays (TaqMan Gene Expression Assays, Applied Biosystems, Rn00580723_m1, Rn01455285_g1, respectively). Thermal cycling comprised initial steps at 50°C for 2 minutes and at 95°C for 10 minutes, followed by 40 cycles at 95°C for 15 seconds and at 60°C for 1 minute. A standard curve was constructed with serial dilutions of a mix of a few samples. The cDNA was amplified and quantified by using a sequence detection system (SDS 7000, Applied Biosystems, Foster City, Calif). To exclude variations from different inputs of total mRNA to the reaction, data on cathepsin K and osteocalcin were normalized to an internal housekeeping gene, GAPDH, for which data was obtained by using TaqMan GAPDH predesigned assays (TaqMan Gene Expression Assays, Applied Biosystems, Rn01462662_g1). All reactions for standard samples and for samples from all 3 groups (control, appliance-only, and cetirizine) were performed in duplicate. The data were averaged from the values obtained in each reaction.
Bone histomorphometry was used to determine alveolar bone volume density, osteoclast volume density, and osteoblast volume density in all 3 groups. On day 42, the remaining 8 animals from each group were killed by intraperitoneal injection of anesthetics and carbon dioxide. Tissue samples of the maxilla containing all 3 molars were taken. Tissue specimens were prepared as described. Histomorphometry was performed by using a point-counting method. For this purpose, the stereologic cycloid grid system incorporated into the ocular of a light microscope (BX-60, Olympus, Tokyo, Japan) was used. All histomorphometric measurements and calculations were made according to the standard nomenclature. The alveolar bone area density, expressed as the percentage of the tissue area consisting of tooth, PDL, connective tissue, and bone marrow spaces (B.Ar/T.Ar), was determined at 10-times magnification. Osteoclast and osteoblast area densities, defined as the alveolar bone area covered with either osteoclasts (multi-nucleated cells at the alveolar bone surface) or osteoblasts (“round” cells at the alveolar bone surface) as a fraction of the total alveolar bone area (Oc.Ar/B.Ar or Ob.Ar/B.Ar), were determined at 40-times magnification. The borders of the tissue area examined were determined as described by Sprogar et al. Figure 1 shows the examined area. Since 20 sections were examined from each part (apical, middle, and cervical) of the root of the maxillary left first molar, the 2-dimensional term “area density” was extrapolated to the 3-dimensional term “volume density.” Alveolar bone area density, osteoclast area density, and osteoblast area density were extrapolated to alveolar bone volume density (BV/TV), osteoclast volume density (Oc.V/BV), and osteoblast volume density (Ob.V/BV).
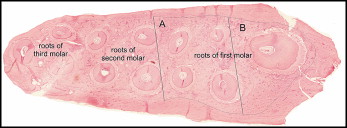
Statistical analysis
The data were expressed as means ± standard errors and calculated for each parameter for all animals of all groups (tooth movement, gene expression levels of cathepsin K and osteocalcin, alveolar bone volume density, and osteoclast and osteoblast volume densities).
Tooth movement was calculated from measurements of the distance between the teeth. Interexaminer reliability was tested by using the intraclass correlation coefficient (ICC), and a paired t test was used to assess the systemic bias. Analysis of systemic bias showed a P value of >0.88, and the ICC was 0.91 ± 0.02. The mean value of 4 measurements was used for statistical analysis.
Within-group and between-group comparisons were made for gene expression levels of cathepsin K and osteocalcin, and for the alveolar bone volume density (BV/TV), osteoclast volume density (Oc.V/BV), and osteoblast volume density (Ob.V/BV) with 1-way analysis of variance (ANOVA). A P value less than 0.05 was considered statistically significant.
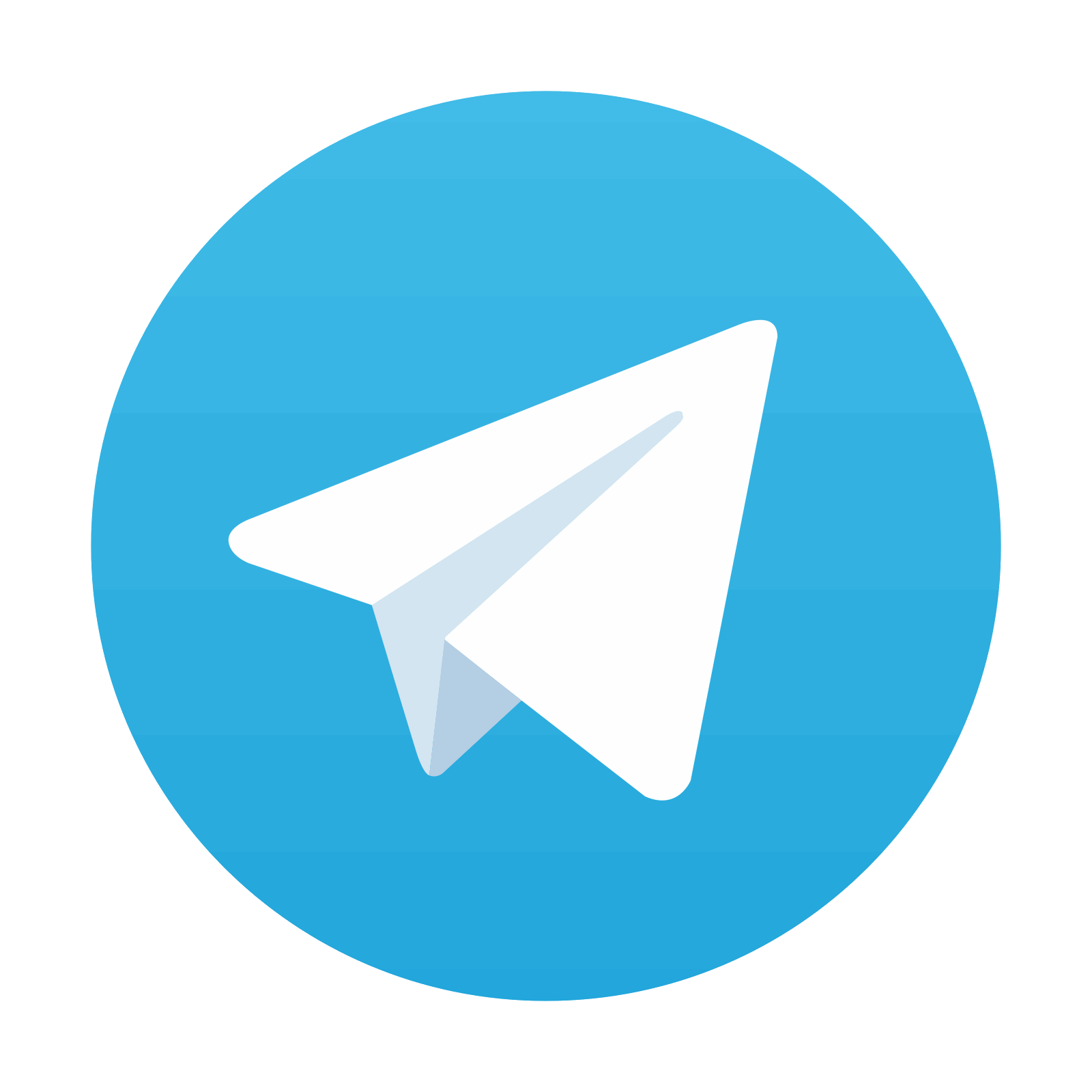
Stay updated, free dental videos. Join our Telegram channel

VIDEdental - Online dental courses
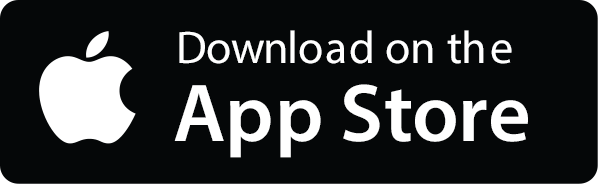
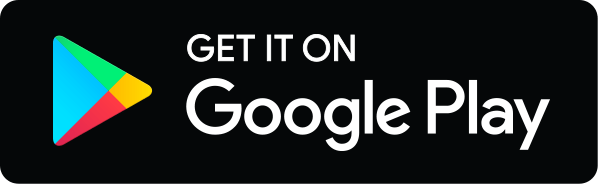