Abstract
Objectives
The effect of the ceramic infrastructure (IS) on the failure behavior and stress distribution of fixed partial dentures (FPDs) was evaluated.
Methods
Twenty FPDs with a connector cross-section of 16 mm 2 were produced for each IS and veneered with porcelain: (YZ) Vita In-Ceram YZ/Vita VM9 porcelain; (IZ) Vita In-Ceram Zirconia/Vita VM7 porcelain; (AL) Vita In-Ceram AL/Vita VM7 porcelain. Two experimental conditions were evaluated ( n = 10). For control specimens, load was applied in the center of the pontic at 0.5 mm/min until failure, using a universal testing machine, in 37 °C deionized water. For mechanical cycling (MC) specimens, FPDs were subjected to MC (2 Hz, 140 N, 10 6 cycles) and subsequently tested as described for the control group. For YZ, an extra group of 10 FPDs were built with a connector cross-section of 9 mm 2 and tested until failure. Fractography and FEA were performed. Data were analyzed by ANOVA and Tukey’s test ( α = 0.05).
Results
YZ16 showed the greatest fracture load mean value, followed by YZ16-MC. Specimens from groups YZ9, IZ16, IZ16-MC, AL16 and AL16-MC showed no significant difference for the fracture load.
Significance
The failure behavior and stress distribution of FPDs was influenced by the type of IS. AL and IZ FPDs showed similar fracture load values but different failure modes and stress distribution. YZ showed the best mechanical behavior and may be considered the material of choice to produce posterior FPDs as it was possible to obtain a good mechanical performance even with a smaller connector dimension (9 mm 2 ).
1
Introduction
The introduction of CAD/CAM (computer-aided design/computer-aided machining) technology in dentistry and the development of ceramics with high crystalline content increased the range of application of all-ceramic restorations . High-crystalline content ceramics, such as yttria stabilized tetragonal zirconia polycrystal (Y-TZP), alumina-based zirconia-reinforced glass-infiltrated ceramic (IZ) and polycrystalline alumina (AL), show higher fracture toughness values than those reported for glass-based materials , justifying their indication to produce all-ceramic fixed partial dentures (FPDs) .
On the other hand, as these ceramics show different chemical compositions and microstructure, different physical and mechanical properties are expected , which could influence the long-term clinical behavior of the restorations . Clinical trials on zirconia-based all-ceramic FPDs reported infrastructure failure rates as low as 6%. In these studies, most clinical failures were related to chipping of the porcelain veneer and secondary caries, which are not infrastructural failures. Yet, the follow-up periods for these clinical studies are still relatively short, varying from 2 to 5 years . One study successfully followed up three to five-unit Y-TZP FPDs for 10 years and reported a survival rate of 91.5% for the zirconia infrastructures . For all-ceramic FPDs, the probability of failure is related to the properties of the ceramic systems, the size and shape of the connectors, the span of the pontic and to the position in the oral cavity . FPDs most commonly fail from flaws located in the connector cervical area, where a higher stress concentration is produced during chewing .
Clinical studies provide reliable information on the longevity and failure behavior of dental restorations; however they are not easily carried out. On the other hand, laboratory tests and computer modeling represent more efficient research tools to obtain data on the stress distribution and failure behavior of dental prostheses . Caution should be taken to guarantee that in vitro experiment properly simulates the clinical situation in all of its complexity . The influence of the restoration configuration (multi-layer system) and its geometry in the stress distribution needs to be determined in order to better understand the long term behavior of all-ceramic prostheses. In vitro set-ups should take into account the processing steps used to produce dental restorations as to obtain the same flaw population leading to clinical failures . Therefore, a more reliable prediction of the failure behavior of ceramic restorations could be obtained through in vitro evaluation of specimens that reproduce the shape of dental restorations, such as crowns and fixed partial dentures (FPDs).
In vitro tests should also simulate the loading and environmental conditions observed in the oral cavity . Cyclic loading, as observed during chewing, contributes to the subcritical crack growth (SCG) of ceramic materials . Ceramic lifetime may be overestimated when predictions are based only on static load and fracture toughness data, without considering the effect of cyclic loading . When a ceramic material is subjected to a long period of intermittent stresses in a humid environment, below the critical stress level (< K Ic ) but above a threshold stress level (≥ K I0 ), pre-existing flaws may grow slowly in areas of stress concentration or near the surface. When these pre-existing flaws reach a critical size due to continue loading, the mechanical capacity of the material is exceeded, resulting in catastrophic failure . Under subcritical conditions, the initial flaw size distribution changes as a function of time, according to each material susceptibility to SCG. Thus, a different failure mode may occur for a prosthesis subjected to mechanical cycling in comparison to fast fracture .
The purpose of this study was to evaluate the effect of the ceramic infrastructure on the fracture load, failure mode and stress distribution of three-unit FPDs. This study tested the hypotheses that (1) the infrastructural material influences the failure behavior and stress distribution of FPDs and (2) the mechanical cycling effect on the fracture load of FPDs varies with the type of ceramic infrastructure.
2
Materials and methods
2.1
Specimen fabrication
FPDs were produced using three high crystalline-content infrastructure ceramics veneered with the recommended porcelain, as follows:
-
YZ – An yttria partially stabilized tetragonal zirconia polycrystal (Y-TZP) infrastructure (IS) (Vita In-Ceram YZ, Vita Zahnfabrik, Bad Sackingen, Germany) veneered with a porcelain (Vita VM9, Vita Zahnfabrik, Bad Sackingen, Germany);
-
IZ – A glass infiltrated zirconia-reinforced alumina-based ceramic IS (Vita In-Ceram Zirconia, Vita Zahnfabrik, Bad Sackingen, Germany) veneered with a porcelain (Vita VM7, Vita Zahnfabrik, Bad Sackingen, Germany);
-
AL – An alumina polycrystal IS (Vita In-Ceram AL, Vita Zahnfabrik, Bad Sackingen, Germany) veneered with a porcelain (Vita VM7, Vita Zahnfabrik, Bad Sackingen, Germany).
Stainless steel models simulating prepared abutment teeth were constructed with 4.5 mm height, 6° of taper and 120° chamfer as finish line . The distance between the centers of the dies was 16 mm, corresponding to the distance between a lower second premolar and a lower second molar (span of 10 mm). An artificial gingiva was produced with acrylic resin (JET, Classico, Sao Paulo, SP, Brazil). Polyvinyl siloxane impressions of the model were taken (Aquasil™, Dentsply, Petropolis, RJ, Brazil) and a working cast was made using type IV special CAD/CAM stone (CAM-base, Dentona AG, Dortmund, Germany).
The stone cast was digitized by the internal laser scanner component of CEREC inLab unit (Sirona Dental Systems, Charlote, NC, USA) to generate a tridimensional image that was used to design the FPDs infrastructures from YZ, IZ and AL ceramic systems. After the milling process, YZ and AL infrastructures were sintered using the Zyrcomat furnace (Vita Zahnfabrik, Bad Sackingen, Germany), and IZ infrastructures were glass infiltrated (Z21N Zirconia Glass Powder, Vita Zahnfabrik, Germany) using the Inceramat 3 furnace (Vita Zahnfabrik, Bad Sackingen, Germany), according to the manufacturer’s instruction. The excess glass was removed with airborne particle abrasion using 50-μm aluminum oxide particles. Only the external surface of the infrastructures was air abraded.
The infrastructures were veneered with the recommended porcelain, which were sintered using a Keramat I furnace (Knebel, Porto Alegre, Brazil) according to the cycle provided by the manufacturer. Before veneering, a bonding agent (Effect Bonder, Vita Zahnfabrik, Bad Sackingen, Germany) was applied on the YZ infrastructures and sintered according to the manufacturer instructions. Three porcelain applications were performed and a polishing bur was used to obtain a uniform thickness of, approximately, 1.2 mm around the crowns and pontic and 0.6 mm around the connectors. The porcelain thickness was measured at six different points as proposed by Sundh et al. (2005) . Finally, all FPDs were subjected to a glaze cycle using Keramat I furnace at 900 °C for 1 min with a rate of 80 °C/min and slow cooling (∼6 min).
The FPDs were cleaned in a sonic bath with distilled water for 15 min and cemented in the metal dies with zinc phosphate cement (Cimento de Zinco, SS White, RJ, Brazil), following the manufacturer’s instructions. A cementation device was used to apply a uniform pressure of 15 N for 10 min in the FPD occlusal surface aiming to guarantee an adequate fit in the dies.
Twenty FPDs with a connector cross-section area of 16 mm 2 (5.0 mm high × 4.2 mm thickness) were produced for each IS material. This connector dimension was chosen based on the manufacturer indication for an IZ FPD with 16 mm span. In addition, for YZ, an extra group of 10 FPDs were built with a connector cross-section area of 9 mm 2 (3.6 mm high × 3.1 mm thickness), following the manufacturer’s recommendation for a YZ FPD with 16 mm 2 span. The experimental groups are described in Table 1 .
Connector | Mechanical cycling | Materials | ||
---|---|---|---|---|
YZ | IZ | AL | ||
9 mm 2 | No | YZ9 | – | – |
16 mm 2 | No | YZ16 | IZ16 | AL16 |
Yes | YZ16-MC | IZ16-MC | AL16-MC |
2.2
Fracture load and fractography
The fracture load of 16 mm 2 connector FPDs was evaluated in two experimental conditions ( n = 10):
- a)
Control : the fracture load was evaluated using a universal testing machine (MTS, MTS Systems Corporation, MN, USA) in 37 °C deionized water. The load was applied in the center of the pontic with a 6 mm diameter stainless steel sphere at 0.5 mm/min until failure.
- b)
Mechanical cycling (MC) : FPDs were subjected to MC using a chewing simulator composed of 10 pneumatic cylinders with a load of 140 N and frequency of 2 Hz for 10 6 cycles in distilled water. Load was applied using a stainless steel sphere with 6 mm diameter. After aging, FPDs were tested until failure as previously described for the control group.
Fracture surfaces were examined using a stereomicroscope to determine the mode of failure based on the fracture origin and fractographic principles . Subsequently, specimens were sputter-coated with gold–palladium and examined using a scanning electron microscope (SEM) to locate and measure the critical flaw dimensions ( c ). The size of a semi-circular flaw, with equal stress intensity factor, was determined using the following Eq. (1) :
c = a b
where a is the crack depth and b is half of the crack width.
Fracture toughness ( K Ic ) was calculated through fractographic analysis of bar-shaped specimens subjected to three-point flexural test. Fracture mechanics was used to estimate the fracture stress ( σ f ) of FPDs, following Griffin–Irwin equation ( Eq. (2) ) :
K Ic = Y σ f c
where Y is a geometric factor, which accounts for the shape and location of the fracture-initiation crack and loading condition, and also depends on the ratio a / b . Y was assumed to be 1.24 for semicircular cracks and 1.4 for corner cracks.
Fracture load, fracture stress and c -values were statistically analyzed by one-way ANOVA and Tukey’s test ( α = 0.05). The relation between the experimental group and failure origin was analyzed using Chi-square test ( α = 0.05).
2.3
Finite element analysis
A SkyScan 1172 micro-CT scanner (Microphotonics, Allentown, PA, USA) was used to produce the FPD models. Two parts were scanned separately: (1) the stainless steel dies simulating the prepared teeth and (2) the three-unit all-ceramic FPD (9 mm 2 and 16 mm 2 connector cross-section).
The scanning parameters were: accelerating voltage of 100 kV, current of 100 μA, exposure time of 2950 ms per frame, Al + Cu filter, and rotation step at 0.4° (180° rotation). For the metal dies, the X-ray beam was irradiated perpendicularly to the preparation long axis, and for the FPDs, the beam was parallel to their long axis. The image pixel size was 6.97 μm. The X-ray projections were reconstructed to form a 3D model, which was saved as a stack of bmp-type files using NRecon software (Microphotonics, Allentown, PA, USA). Beam hardening correction of 80% and ring artifact correction of 7 were used for the reconstruction. Tomography images of the structures were generated at each 0.034 mm and processed by an interactive medical image control system (Mimics 13.0, Materialise, Belgium) .
The micro-CT files corresponding to the metal dies and the FPD were processed separately to reduce the amount of manual work necessary to build three different masks with Mimics software. A mask of the dies was obtained though brightness and contrast adjustment, and a 3D object was produced and converted into a STL file (stereolithography, bilinear and interplane interpolation algorithm). For the FPD, segmentation based on image density thresholding of different value scale intensity levels was used to generate two masks, corresponding to the infrastructure and the veneering layer. 3D objects were obtained for the infrastructure and the veneer. The metal dies STL file was imported to the FPD file, making for a three-part final file: metal dies, infrastructure and veneer.
The masks of each part of the models were separately converted into STL files, which are improper for FEA because of the amount and shape of the triangles. Thus, the files of different parts were incorporated into non-manifold assembly in Mimics, and edited simultaneously. The parts were remeshed with a maximum geometrical error of 0.05%, maintaining the original geometry. Wrapping and smoothing operations were used to remove undesired sharp edges. The mesh quality was defined by controlling triangle edge length, the ratio between height and base of the triangles and removing sharp triangles using a triangle filter, so that the file could be imported into the FEA software without generating errors. Subsequently, the objects were separated according to the original parts and volumetric meshes were generated and imported by the FEA software (ABAQUS V6.8, Simulia, USA).
The final FPD model was composed of tetrahedral elements and three layers: (1) metal dies, (2) ceramic infrastructure and (3) porcelain layer. Two interfaces were created, between the metal dies and the infrastructure and between the infrastructure and the porcelain layer. The cement layer was neglected.
Nodes in the bottom surface of the dies were fixed in all directions; no rotation or translation was allowed. A compressive load, perpendicular to the restoration long axis, was applied in the center of the occlusal surface of the pontic. A load of 200 N was distributed uniformly over 20 nodes located in a circular area with 3 mm diameter.
The following considerations were made: (1) the materials were considered to be isotropic and homogeneous and to have a linear elastic behavior; (2) the effects of the periodontal ligament and pulp chamber were not considered; (3) elastic modulus ( E ) and Poisson’s ratio ( v ) were maintained constant. The values of the material properties used to perform the analysis are presented in Table 2 .
Materials | ||||||
---|---|---|---|---|---|---|
YZ | IZ | AL | VM7 | VM9 | Stainless steel | |
E (GPa) | 209 | 247 | 390 | 67 | 67 | 190 |
ν | 0.32 | 0.26 | 0.24 | 0.23 | 0.21 | 0.27 |
The stress distributions from the linear finite element analyses were evaluated according to the location and magnitude of the maximum first principal stress.
2
Materials and methods
2.1
Specimen fabrication
FPDs were produced using three high crystalline-content infrastructure ceramics veneered with the recommended porcelain, as follows:
-
YZ – An yttria partially stabilized tetragonal zirconia polycrystal (Y-TZP) infrastructure (IS) (Vita In-Ceram YZ, Vita Zahnfabrik, Bad Sackingen, Germany) veneered with a porcelain (Vita VM9, Vita Zahnfabrik, Bad Sackingen, Germany);
-
IZ – A glass infiltrated zirconia-reinforced alumina-based ceramic IS (Vita In-Ceram Zirconia, Vita Zahnfabrik, Bad Sackingen, Germany) veneered with a porcelain (Vita VM7, Vita Zahnfabrik, Bad Sackingen, Germany);
-
AL – An alumina polycrystal IS (Vita In-Ceram AL, Vita Zahnfabrik, Bad Sackingen, Germany) veneered with a porcelain (Vita VM7, Vita Zahnfabrik, Bad Sackingen, Germany).
Stainless steel models simulating prepared abutment teeth were constructed with 4.5 mm height, 6° of taper and 120° chamfer as finish line . The distance between the centers of the dies was 16 mm, corresponding to the distance between a lower second premolar and a lower second molar (span of 10 mm). An artificial gingiva was produced with acrylic resin (JET, Classico, Sao Paulo, SP, Brazil). Polyvinyl siloxane impressions of the model were taken (Aquasil™, Dentsply, Petropolis, RJ, Brazil) and a working cast was made using type IV special CAD/CAM stone (CAM-base, Dentona AG, Dortmund, Germany).
The stone cast was digitized by the internal laser scanner component of CEREC inLab unit (Sirona Dental Systems, Charlote, NC, USA) to generate a tridimensional image that was used to design the FPDs infrastructures from YZ, IZ and AL ceramic systems. After the milling process, YZ and AL infrastructures were sintered using the Zyrcomat furnace (Vita Zahnfabrik, Bad Sackingen, Germany), and IZ infrastructures were glass infiltrated (Z21N Zirconia Glass Powder, Vita Zahnfabrik, Germany) using the Inceramat 3 furnace (Vita Zahnfabrik, Bad Sackingen, Germany), according to the manufacturer’s instruction. The excess glass was removed with airborne particle abrasion using 50-μm aluminum oxide particles. Only the external surface of the infrastructures was air abraded.
The infrastructures were veneered with the recommended porcelain, which were sintered using a Keramat I furnace (Knebel, Porto Alegre, Brazil) according to the cycle provided by the manufacturer. Before veneering, a bonding agent (Effect Bonder, Vita Zahnfabrik, Bad Sackingen, Germany) was applied on the YZ infrastructures and sintered according to the manufacturer instructions. Three porcelain applications were performed and a polishing bur was used to obtain a uniform thickness of, approximately, 1.2 mm around the crowns and pontic and 0.6 mm around the connectors. The porcelain thickness was measured at six different points as proposed by Sundh et al. (2005) . Finally, all FPDs were subjected to a glaze cycle using Keramat I furnace at 900 °C for 1 min with a rate of 80 °C/min and slow cooling (∼6 min).
The FPDs were cleaned in a sonic bath with distilled water for 15 min and cemented in the metal dies with zinc phosphate cement (Cimento de Zinco, SS White, RJ, Brazil), following the manufacturer’s instructions. A cementation device was used to apply a uniform pressure of 15 N for 10 min in the FPD occlusal surface aiming to guarantee an adequate fit in the dies.
Twenty FPDs with a connector cross-section area of 16 mm 2 (5.0 mm high × 4.2 mm thickness) were produced for each IS material. This connector dimension was chosen based on the manufacturer indication for an IZ FPD with 16 mm span. In addition, for YZ, an extra group of 10 FPDs were built with a connector cross-section area of 9 mm 2 (3.6 mm high × 3.1 mm thickness), following the manufacturer’s recommendation for a YZ FPD with 16 mm 2 span. The experimental groups are described in Table 1 .
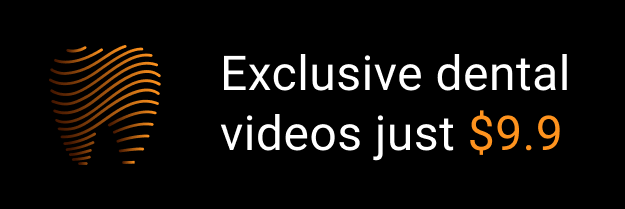