Chapter 9
Digital Workflow in Orthodontics
Guilherme S. Nakagawa, Juliana No-Cortes,Adriano G.B. de Castro, Fernando Barriviera, Maurício Barriviera, and Arthur R.G. Cortes
9.1 CAD‐CAM Guides for Orthodontic Brackets
Indirect bonding is an orthodontic bonding technique in which orthodontic brackets are transferred from working models and bonded onto the teeth surfaces using a transfer silicone tray. This technique includes two stages: the lab stage, where models and trays are manufactured, and the clinical stage, where brackets or appliances are positioned and bonded in the patient’s mouth. Since the majority of the work is done virtually with the software, the placement of orthodontic brackets on the teeth requires less chairside time. The indirect technique has been used for decades, but with digital technology it is even more optimized and precise.
The digital workflow starts with capturing the models as an STL file of the mouth. Using orthodontic software, brackets are virtually positioned on the surface of the teeth. Working with the digital method, DICOM files can be used to determine exactly the axis of the roots and crowns, making it even more complete then the conventional lab method. After the designing step, a custom tray is created which allows the transfer of brackets to the patient’s teeth.
A generic example of CAD‐CAM technology for indirect orthodontic bonding (eXceed™) is shown in Figures 9.1–9.14.
9.2 CAD‐CAM Guides for Orthodontic Miniscrews
Successful treatment of full Class II malocclusion and bimaxillary dentoalveolar protrusion requires efficient anchorage, which can be achieved with several methods, such as headgear, transpalatal arches, and Nance button [1, 2]. However, conventional approaches have been associated with drawbacks such as anchorage loss and mesial migration of posterior dental anchorage units [3]. Furthermore, despite the use of extraoral appliances to enable satisfactory anchorage control [4], such methods are highly affected by patient compliance, leading to variable levels of outcome [5].
An alternative to conventional forms of anchorage is the use of orthodontic mini‐implants and temporary anchorage devices (TAD) [6–8]. In this context, orthodontic mini‐implants allow for direct anchorage by being loaded with reactive forces, and for indirect anchorage, by stabilizing a dental anchorage [9]. Such mini‐implants can be loaded immediately after insertion and are generally removed after treatment completion.
Despite the satisfactory anchorage which can be achieved, orthodontic mini‐implants require precise placement in interradicular spaces with satisfactory bone conditions [10, 11]. For this purpose, a surgical guide can be developed from CBCT scans to decrease the risk of complications such as root damage, penetration into the maxillary sinus, or lack of anchorage due to inadequate mini‐implant position [12]. Advantages of accurate mini‐implant positioning include improved retention during orthodontic loading and precise control of the force vector. Surgical guides for implants can also be developed by means of time‐efficient digital workflows, in which intraoral scans are performed and superimposed to CBCT images, enabling the digital design of a surgical guide [13]. Nevertheless, little is known about using such digital workflow methodology for creating surgical guides to ensure proper positioning of mini‐implants.

Figure 9.1 Initial STL file imported to the software. (a) Frontal view. (b) Lateral view – right side. (c) Lateral view – left side.

Figure 9.2 Initial STL file imported to the software. (a) Occlusal view of the upper arch. (b) Occlusal view of the lower arch.

Figure 9.3 Creation of an orthodontic virtual set‐up simulating the desired final occlusion. (a) Frontal view. (b) Lateral view – right side. (c) Lateral view – left side.

Figure 9.4 After checking and approving the final planning, orthodontic brackets are positioned passively on the arch. Later, this virtual arrangement will be transferred to the initial situation. (a) Frontal view. (b) Lateral view – right side. (c) Lateral view – left side. (d) Occlusal view of the lower arch. (e) Occlusal view of the upper arch.

Figure 9.5 Wire shape templates are created to be printed on paper. The final teeth positioning will follow the virtually planned shape of the arch, leading to an extremely precise, reliable result.

Figure 9.6 Models obtained by 3D printing machine for orthodontic bracket positioning.

Figure 9.7 Double transfer tray for indirect orthodontic bracket bonding – first step: a 1 mm thick sheet of ethylene vinyl acetate (EVA) is used. The main function of this EVA layer is to retain the orthodontic brackets.

Figure 9.8 Double transfer tray for indirect bracket bonding – second step: a 1 mm thick sheet of polyethylene glycol terephthalate (PEGT) applied over the EVA. The main function of this layer is to provide rigidity and stability to the tray.

Figure 9.9 Enamel etching with phosphoric acid for orthodontic bracket bonding. Following this, the dental surfaces should be washed and dried completely and adhesive should be applied to the base of each orthodontic bracket.

Figure 9.10 The double transfer tray is fitted to the upper teeth and the adhesive is light‐cured.

Figure 9.11 The double transfer tray is fitted to the lower teeth and the adhesive is then light‐cured.

Figure 9.12 The PEGT layer of the double transfer tray is removed.

Figure 9.13 The EVA layer of the double transfer tray is then removed.

Figure 9.14 Final clinical aspect: finalization of orthodontic bracket bonding and wires in position.
Thus, the aim of the present report is to describe a full digital workflow involving combination of intraoral and CBCT scans to virtually design and 3D print surgical guides used to ensure mini‐implant placements in optimal locations.
9.2.1 Technique
The process commences by scanning both maxillary and mandibular arches, as well as the occlusion of the patient using an intraoral scanner (TRIOS®, 3Shape). The initial intraoral scans are first imported to a CAD software (OrthoAnalyzer®, 3Shape) as STL files. With this software, orthodontic model bases are digitally applied to all resulting STL files, which can then be 3D‐printed (MoonRay®, Sprintray) and used for orthodontic planning.

VIDEdental - Online dental courses
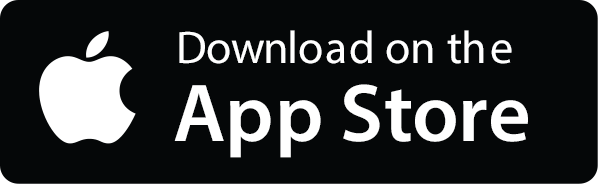
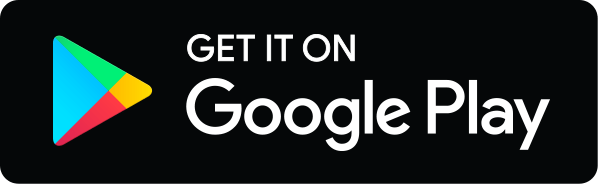