Highlights
- •
BPA can be released in wastewater after grinding of composite materials.
- •
The amount of released BPA is material dependent.
- •
Treatment with activated carbon is effective in wastewater clearance of BPA.
Abstract
Objectives
This study evaluated the release of bisphenol A (BPA) in wastewater after grinding of resin composites and tested three filtration materials.
Methods
Three resin composites (Ceram X, Filtek Supreme XTE and Core-X flow) were used. Samples (5 mm × 2 mm, n = 10) were prepared using a metal mold and were polymerized for 20 s according to manufacturers’ instructions. A dental unit was disconnected from wastewater circulation and composite samples were ground under standardized procedures (200,000 rpm; 90 s). Wastewater was collected in glass bottles. Water samples were collected as control by performing the same procedure without grinding resin composite. All samples were stored at 7 °C for 6 months to simulate storage. Then they were analyzed by HPLC–FLD. Three filtration materials (Zeosorb, Katalox Light and Catalytic Carbon) were used for water treatment to remove BPA. BPA-water solutions were prepared; corresponding to the highest amount released by the resin composites. These solutions were analyzed before and after filtration by HPLC–FLD and their efficacy (%) was calculated.
Results
BPA was detected in all composite solutions: Ceram X and Filtek Supreme XTE showed similar findings ( p > 0.05) which were significantly higher than the control ( p < 0.001) and Core-X flow ( p = 0.001). The efficacy of the filtration materials was: Katalox Light (5.09%) < Zeosorb (7.91%) < Catalytic Carbon (99.38%). Only Catalytic Carbon caused a clinically significant reduction of BPA ( p < 0.05).
Significance
BPA can be released in wastewater during dental procedures. This release seems to be material dependent. Among the filtration methods tested, Catalytic carbon seems to be effective for BPA reduction in water.
1
Introduction
Bisphenol A (BPA) is a chemical substance commonly used in the production of polycarbonate plastics and additionally as part of base molecules, like BisGMA, used for manufacturing of dental resin composites. In the past decades the release of substances from resin composites has been widely evaluated, and BPA is found to be eluted from several dental materials , increasing the concern of possible impact on human health. Several parameters like pH, temperature and time are thought to influence the release of BPA . The difficulty of BPA detection in solutions has resulted in inhomogenous data in the literature due to different methods used for the analysis .
BPA as an endocrine-disrupting chemical binds to and activates the estrogen receptor . It is claimed to be linked to several diseases like breast cancer, heart disease, obesity, cardiovascular problems, infertility and diabetes . It has been found that BPA can influence gene- and cells behavior, by triggering DNA strand breaks and affecting cell division . Although, a lot of data exist concerning the potential effects of BPA on human health, the risk arising from low-dose BPA is still controversial. Due to the increased concern about the potential health risk through BPA exposure, in 2015, the tolerable daily intake (TDI) limit for BPA was reduced from 50 μg/kg of bw/day to 4 μg/kg of bw/day . In 2016, BPA was classified as a category 1B presumed reproductive toxicant , meaning that this substance can adversely affect the human reproductive system. Beside these, BPA was added to the “Candidate List” of substances of very high concern causing adverse effects to the environment by ECHA (European Chemicals Agency) in 2018 . The primary source of BPA exposure to humans is thought to be through diet, as BPA might leach from food containers and water bottles . After oral intake, BPA is absorbed from the gastrointestinal tract and transported to the liver, where it is biotransformed to its conjugated forms . However, toxicokinetic data indicate that the conjunction is not complete and some free BPA remains in the circulation . BPA has been found in human urine samples, human serum, sweat, placental tissue, or ovarian follicular fluid , suggesting that the BPA concentration in the body might be reduced slower than expected based on an originally short BPA-half-life of 5.3 h in humans .
As mentioned above, BPA has been claimed to be released from dental resin composites and sealants , increasing the concern about the biocompatibility of dental restorations. Additionally, recent published data indicate the risk of grinding dental resin composites for human health due to generation of particles that might be inhaled. Cokic et al. showed in a previous work that monomers might be released from the generated particles. Beside the direct impact of the particles and their eluted substances on the dental personal and patients, they can also be released into wastewater through the daily dental grinding procedures.
The daily human intake of BPA next to the increased concern on its effects on human health point out the need of performing a risk assessment concerning the evaluation of the potential release of BPA from dental restorative materials during dental grinding procedures and the implementation of prevention strategies. Therefore, the aim of the present study was to evaluate the release of BPA in wastewater through the dental grinding procedures and to evaluate possible preventive strategies to treat wastewater for BPA reduction. The hypotheses of the study were that (i) BPA is released in wastewater by grinding of resin composites, (ii) the release is material dependent, and (iii) all filtration methods used for cleaning of wastewater can reduce the release of BPA.
2
Materials and methods
2.1
Resin composites used
In the present study, three different resin composites were used: an ormocer (Ceram X®; Dentsply Sirona, Bensheim, Germany), a nanofilled resin composite (Filtek™ Supreme XTE; 3M ESPE, Seefeld, Germany) and a dual-cure core build-up material (Core-X® flow; Dentsply Sirona, Bensheim, Germany). Detailed information about the resin composites is given in Table 1 .
Name/Type/Shade/Batch Number | Manufacturer | Main momomers composition |
---|---|---|
Ceram X®/Ormocer/M2 shade/1209000486 | Dentsply Sirona, Bensheim, Germany | Methacrylate modified polysiloxane, dimethacrylate resin |
Filtek™ Supreme XTE/Nanofilled resin composite/A2 shade/N901475 | 3M GmbH, Seefeld, Germany | BisGMA, BisEMA, TEGDMA, UDMA |
Core X Flow/dual-cure core build-up material/tooth-colored shade/171010 | Dentsply Sirona, Bensheim, Germany | UDMA, di- and tri-functional methacrylates (Urethane modified BisGMA) |
2.2
Preparation of resin composite samples and eluates
A dental unit (KaVo Estetica E70T, KaVo Dental GmbH, Biberach/Riß, Germany) was used in order to obtain the wastewater samples; it was disconnected from the waterflow for the experimental procedures. Resin composite samples (diameter 5 mm × 2 mm depth) were prepared in a metal mold plate. The resin composite material was inserted into the mold in one increment, flattened with a plugger and covered by a matrix strip. The polymerization of the samples was carried out by using a LED light-curing unit (bluephase®, Ivoclar Vivadent AG, Schaan, Lichtenstein) with constant light intensity (950 mW/cm 2 ) according to the manufacturers’ instructions (20 s curing time). Grinding was carried out under standardized conditions, to simulate the clinical procedures in the dental practice. Each resin composite sample was ground for 90 s by using a diamond bur with a standard grit size of 106 μm (Komet, Lemgo, Germany) at 200,000 rpm with a red contra-angle handpiece. To avoid a possible contamination of the water samples with BPA-containing particles from previous experiments, a wash-out phase of the dental unit for 5 min took place between each grinding procedure. The water flow was kept stable, after setting the water flow of the motor at maximum, so that after grinding each sample 750 mL of water were collected in a 1 L brown glass bottle (Carl Roth GmbH + Co. KG, Karlsruhe; Germany), respectively. In addition, 10 negative control samples were prepared, by collecting water samples after performing the same procedure without grinding a composite sample. For this, all the parameters were the same as the ones used for grinding the resin composite samples. The bottles were stored in darkness at 7 °C for 6 months, to simulate a long storage of composite dust in wastewater.
After the storage period, the water bottles were dispersed at 23 °C room temperature in an ultrasonic bath for 20 min. For reliable qualitative and quantitative determination of BPA by HPLC analysis, all water samples were concentrated. Preliminary tests showed that a five-fold concentration of the wastewater samples was necessary for a valuable data analysis; being able to measure BPA concentrations above the quantification limit. This concentration step was performed using solid phase extraction (SPE) with Chromabond® Easy cartridges with polar-modified polystyrene-divinylbenzene matrix (Macherey-Nagel, Düren, Germany). For each single sample a new cartridge was used. The cartridges had to be preconditioned before use. For this step, first, 6 mL of methanol (MeOH) were added to the cartridge followed by rinsing with 6 mL ultrapure H 2 O. After this prewash step, 5 mL of the dispersed water samples were applied to the preconditioned SPE cartridges using a pipette (Eppendorf, Hamburg, Germany). After complete passage of the water samples, the cartridges were dried for 30 min at reduced pressure (0.2 bar). At the end of the drying period, the adsorbed BPA was eluted from the SPE matrix with 4 mL of methanol and collected by applying a slight vacuum (approximately 0.2 bar) in a brown threaded bottle (4 mL). The further procedure took place in a test tube holder. The samples were evaporated in their threaded bottles with nitrogen to complete dryness. To recover the dried powder, 1 mL of pure ethanol (EtOH) was added. Then the bottles were sealed and shaken for 1 min using a vortex mixer (Bender and Hobein GmbH, Freiburg, Germany) followed by retrieving the liquid from the 4 ml threaded bottles in 1 mL HPLC threaded bottles. The finished samples were analysed by HPLC–FLD (high performance liquid chromatography–fluorescence detector). The used method was verified in preliminary studies by using reference BPA samples prepared with the method mentioned above. This testing revealed for the method a good accuracy and a sufficient recovery rate of 87–99%. Between measurements, the samples were stored in darkness at a constant temperature (4 °C).
2.3
Analysis of BPA using HPLC–FLD
The BPA measurements were carried out on a HPLC unit (Shimadzu Deutschland GmbH, Duisburg, Germany). The system consisted of the following components: CBM20A System Controller, Degasser DGU-20a5, two LC20AT pumps, a SIL20AC auto-sampler, a CTO20AC column oven, a M20A diode array detector and a RF10AXL fluorescence detector. The LC Solution software from the same company was used. For BPA analysis, a HPLC column 125/4 Nucleodur 100-5 C18ec (pore size: 100 Å) (Macherey-Nagel, Düren, Germany) was used. The HPLC column and the entire remaining HPLC unit were calibrated once a day according to a fixed procedure. This step was performed using acetonitrile (ACN) and formic acid (0.1%) containing water (H 2 O and CH 2 O 2 ). Both reagents were used additionally as eluents during the measurements. First, a rinse was carried out at a rate of 99:1 (ACN/CH 2 O 2 ) for 30 min, then at 55:45 (ACN/CH 2 O 2 ) for 30 min, and finally at 35:65 (ACN/CH 2 O 2 ) for another 30 min for calibration of the system immediately before the measurements. Detection of BPA was carried out with an Altus A-30 Fluorescence detector (FLD). The excitation wavelength was at 275 nm and the emission wavelength at 313 nm. Using these HPLC measurement parameters, BPA showed a retention time of 7.15 min. For each measurement, 20 μL of the water sample were injected, at a constant flow rate of 0.75 mL/min and a temperature of 35 °C.
For the qualitative and quantitative determination of BPA, a dilution (100 mg/L) of pure BPA powder and pure ethanol (≥99%) was prepared as stock solution. This solution was the first data point for all dilution series. Seven calibration reference standards were determined: 0.005/0.01/0.05/0.1/0.5/0.75 and 1[mg/L] ( Fig. 1 ). Based on the reference standards, the linearity of the chromatographic analysis could be evaluated as shown in Fig. 2 . The detection limit (LOD) was set at 0.001 mg/L and the quantification limit (LOQ) at 0.005 mg/L. The fluorescence diagrams ( Fig. 3 ) were evaluated by the LC Solution Software from Shimadzu. Each measurement result was – despite automatic, software-supported evaluation – re-integrated and controlled manually.



2.4
Filtration materials
For the second part of the study, three different materials were used for filtration of BPA-containing water samples: Zeosorb, Katalox Light and Catalytic Carbon (Watch GmbH, Mannheim, Germany) ( Fig. 3 ). The materials differ in particle size, matrix, kind of reactive surface and separation process. Detailed properties and information about the three filtration materials are given in Table 2 .
Zeosorb | Katalox light | Catalytic carbon | |
---|---|---|---|
Manufacturer | SZ Water Treatment System GmbH (Bubenheim, Germany) | ||
Batch number | Not given | KL2013456789 | 3-44-15-0008 |
Filtering matrix | Alumino-silicate and green sand | MnO 2 coated Zeosorb + Calcium hydroxide | Activated carbon + catalyst FeOH |
Surface structure | Microporous, crystalline(Hydrophilic) | Microporous, crystalline (Hydrophilic) | Microporous (Hydrophilic) |
Color | Green/gray | Gray/black | Black |
Density | 820 kg/m 3 | 1060 kg/m 3 | 630–640 kg/m 3 |
Reaction surface | <270 m 2 /g | Not given | 2000–2500 m 2 /g |
Target substances | Ammonium, nitrogen, heavy metals, radionuclides, dyes, microorganisms | Hydrogen sulfide, heavy metals, radionuclides (e.g., radium, uranium) | Hydrogen sulphide, heavy metals, tannins, phenols, chloramines, trihalomethanes, fragrances and dyes |
Flow rate | ≤10 m/h | ≤20 m/h | Not given |
Separation process | Filtration | Filtration | Adsorption |
Surface charge | Negative | Negative | Positive |
Particle filtration up to | <3 μm | <3 μm | ≤1 μm |
Particle size | 50–150 μm | 60–140 μm | 60–240 μm |
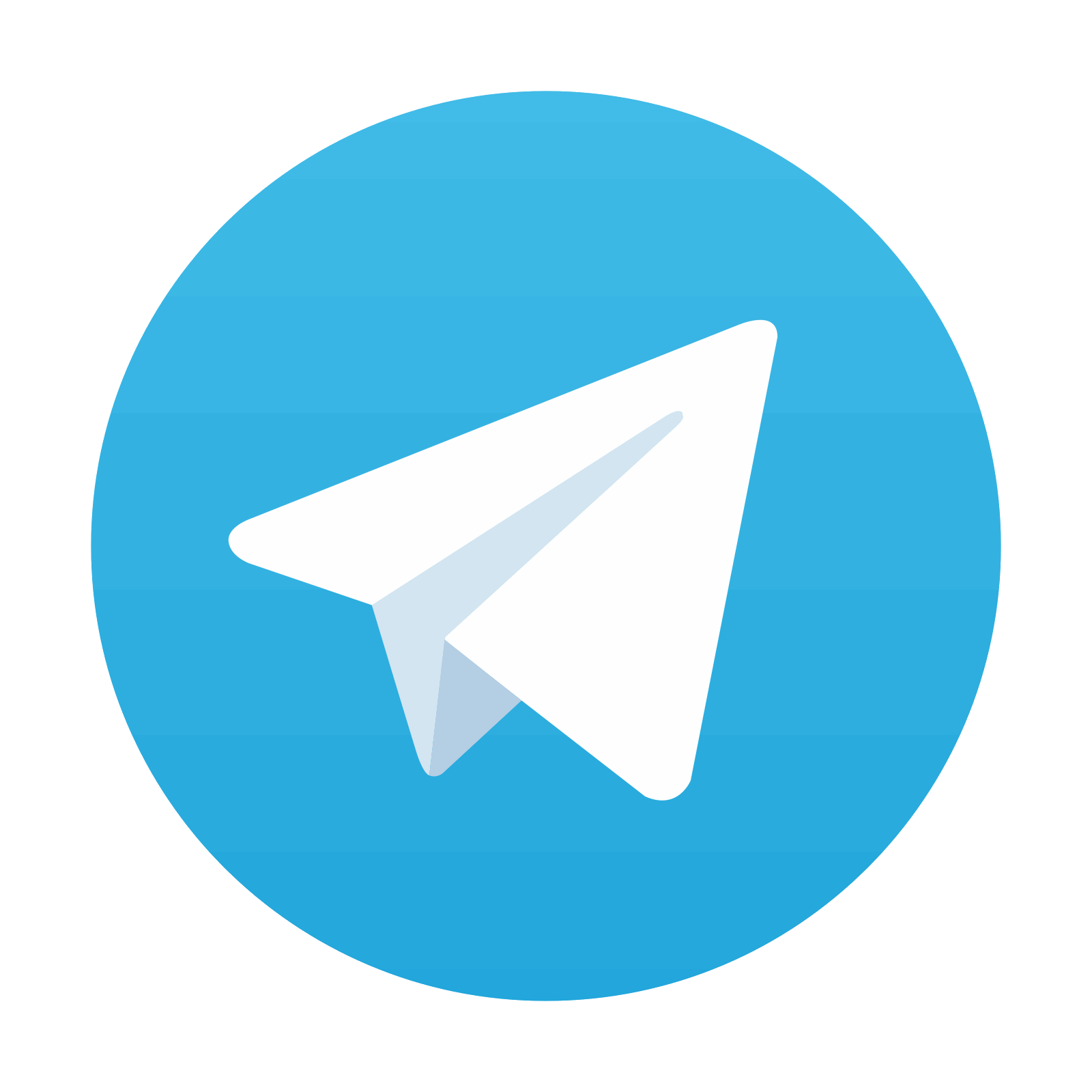
Stay updated, free dental videos. Join our Telegram channel

VIDEdental - Online dental courses
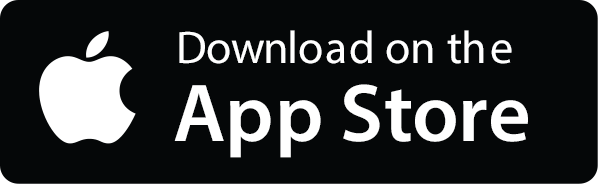
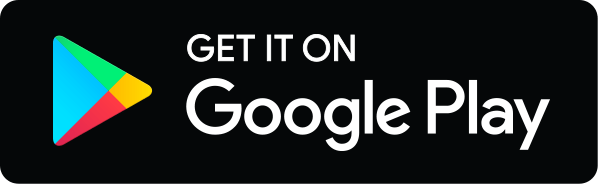
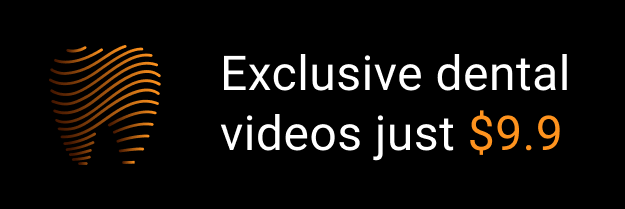