Abstract
Objective
Caries adjacent to restorations is one of the main causes for restoration replacement. Antimicrobial substances incorporated into dental materials would potentially be able to reduce secondary caries initiation and progression. This study investigated biofilm growth of Streptococcus mutans UA159 on the surface of composite materials containing the biomolecule carolacton compared to materials containing chlorhexidine (CHX) and triclosan.
Methods
Biofilm inhibition was investigated by counting colony forming units (CFU), viability staining (Life/Dead), and real-time quantitative PCR.
Results
First, the antimicrobial substances were added to the cultivation medium at 2.5 μg/ml (0.0002%) and 0.25 μg/ml (0.00002%). CHX eliminated bacterial growth and biofilm formation completely. Triclosan was effective at 2.5 μg/ml, but at 0.25 μg/ml biofilm mass and viability were unchanged, yet the number of CFU increased due to disruption of cell chains and biofilm aggregates. Carolacton had a limited effect on biofilm growth and mass, but reduced viability significantly. When incorporated into composite materials carolacton (25 μg/ml resp. 0.002%, w/w) had no adverse effect on physical/mechanical properties and retained its biofilm inhibiting effect. Life/Dead staining revealed a reduction of biofilm viability of up to 64%. CFUs were reduced by 98% and qPCR demonstrated a mean inhibition of 87%. In contrast, materials containing CHX or triclosan showed an insignificant effect on biofilm formation, even at a 100fold increased concentration (0.2%). The anti-biofilm activity of composite material containing carolacton was stable over a period of 42 days.
Significance
Carolacton incorporated into dental filling material has a strong biofilm-inhibiting effect on S. mutans and is therefore potentially able to prevent secondary caries formation.
1
Introduction
Caries adjacent to dental materials is a major clinical problem in dentistry. Micro-spaces between filling materials and dental hard tissues or areas around orthodontic brackets, where tooth brushing is difficult, favor biofilm accumulation and demineralization of the tooth enamel. The prevalence of white spot lesions and demineralization after orthodontic treatment is reported to be up to 84% . Secondary caries adjacent to dental restorations accounts for up to 55% of failures of fillings and is therefore one of the main causes for restoration replacement .
Biofilm forming bacteria are essential in the etiology of dental caries. There seems to be no difference in the composition of the biofilm comparing primary and secondary caries . However, it has been reported that the properties of the dental materials influence plaque accumulation and development of secondary caries . Composite materials, nowadays predominantly used in dentistry, display a higher adhesion of bacteria than other dental materials .
Among the different bacterial species, streptococci are considered to play a major role in plaque biofilm formation and development. Streptococcus mitis , Streptococcus sanguinis , and Streptococcus gordonii are regarded as early colonizing bacteria while Streptococcus mutans is considered to be involved in later stages and with the highest cariogenic potential .
Considering the previously mentioned facts it is not surprising that the development of dental materials with an antibacterial activity has been the focus of dental research for quite some time. To this end, several antimicrobials have been incorporated in restorative materials and bonding systems. For instance a polymerizable bactericidal monomer, 12-metacryloyloxydodecylpyridinium bromide (MDPB) was immobilized in a resin which reduced bacterial growth . Other studies incorporated chlorhexidine (CHX) or silver ions (Ag + ) in different formulations . So far there is no evidence that these antibacterial agents are effective in improving clinical outcomes .
Only recently, the antibacterial activity of carolacton against S. mutans biofilms was described . Carolacton is a secondary metabolite from the myxobacterium Sorangium cellulosum and acts by interfering with PknB-mediated signaling in growing S. mutans cells. The resulting dramatically altered cell wall morphology causes membrane damage and cell death at low pH . Carolacton is a small molecule which is active already at 10 nMol. The advantage of incorporating it into dental filling material would be that only very small concentrations might be needed which are unlikely to affect the mechanical properties of the composite.
Here we describe for the first time the incorporation of carolacton into dental composite materials and the resulting activity against S. mutans biofilms in vitro .
2
Materials and methods
2.1
Composite materials
2.1.1
Preparation of composite materials
Preparation of composite resins was done according to standard procedures. The monomers and co-monomers, together with the photopolymerization system, were homogenized. The pyogenic silica Aerosil was added by using a mixer (TGC8AWDS-102, PC Laborsysteme, Magden, Switzerland). For filler loading, the respective particles were added in batches and the resin paste was mixed for 1 h until it was homogenous. To disperse all filler particles the mixture was milled with a three-roll mill (EXAKT 80 E, Norderstedt, Germany). Thereafter, the paste was dispersed in low vacuum (0.8 bar) for 10 min to remove air bubbles ( Table 1 ).
Contents | V47188-1 | V47188-2 | V47188-3 |
---|---|---|---|
Bis-GMA | 41% | 41% | 32% |
TEDMA | 21% | 21% | 35.5% |
HEDMA | 38% | 38% | – |
UDMA | – | – | 32% |
Campherchinon (CQ) | 0.1% | 0.1% | 0.2% |
P-dimethylaminobenzoic acid ethyl ester (DABE) | 0.15% | 0.15% | 0.3% |
Resin materials total | 20% | 22% | 22% |
Nano-SiO 2 particles, silanized | 9% | – | – |
Glass-ceramics, silanized, D50 = 4 μm | – | 53.4% | 53.4% |
Glass-ceramics, silanized, D50 = 2 μm | 58% | – | – |
Glass-ceramics, silanized, D50 = 0.7 μm | 13% | 13.8% | 13.8% |
Pyrogenic silica, silanized | – | 10.8% | 10.8% |
Resulting characteristics | Nano-filled material, small particle size | Agglomerated nanofillers, large particles | Agglomerated nanofillers, large particles |
2.2
Physical and mechanical properties of composite materials
2.2.1
Flexural strength and elastic modulus
For the composite materials, the mechanical properties were measured according to ISO 4049:2000 Dentistry-Polymer based restorative materials . In brief: for flexural strength and elastic modulus measurement rectangular bar samples (25 mm × 2 mm × 2 mm) were prepared in a teflon mould between two glass slides and cured for 20 s with overlapping irradiation zones from both sides using a commercial dental polymerization unit (Celalux II, VOCO Cuxhaven, Germany). The samples were then stored in distilled water at 37 °C for 1 h. A group of n = 10 samples each was tested for flexural strength and elastic of modulus. The thickness and width of the samples were measured using a digital micrometer (Mitutoyo 293-821, Neuss, Germany) with an accuracy of 0.01 mm at three locations along the rectangular bar samples. The mean value was used for calculations. A three-point bending test was carried out using a universal testing machine (Zwick Z010 TN, Ulm, Germany). The crosshead speed was set at 1 mm/min. The apparatus consisted of two rods, mounted parallel with the distance of 20 mm between their centers. The maximum load supported by the sample prior to failure and the load-deformation profile was recorded electronically. Flexural strength in megapascals (MPa), was calculated using the formula σ = 3 Pl /2 bd 2 . Where P is the maximum load at the point of fracture (Newton), l is the distance between the supports (20 mm), b is the width of sample (mm), and d is the thickness of the sample (mm) measured prior to testing.
The elastic modulus in megapascals (MPa) was determined from the slope of the stress strain curve generated from the three-point bending test results by using the equation: E = (Δ F /Δ Y ) × (l3/4 bd 3 ). Where Δ F /Δ Y is the change in force (Δ F ) per unit change in deflection of the center of the sample (Δ Y ), l is the distance between the supports on the tension surface (20 mm), b is the width of the sample, and d is the mean thickness of the sample between the tension and compression surfaces.
2.2.2
Water sorption and water solubility
Specimen discs were prepared in a split ring mould with internal dimensions of 15 mm in diameter and 1 mm depth between two glass slides and were cured from both sides as described before. Thereafter the moulds were transferred to an oven and maintained at 37 °C for 15 min. The specimens were forwarded to a two-step desiccation process. In the first desiccator the samples were maintained at 37 °C for 22 h and thereafter stored in a second desiccator at 23 °C for 2 h. Samples were then weigh with an analytic balance (Sartorius, Cubis MSE324S-1CE-DU, Göttingen, Germany) to an accuracy of 0.1 mg. This cycle was repeated until a constant mass ( m 1 ), was obtained.
Next, the specimens were immersed in water at 37 °C for 7 d. After blotting away surface water and waving the samples in the air for 15 s, the weight was recorded ( m 2 ). After this weighing, recondition the specimens to constant mass were done in the desiccators using the cycle described before. Finally, constant mass ( m 3 ) was measured.
Calculation of water sorption, W sp , in micrograms per cubic millimeter (μg/mm 2 ), for each of the five specimens was done according the equation: W sp = ( m 2 − m 3 )/ V . Where V is the volume of the specimen in cubic millimeters.
Solubility W sl in microgram per cubic millimeter was calculated using the equation: W sl = ( m 1 − m 3 )/ V .
Mean vales for n = 5 samples of each material were calculated.
2.3
Antibacterial agents
Carolacton, derived from a bioreactor fermentation of S. cellulosum , was dissolved in methanol at a concentration of 1 mg/ml. Based on this stock solution, different dilutions in methanol were made with final methanol concentrations in media not exceeding 2.5% (vol/vol).
Chlorhexidine diacetate and triclosan were purchased from Sigma–Aldrich (Taufkirchen, Germany). Both antibacterial agents were treated like carolacton using stock solutions of 1 mg/ml. For the experiment with 2500 μg/ml a stock solution of 0.1 g/ml was used.
2.4
Microbiology
2.4.1
Strain, media, and growth conditions
S. mutans strain UA159 (ATCC 700610) was grown routinely in Todd-Hewitt broth (THB) (Becton Dickinson, Heidelberg, Germany). For biofilm growth, 0.5% sucrose (Sigma, Taufkirchen, Germany) was added to THB (THBS). All media were degassed by flushing with nitrogen, and experiments were carried out at 37 °C in an anaerobic chamber (Don Whitley Scientific, Shipley, England) which provided an atmosphere of 80% N 2 , 10% H 2 , and 10% CO 2 .
2.4.2
Biofilm cultivation
Biofilm formation was induced as follows: Overnight cultures of S. mutans UA159 were diluted to an OD 600 of 0.01–0.03 in fresh THBS. Aliquots (200 μl) were pipetted into all wells of the microtiter plate containing test samples or controls (test wells containing composite material with antimicrobial substances, controls with agent-free composites, controls without any composite). The microtiter plates were incubated at 37 °C without shaking under anaerobic conditions for 20 h. For every biofilm quantification technique (see below) separate plates were prepared.
2.4.3
Determination of optical density (OD 600 )
The optical density of bacterial cultures was measured at λ = 600 nm using a Ultraspec 3100pro UV/Visible spectrophotometer (Biochrom Ltd., Cambridge, England). The optical density of biofilm cultures in microtiter plates was determined in a microtiter plate reader (Wallac Victor3™1420 Multilabel Counter, Perkin-Elmer Life Sciences) after 20 h of incubation before the planktonic bacteria in the supernatant were removed. It is thus a rough estimate of growth of both planktonic and attached bacteria.
2.4.4
Determination of cell viability by counting colony forming units (CFU)
After discarding the supernatant and washing the attached biofilm with 100 μl of 0.85% NaCl, the biofilm was harvested by adding a small volume of PBS and gently scraping it off the bottom of the well with the tip of the pipette. This procedure was repeated several times and the biofilms from 3 wells were pooled in a final volume of 1 ml PBS. Samples were serially diluted in 0.85% NaCl, and 50 μl of up to five appropriate dilutions were plated onto Todd-Hewitt agar plates in triplicate and incubated anaerobically at 37 °C for 2 days before counting colony-forming units (CFU).
2.4.5
LIVE/DEAD BacLight bacterial viability staining
In addition, biofilms were analysed using the LIVE/DEAD BacLight bacterial viability staining kit L13152 (Invitrogen, Molecular Probes, Inc., Eugene, OR, USA) according to the manufacturer’s instructions. The kit consists of two stains, propidium iodide and SYTO9, which both stain nucleic acids. When used alone, green fluorescent SYTO9 generally labels all bacteria in a population, whereas red fluorescent propidium iodide only penetrates bacteria with damaged membranes, causing a reduction in the green SYTO9 stain fluorescence. Thus, with an appropriate mixture of the SYTO9 and propidium iodide stains, bacteria with intact membranes stain fluorescent green, while bacteria with damaged membranes stain fluorescent red. Staining of biofilms was usually carried out for 15 min in the dark at room temperature with 100 μl of a 1:1 mixture of the two dye components. To remove planktonic and loosely bound bacteria the biofilms were carefully washed before staining with 100 μl of 0.85% NaCl. Fluorescence was measured in a microtiter plate reader (Wallac Victor3™1420 Multilabel Counter, Perkin-Elmer Life Sciences) equipped with detectors and filter sets for monitoring red (630 nm) and green (535 nm) fluorescence. Inhibition of biofilm viability was calculated as reduction of the ratio of green/red fluorescence compared to untreated controls.
2.4.6
DNA extraction and real-time-quantitative qPCR
The biofilm attached to test-composites, control-composites and of wells without any composite was harvested as described above and the DNA was extracted with a Qiamp DNA Mini Kit (‘tissue protocol’, Qiagen, Hilden, Germany) according to the manufacturer’s instructions. Selective amplification and detection of S. mutans DNA by qPCR directed against the virulence factor genes gtf B and gtf C (encoding the glucosyltransferases-I and -SI) was performed on a Light Cycler 2.0 (Roche Applied Science, Penzberg, Germany) using LightCycler ® FastStart DNA Masterplus SYBR Green I in a total volume of 20 μl. Final reactions contained 100 nM of each primer, and 2 μl of template DNA (approximately 75 ng). The primers used were: SmF5 5′-AGCCATGCGCAATCAACAGGTT-3′ (forward primer), SmR4 5′-CGCAACGCGAACATCTTGATTAG-3′ (reverse primer) . Conditions for this PCR were: 95 °C, 10 min; followed by 50 cycles of 95 °C for 10 s; annealing temperature 68 °C for 15 s and 72 °C for 20 s; melting curve analysis for confirmation was run subsequently. The length of the gtf amplicon was 415 bp. Data acquisition and subsequent analysis was performed using LightCycler ® Software 3.5 (Roche Applied Science). The DNA preparation was done in duplicate and the qPCR was run in triplicate (resulting in six sets of data, Table 4 ). Melting curve analysis was performed to determine the melting point of the amplification products and to assess reaction specificity. To avoid any possible primer dimer interference, the temperature at which the fluorescence was read during each cycle was adjusted to one degree just below the melting point of the amplification product. Gel electrophoresis on a 1% agarose gel was performed to confirm the correct fragment size of the generated PCR amplicons (415 bp). The amount of initial target DNA was calculated by determining the crossing point (Cp), the cycle at which the fluorescence exceeds a threshold value significantly higher than the background fluorescence. Quantification was performed using the automated (default) algorithm, a strategy that calculates the crossing point as the first maximum of the second derivative of the amplification curve. The conversion of crossing points to initial gene target molecule numbers was based on dilution series of target DNA with defined target molecule amounts. Per S. mutans cell two ( gtf B and gtfC ) glucosyltransferase-genes are amplified by the PCR which was considered when calculating corresponding cell numbers.
2.5
Test of antimicrobial compounds added to the biofilm cultivation medium
First we tested the effect of the antimicrobial compounds on biofilm growth in standard microtiter culture dishes. The three compounds were added in final concentrations of 0.25 and 2.5 μg/ml (corresponding to 0.00002% and 0.0002%, w/w) to 200 μl of standardized S. mutans culture as described previously . After 20 h biofilm growth, OD 600 , CFU and fluorescence after Live/Dead staining were measured as described before. Every experiment was then completely repeated using a second plate.
2.6
Test of composite material containing antimicrobial compounds
Next, carolacton was incorporated into composite material during preparation. For this purpose, an aliquot of the stock solution (1 mg/ml) was added to the composite material and homogenized resulting in a final concentration of 25 μg/ml (0.002%, w/w). As controls chlorhexidine diacetate (CHX) or triclosan were incorporated into the composite material at concentrations of both 25 μg/ml and 2500 μg/ml (0.2%, w/w) respectively. The methanol used as dissolving agent was totally evaporated in the final vacuum preparation step.
The experimental composite materials were cured for 20 s by a commercial dental polymerization unit (Celalux II, VOCO Cuxhaven, Germany) from both sides. Thereafter the materials were prepared to fit into 96 well microtiter plates. The samples were fixed with a little portion of the uncured material, which was then polymerized for 20 s. The samples formed 1 mm thin disks on the bottom of the microtiter wells (diameter of 4.26 mm). All wells were cleaned and disinfected by 70% alcohol before use.
Each material containing one of the antimicrobial compounds was tested in 10 replicates (10 wells). Wells containing the same composite material without any antimicrobial compound and wells containing no composite material served as controls.
Because the allocation of the microtiter plates and the following microbiological experiments were done at different locations and by different persons, the test set-up was single-blinded.
2.7
Test for anti-biofilm activity of carolacton containing composite material over time
By analysing a time series of samples we conducted two experiments to test if the biofilm inhibitory effect of composite material containing carolacton is stable over time. To this end, the wells of a microtiter plate with V47188-1-carolacton (0.002%) composites (1 mm thickness) were covered with sterile phosphate-buffered saline (PBS) and stored at 4 °C for 19 (experiment 1) and 42 days (experiment 2), respectively. At the time point for testing, the supernatant was removed for a subset of samples, and biofilm formation and inhibition was determined on those same samples by Live/Dead staining as described. A potential biofilm inhibitory effect of the supernatant was also tested. In experiment 1 which lasted 19 days, samples were analysed on days 1, 7 and 19. Since little decline in anti-biofilm activity was observed, the experimental period was extended to 42 days in experiment 2, and the sampling days were days 1, 19, and 42.
2
Materials and methods
2.1
Composite materials
2.1.1
Preparation of composite materials
Preparation of composite resins was done according to standard procedures. The monomers and co-monomers, together with the photopolymerization system, were homogenized. The pyogenic silica Aerosil was added by using a mixer (TGC8AWDS-102, PC Laborsysteme, Magden, Switzerland). For filler loading, the respective particles were added in batches and the resin paste was mixed for 1 h until it was homogenous. To disperse all filler particles the mixture was milled with a three-roll mill (EXAKT 80 E, Norderstedt, Germany). Thereafter, the paste was dispersed in low vacuum (0.8 bar) for 10 min to remove air bubbles ( Table 1 ).
Contents | V47188-1 | V47188-2 | V47188-3 |
---|---|---|---|
Bis-GMA | 41% | 41% | 32% |
TEDMA | 21% | 21% | 35.5% |
HEDMA | 38% | 38% | – |
UDMA | – | – | 32% |
Campherchinon (CQ) | 0.1% | 0.1% | 0.2% |
P-dimethylaminobenzoic acid ethyl ester (DABE) | 0.15% | 0.15% | 0.3% |
Resin materials total | 20% | 22% | 22% |
Nano-SiO 2 particles, silanized | 9% | – | – |
Glass-ceramics, silanized, D50 = 4 μm | – | 53.4% | 53.4% |
Glass-ceramics, silanized, D50 = 2 μm | 58% | – | – |
Glass-ceramics, silanized, D50 = 0.7 μm | 13% | 13.8% | 13.8% |
Pyrogenic silica, silanized | – | 10.8% | 10.8% |
Resulting characteristics | Nano-filled material, small particle size | Agglomerated nanofillers, large particles | Agglomerated nanofillers, large particles |
2.2
Physical and mechanical properties of composite materials
2.2.1
Flexural strength and elastic modulus
For the composite materials, the mechanical properties were measured according to ISO 4049:2000 Dentistry-Polymer based restorative materials . In brief: for flexural strength and elastic modulus measurement rectangular bar samples (25 mm × 2 mm × 2 mm) were prepared in a teflon mould between two glass slides and cured for 20 s with overlapping irradiation zones from both sides using a commercial dental polymerization unit (Celalux II, VOCO Cuxhaven, Germany). The samples were then stored in distilled water at 37 °C for 1 h. A group of n = 10 samples each was tested for flexural strength and elastic of modulus. The thickness and width of the samples were measured using a digital micrometer (Mitutoyo 293-821, Neuss, Germany) with an accuracy of 0.01 mm at three locations along the rectangular bar samples. The mean value was used for calculations. A three-point bending test was carried out using a universal testing machine (Zwick Z010 TN, Ulm, Germany). The crosshead speed was set at 1 mm/min. The apparatus consisted of two rods, mounted parallel with the distance of 20 mm between their centers. The maximum load supported by the sample prior to failure and the load-deformation profile was recorded electronically. Flexural strength in megapascals (MPa), was calculated using the formula σ = 3 Pl /2 bd 2 . Where P is the maximum load at the point of fracture (Newton), l is the distance between the supports (20 mm), b is the width of sample (mm), and d is the thickness of the sample (mm) measured prior to testing.
The elastic modulus in megapascals (MPa) was determined from the slope of the stress strain curve generated from the three-point bending test results by using the equation: E = (Δ F /Δ Y ) × (l3/4 bd 3 ). Where Δ F /Δ Y is the change in force (Δ F ) per unit change in deflection of the center of the sample (Δ Y ), l is the distance between the supports on the tension surface (20 mm), b is the width of the sample, and d is the mean thickness of the sample between the tension and compression surfaces.
2.2.2
Water sorption and water solubility
Specimen discs were prepared in a split ring mould with internal dimensions of 15 mm in diameter and 1 mm depth between two glass slides and were cured from both sides as described before. Thereafter the moulds were transferred to an oven and maintained at 37 °C for 15 min. The specimens were forwarded to a two-step desiccation process. In the first desiccator the samples were maintained at 37 °C for 22 h and thereafter stored in a second desiccator at 23 °C for 2 h. Samples were then weigh with an analytic balance (Sartorius, Cubis MSE324S-1CE-DU, Göttingen, Germany) to an accuracy of 0.1 mg. This cycle was repeated until a constant mass ( m 1 ), was obtained.
Next, the specimens were immersed in water at 37 °C for 7 d. After blotting away surface water and waving the samples in the air for 15 s, the weight was recorded ( m 2 ). After this weighing, recondition the specimens to constant mass were done in the desiccators using the cycle described before. Finally, constant mass ( m 3 ) was measured.
Calculation of water sorption, W sp , in micrograms per cubic millimeter (μg/mm 2 ), for each of the five specimens was done according the equation: W sp = ( m 2 − m 3 )/ V . Where V is the volume of the specimen in cubic millimeters.
Solubility W sl in microgram per cubic millimeter was calculated using the equation: W sl = ( m 1 − m 3 )/ V .
Mean vales for n = 5 samples of each material were calculated.
2.3
Antibacterial agents
Carolacton, derived from a bioreactor fermentation of S. cellulosum , was dissolved in methanol at a concentration of 1 mg/ml. Based on this stock solution, different dilutions in methanol were made with final methanol concentrations in media not exceeding 2.5% (vol/vol).
Chlorhexidine diacetate and triclosan were purchased from Sigma–Aldrich (Taufkirchen, Germany). Both antibacterial agents were treated like carolacton using stock solutions of 1 mg/ml. For the experiment with 2500 μg/ml a stock solution of 0.1 g/ml was used.
2.4
Microbiology
2.4.1
Strain, media, and growth conditions
S. mutans strain UA159 (ATCC 700610) was grown routinely in Todd-Hewitt broth (THB) (Becton Dickinson, Heidelberg, Germany). For biofilm growth, 0.5% sucrose (Sigma, Taufkirchen, Germany) was added to THB (THBS). All media were degassed by flushing with nitrogen, and experiments were carried out at 37 °C in an anaerobic chamber (Don Whitley Scientific, Shipley, England) which provided an atmosphere of 80% N 2 , 10% H 2 , and 10% CO 2 .
2.4.2
Biofilm cultivation
Biofilm formation was induced as follows: Overnight cultures of S. mutans UA159 were diluted to an OD 600 of 0.01–0.03 in fresh THBS. Aliquots (200 μl) were pipetted into all wells of the microtiter plate containing test samples or controls (test wells containing composite material with antimicrobial substances, controls with agent-free composites, controls without any composite). The microtiter plates were incubated at 37 °C without shaking under anaerobic conditions for 20 h. For every biofilm quantification technique (see below) separate plates were prepared.
2.4.3
Determination of optical density (OD 600 )
The optical density of bacterial cultures was measured at λ = 600 nm using a Ultraspec 3100pro UV/Visible spectrophotometer (Biochrom Ltd., Cambridge, England). The optical density of biofilm cultures in microtiter plates was determined in a microtiter plate reader (Wallac Victor3™1420 Multilabel Counter, Perkin-Elmer Life Sciences) after 20 h of incubation before the planktonic bacteria in the supernatant were removed. It is thus a rough estimate of growth of both planktonic and attached bacteria.
2.4.4
Determination of cell viability by counting colony forming units (CFU)
After discarding the supernatant and washing the attached biofilm with 100 μl of 0.85% NaCl, the biofilm was harvested by adding a small volume of PBS and gently scraping it off the bottom of the well with the tip of the pipette. This procedure was repeated several times and the biofilms from 3 wells were pooled in a final volume of 1 ml PBS. Samples were serially diluted in 0.85% NaCl, and 50 μl of up to five appropriate dilutions were plated onto Todd-Hewitt agar plates in triplicate and incubated anaerobically at 37 °C for 2 days before counting colony-forming units (CFU).
2.4.5
LIVE/DEAD BacLight bacterial viability staining
In addition, biofilms were analysed using the LIVE/DEAD BacLight bacterial viability staining kit L13152 (Invitrogen, Molecular Probes, Inc., Eugene, OR, USA) according to the manufacturer’s instructions. The kit consists of two stains, propidium iodide and SYTO9, which both stain nucleic acids. When used alone, green fluorescent SYTO9 generally labels all bacteria in a population, whereas red fluorescent propidium iodide only penetrates bacteria with damaged membranes, causing a reduction in the green SYTO9 stain fluorescence. Thus, with an appropriate mixture of the SYTO9 and propidium iodide stains, bacteria with intact membranes stain fluorescent green, while bacteria with damaged membranes stain fluorescent red. Staining of biofilms was usually carried out for 15 min in the dark at room temperature with 100 μl of a 1:1 mixture of the two dye components. To remove planktonic and loosely bound bacteria the biofilms were carefully washed before staining with 100 μl of 0.85% NaCl. Fluorescence was measured in a microtiter plate reader (Wallac Victor3™1420 Multilabel Counter, Perkin-Elmer Life Sciences) equipped with detectors and filter sets for monitoring red (630 nm) and green (535 nm) fluorescence. Inhibition of biofilm viability was calculated as reduction of the ratio of green/red fluorescence compared to untreated controls.
2.4.6
DNA extraction and real-time-quantitative qPCR
The biofilm attached to test-composites, control-composites and of wells without any composite was harvested as described above and the DNA was extracted with a Qiamp DNA Mini Kit (‘tissue protocol’, Qiagen, Hilden, Germany) according to the manufacturer’s instructions. Selective amplification and detection of S. mutans DNA by qPCR directed against the virulence factor genes gtf B and gtf C (encoding the glucosyltransferases-I and -SI) was performed on a Light Cycler 2.0 (Roche Applied Science, Penzberg, Germany) using LightCycler ® FastStart DNA Masterplus SYBR Green I in a total volume of 20 μl. Final reactions contained 100 nM of each primer, and 2 μl of template DNA (approximately 75 ng). The primers used were: SmF5 5′-AGCCATGCGCAATCAACAGGTT-3′ (forward primer), SmR4 5′-CGCAACGCGAACATCTTGATTAG-3′ (reverse primer) . Conditions for this PCR were: 95 °C, 10 min; followed by 50 cycles of 95 °C for 10 s; annealing temperature 68 °C for 15 s and 72 °C for 20 s; melting curve analysis for confirmation was run subsequently. The length of the gtf amplicon was 415 bp. Data acquisition and subsequent analysis was performed using LightCycler ® Software 3.5 (Roche Applied Science). The DNA preparation was done in duplicate and the qPCR was run in triplicate (resulting in six sets of data, Table 4 ). Melting curve analysis was performed to determine the melting point of the amplification products and to assess reaction specificity. To avoid any possible primer dimer interference, the temperature at which the fluorescence was read during each cycle was adjusted to one degree just below the melting point of the amplification product. Gel electrophoresis on a 1% agarose gel was performed to confirm the correct fragment size of the generated PCR amplicons (415 bp). The amount of initial target DNA was calculated by determining the crossing point (Cp), the cycle at which the fluorescence exceeds a threshold value significantly higher than the background fluorescence. Quantification was performed using the automated (default) algorithm, a strategy that calculates the crossing point as the first maximum of the second derivative of the amplification curve. The conversion of crossing points to initial gene target molecule numbers was based on dilution series of target DNA with defined target molecule amounts. Per S. mutans cell two ( gtf B and gtfC ) glucosyltransferase-genes are amplified by the PCR which was considered when calculating corresponding cell numbers.
2.5
Test of antimicrobial compounds added to the biofilm cultivation medium
First we tested the effect of the antimicrobial compounds on biofilm growth in standard microtiter culture dishes. The three compounds were added in final concentrations of 0.25 and 2.5 μg/ml (corresponding to 0.00002% and 0.0002%, w/w) to 200 μl of standardized S. mutans culture as described previously . After 20 h biofilm growth, OD 600 , CFU and fluorescence after Live/Dead staining were measured as described before. Every experiment was then completely repeated using a second plate.
2.6
Test of composite material containing antimicrobial compounds
Next, carolacton was incorporated into composite material during preparation. For this purpose, an aliquot of the stock solution (1 mg/ml) was added to the composite material and homogenized resulting in a final concentration of 25 μg/ml (0.002%, w/w). As controls chlorhexidine diacetate (CHX) or triclosan were incorporated into the composite material at concentrations of both 25 μg/ml and 2500 μg/ml (0.2%, w/w) respectively. The methanol used as dissolving agent was totally evaporated in the final vacuum preparation step.
The experimental composite materials were cured for 20 s by a commercial dental polymerization unit (Celalux II, VOCO Cuxhaven, Germany) from both sides. Thereafter the materials were prepared to fit into 96 well microtiter plates. The samples were fixed with a little portion of the uncured material, which was then polymerized for 20 s. The samples formed 1 mm thin disks on the bottom of the microtiter wells (diameter of 4.26 mm). All wells were cleaned and disinfected by 70% alcohol before use.
Each material containing one of the antimicrobial compounds was tested in 10 replicates (10 wells). Wells containing the same composite material without any antimicrobial compound and wells containing no composite material served as controls.
Because the allocation of the microtiter plates and the following microbiological experiments were done at different locations and by different persons, the test set-up was single-blinded.
2.7
Test for anti-biofilm activity of carolacton containing composite material over time
By analysing a time series of samples we conducted two experiments to test if the biofilm inhibitory effect of composite material containing carolacton is stable over time. To this end, the wells of a microtiter plate with V47188-1-carolacton (0.002%) composites (1 mm thickness) were covered with sterile phosphate-buffered saline (PBS) and stored at 4 °C for 19 (experiment 1) and 42 days (experiment 2), respectively. At the time point for testing, the supernatant was removed for a subset of samples, and biofilm formation and inhibition was determined on those same samples by Live/Dead staining as described. A potential biofilm inhibitory effect of the supernatant was also tested. In experiment 1 which lasted 19 days, samples were analysed on days 1, 7 and 19. Since little decline in anti-biofilm activity was observed, the experimental period was extended to 42 days in experiment 2, and the sampling days were days 1, 19, and 42.
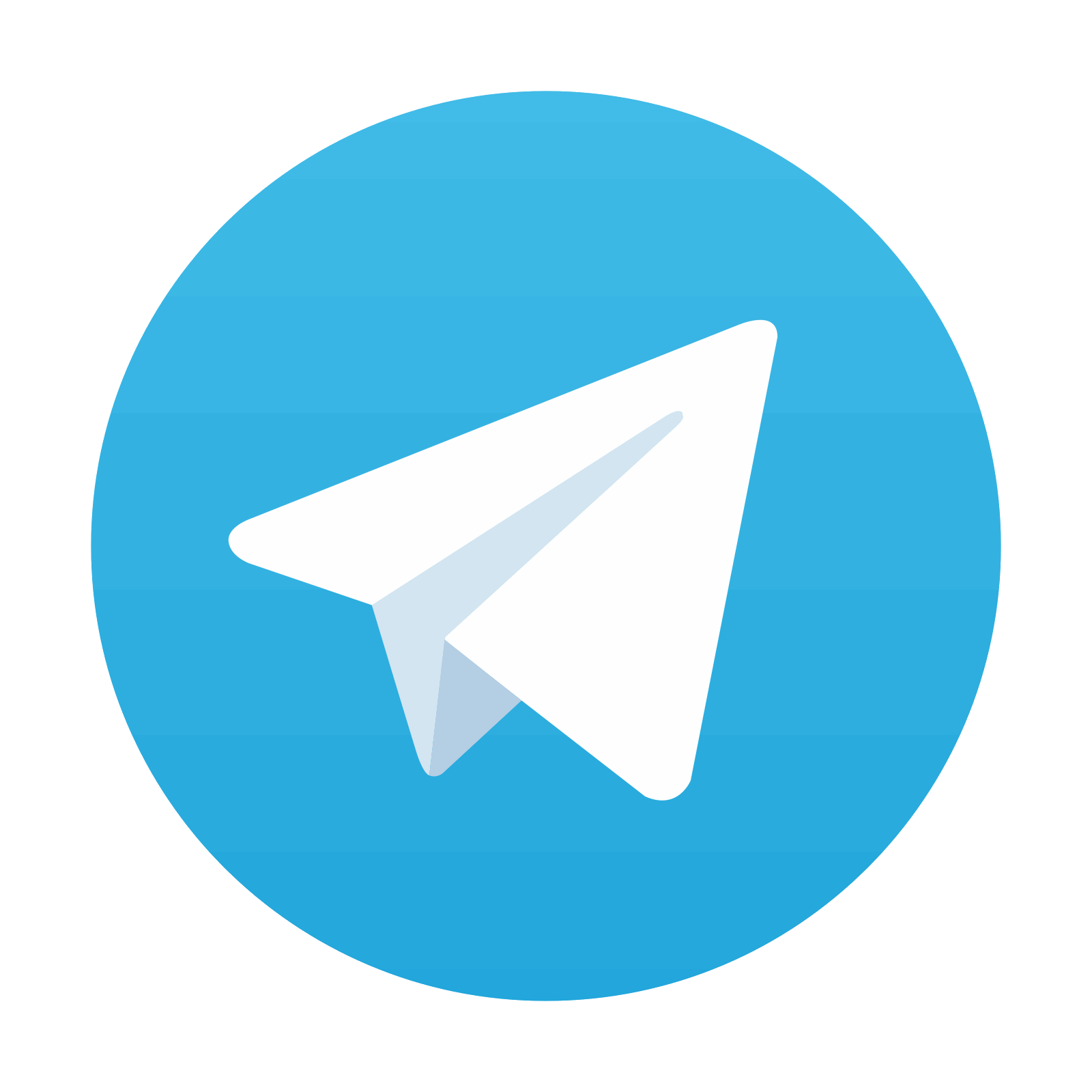
Stay updated, free dental videos. Join our Telegram channel

VIDEdental - Online dental courses
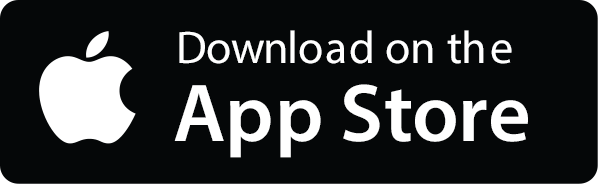
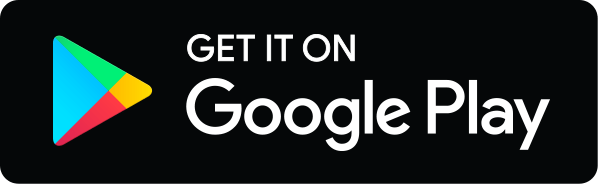