Abstract
Objective
The aim of this study was to investigate the effect of aluminosilicate clay nanotubes (Halloysite, HNT) incorporated into the adhesive resin of a commercially available three-step etch and rinse bonding system (Adper Scotchbond Multi-Purpose/SBMP) on dentin bond strength, as well as the effect on several key physicochemical properties of the modified adhesive.
Methods
Experimental adhesives were prepared by adding five distinct HNT amounts (5–30 wt.%) into the adhesive resin (w/v) of the SBMP dentin bonding system. Bond strength to human dentin, microhardness, and degree of conversion (DC) of the modified adhesives were assessed.
Results
From the shear bond strength data, it was determined that HNT incorporation at a concentration of 30 wt.% resulted in the highest bond strength to dentin that was statistically significant ( p = 0.025) when compared to the control. Even though a significant increase in microhardness ( p < 0.001) was seen for the 30 wt.% HNT-incorporated group, a significantly lower DC ( p < 0.001) was recorded when compared to the control.
Significance
It was concluded that HNT can be incorporated up to 20 wt.% without jeopardizing important physicochemical properties of the adhesive. The modification of the SBMP dentin bonding agent with 20 wt.% HNT appears to hold great potential toward contributing to a durable dentin bond; not only from the possibility of strengthening the bond interface, but also due to HNT intrinsic capability of encapsulating therapeutic agents such as matrix metalloproteinase (MMP) inhibitors.
1
Introduction
Adhesive dentistry has advanced tremendously over the past twenty years . Needless to say, bonding of resin composite restorations to enamel using the acid-etch technique advocated by Buonocore has been considered a safe and predictable restorative procedure . Regrettably, the achievement of a reliable and durable bond to dentin still poses a great challenge to the field, in part due to the high organic content levels existing in dentin (e.g., collagen) when compared to enamel . Nevertheless, another major contributor to resin–dentin bond degradation relates to the well-known hydrolytic process experienced by underpolymerized hydrophilic resins and the deterioration of water-rich, resin–sparse collagen matrices by matrix metalloproteinases (MMPs) and cysteine cathepsins .
In order to hinder resin–dentin bond degradation and therefore improve the longevity of resin-based restorations, numerous adhesive chemistries and bonding strategies have been proposed . Among them, recent advances in nanoscience and nanomaterials processing have supported the use of an ample variety of fillers in the form of nanorods, nanoparticles, whiskers and nanotubes as reinforcing agents in adhesive systems targeting not only improved material properties (i.e., adhesive) but also the enhancement of bonding to dentin . Noteworthy, the size scale of these fillers and the concomitant increased surface area yield a unique combination of mechanical properties through the reinforcement of the polymer matrix via localized plastic deformation around the fillers and crack deflection . Furthermore, it has been suggested that these nanofillers can infiltrate into the dentinal tubules, decreasing polymerization shrinkage, stiffening the adhesive layer, and ultimately strengthening the adhesive interface via micromechanical bonding .
A series of studies investigating the mechanical properties enhancement of engineered resins incorporated with aluminosilicate (Al 2 Si 2 O 5 (OH) 4 · n H 2 O) clay nanotubes (Halloysite, HNT) have been reported . Overall, the results from those studies led to the present work for two major reasons. First, aluminosilicate clay nanotubes have many advantages (e.g., biocompatibility, hydrophilicity, and high mechanical strength) that make them a good candidate to be used as a reinforcing agent for improving resin-based dental adhesive properties. Second, these clay nanotubes have been demonstrated to act as biologically safe reservoirs for encapsulation and controlled delivery of a wide variety of therapeutic drugs , which in adhesive dentistry could potentially serve as a carrier of MMP inhibitors, that could in turn positively contribute to minimizing and/or eliminating resin–dentin bond degradation . Currently, there appears to be no reports on the use of Halloysite in adhesive dental resins. The purpose of this study was to investigate the effect of incorporating Halloysite in the adhesive resin of a commercially available three-step etch and rinse bonding system on dentin bond strength. Physical properties of the HNT-incorporated adhesives such as microhardness and degree of conversion were also evaluated. The null hypotheses tested were that HNT incorporation would neither increase bond strength to dentin nor negatively impact adhesive properties such as degree of conversion and microhardness.
2
Materials and methods
2.1
Materials and experimental adhesive formulation
Halloysite aluminosilicate clay nanotubes (HNTs, Dragonite-1415JM ® ) were donated by Applied Minerals (New York, NY, USA). Previous to dentin bonding agent modification (i.e., the adhesive resin of Adper Scotchbond Multi-Purpose/SBMP), an aqueous dispersion of HNTs was prepared to confirm the surface morphology as well as the hollow structure of the nanotubes by scanning (FE-SEM, JEOL, JSM6701-F, Tokyo, Japan) and transmission electron microscopy (TEM, JEM-2010, JEOL, Tokyo, Japan), respectively . On an Al stub for HNT imaging, a predetermined approximate 0.5 mL volume of the HNT aqueous dispersion was added, allowed to dry and then sputter-coated with Au before SEM evaluation. Similarly, one 10 μL drop of the dispersion was added to a holey carbon TEM grid (Cu 200 mesh, SPI Supplies, West Chester, PA, USA), allowed to air-dry and imaged at 100 kV . Representative FE-SEM and TEM images of the HNTs are shown in Fig. 1 . HNTs displayed an overall uniform rod-like tubular structure with an estimated mean length in the submicron range ( Fig. 1 A). The HNT hollow structure was confirmed by TEM ( Fig. 1 B).
As previously mentioned, the experimental adhesives were prepared by adding five distinct HNT amounts (5, 10, 15, 20 and 30 wt.%) accurately (five-decimal-places) weighed using an analytical balance (Mettler Toledo, Ohio, OH, USA) into the adhesive resin (w/v) of the SBMP dentin bonding system. Briefly, HNTs were incorporated into a predetermined adhesive resin volume (w/v), mixed with a motorized mechanical stirrer using the companion conical micro pestle adapter (Roti-Speed, Roth, Karlsruhe, Germany) and then sonicated (Ultrasonic cleaning L&R 2014, Mfg Co., Kearney, NJ, USA) for 1 h to further enhance the dispersion of HNTs within the adhesive resin. Detailed information of the commercial dentin bonding system (SBMP) used is given in Table 1 .
Adper Scotchbond Multi-Purpose (SBMP) | Manufacturer | Batch no. | Components |
---|---|---|---|
1. Etchant | 3M/ESPE, USA | N190139 | 35% H 3 PO 4 |
2. Primer | N195379 | HEMA, polyalkenoic acid polymer, water | |
3. Adhesive | N311862 | Bis-GMA, HEMA, tertiary amines, photo-initiator |
2.2
Dentin bonding, shear testing, failure mode and resin–dentin interface evaluation
Shear bond testing has been considered one of the most simple methods for testing adhesives bond strength in vitro . Here, we used this test to screen for significant deleterious impact on the dentin bond strength achieved through HNT incorporation into the adhesive resin of a commercially available three-step etch and rinse bonding system (SBMP).
Seventy two caries-free human third molars were immersed in 0.5% chloramine solution after soft tissue removal and then stored in distilled water in a refrigerator at 4 °C. Teeth within six months of extraction were used under an approved IRB (Institutional Review Board, Indiana University-Purdue University Indianapolis) protocol (NS1004-03). Each tooth’s occlusal third was removed with a low-speed diamond saw (Buehler Ltd., Lake Bluff, IL, USA) mounted on a precision cutting machine (Isomet 1000, Buehler Ltd., Lake Bluff, IL). Teeth were individually embedded in Teflon molds using acrylic resin (Bosworth Fastray, powder lot# D681092139209, liquid lot# D68109213950C, Bosworth Company, Skokie, IL, USA). Following that, a flat mid-coronal dentin surface was obtained using a finishing procedure with SiC abrasive papers 240–600 grit under running water to ensure a standardized and uniform smear layer. The teeth were then randomly distributed into six groups ( n = 12), namely the control (G1-0 wt.%, no HNT incorporation) and five experimental groups (G2-5 wt.%, G3-10 wt.%, G4-15 wt.%, G5-20 wt.% and G6-30 wt.%).
For all groups (G1–G6), the exposed dentin surface was etched with 35% phosphoric acid for 15 s, rinsed copiously with distilled water for 30 s and gently blot dried with low-linting wipes (Kimwipes, Kimberly-Clark Professional, Roswell, GA, USA) according to the wet bonding technique as per the manufacturer’s recommendations. Next, the primer was applied with a microbrush and gently air-dried for 10 s. To ensure a homogeneous application, the primer was applied until a shiny surface was observed. Finally, the HNT-incorporated or the unfilled adhesive (G1-control) were applied to the primed dentin surface with a microbrush in agitating motions for 10 s and was gently dried for 5 s. A mild air blow was performed to help remove the excess solvent and then the adhesive cured for 20 s using a halogen curing unit (Optilux 501, Demetron/Kerr, Orange, CA, USA) with an output intensity of 720 mW/cm 2 . Next, a dental resin composite (Z100™, shade A2, lot# N290515) button (2.38 mm in diameter × 2 mm in thickness) was incrementally built on the bonded dentin surfaces using a dedicated jig (Ultradent, South Jordan, UT, USA) for shear bond testing and cured for 40 s in accordance with the manufacturer instructions. Specimens were stored in distilled water at 37 °C. After 24 h from bonding procedures, the specimens were individually placed in a stainless steel base (Ultradent, South Jordan, UT, USA) and loaded by a custom notched fixture (Ultradent, South Jordan, UT, USA) in compression using a universal testing machine (MTS Sintech Renew 1123, Eden Prairie, MN, USA) at a crosshead speed of 1 mm/min. Shear bond strength (SBS, in MPa) was calculated by dividing the maximum load (in N) at the time of fracture by the bonded area (in mm 2 ). The failure modes were assessed by means of light microscopy at 40× magnification and categorized as follows: adhesive (at the dentin–resin interface), cohesive (exclusively within the dentin substrate or composite), or mixed (at the dentin–resin interface including cohesive failure of one of the substrates). Randomly selected specimens representing each of the evaluated groups were sputter-coated with Au and imaged using a scanning electron microscope (SEM, JSM-5310LV, JEOL, Tokyo, Japan).
To investigate the resin–dentin interface of the HNT-incorporated adhesives, additional caries-free human third molars were used to obtain dentin slabs (ca. 2 mm in thickness) from mid-coronal dentin with a low-speed diamond saw (Buehler) mounted on a precision cutting machine (Isomet 1000, Buehler) . Bonding procedures were performed as previously mentioned in our dentin bonding protocol. In brief, the HNT-incorporated adhesives and the control groups were prepared and similarly applied to the smear-layer standardized dentin. Then a 1-mm thick flowable resin composite layer (Tetric ® EvoFlow, lot# P80394, Ivoclar-Vivadent, Schaan, Liechtenstein) was built and light-cured according to the manufacturer instructions. Bonded samples were stored at 37 °C in deionized water for 24 h. Bonded samples were sectioned perpendicular to the bonding interface using a low-speed water cooled diamond saw . Samples were mounted in self-cure epoxy resin (EpoxiCure ® , Buehler). Finally, the exposed cross-sectional interfaces of the resin/adhesive/dentin were wet-finished with SiC papers (400–1200 grit) followed by polishing using diamond impregnated papers 30, 9, and 3 μm (Apex ® Diamond Grinding Discs DGD, Buehler Ltd, USA). Samples were ultrasonically cleaned in distilled water for 5 min in between each of the diamond polishing steps and then in ethanol for additional 5 min to remove any remaining polishing debris . Finally, the polished surfaces were then demineralized with 6 N HCl for 30 s, followed by deproteinization in 5% sodium hypochlorite for 5 min, rinsed with deionized water and air-dried prior to Au sputtering for SEM imaging (JSM-6390, JEOL, Tokyo, Japan).
2.3
Knoop microhardness (KHN)
Disk-shaped (10 mm in diameter × 1.0 mm) specimens ( n = 5) of the adhesive for all the groups were fabricated using a custom made silicone mold (Exaflex ® putty, lot#1208281, GC America Inc., Alsip, IL) and stored at 37 °C for 24 h. The specimens were subjected to a microhardness test (M-400, Leco Corp., St. Joseph, Michigan USA) using a Knoop diamond indenter at a 50 gf load and 15 s dwell time. Eight readings at different locations (at least 1 mm apart) were obtained from each specimen. The diagonal lengths were measured immediately after each indentation. The values were converted to KHN number (kg/mm 2 ).
2.4
Degree of conversion (DC)
DC for the formulated HNT-incorporated adhesives, as well as the control, was evaluated at RT using a Fourier Transform infrared spectrometer (Jasco 4100, Jasco Corp., Tokyo, Japan) in attenuated total reflection (ATR) mode. Two absorbance bands at 1637 cm −1 (methacrylate group, C C) and 1715 cm −1 (ester group, C O) were used to calculate the DC. The scans were run twice per specimen ( n = 10). The average was used for analysis based on a previously established protocol . The degree of conversion was calculated by the following equation :
DC = 1 − ( Absorbance 1637 cm − 1 / Absorbance 1715 cm − 1 ) of polymerized sample ( Absorbance 1637 cm − 1 / Absorbance 1715 cm − 1 ) of unpolymerized sample × 100 %
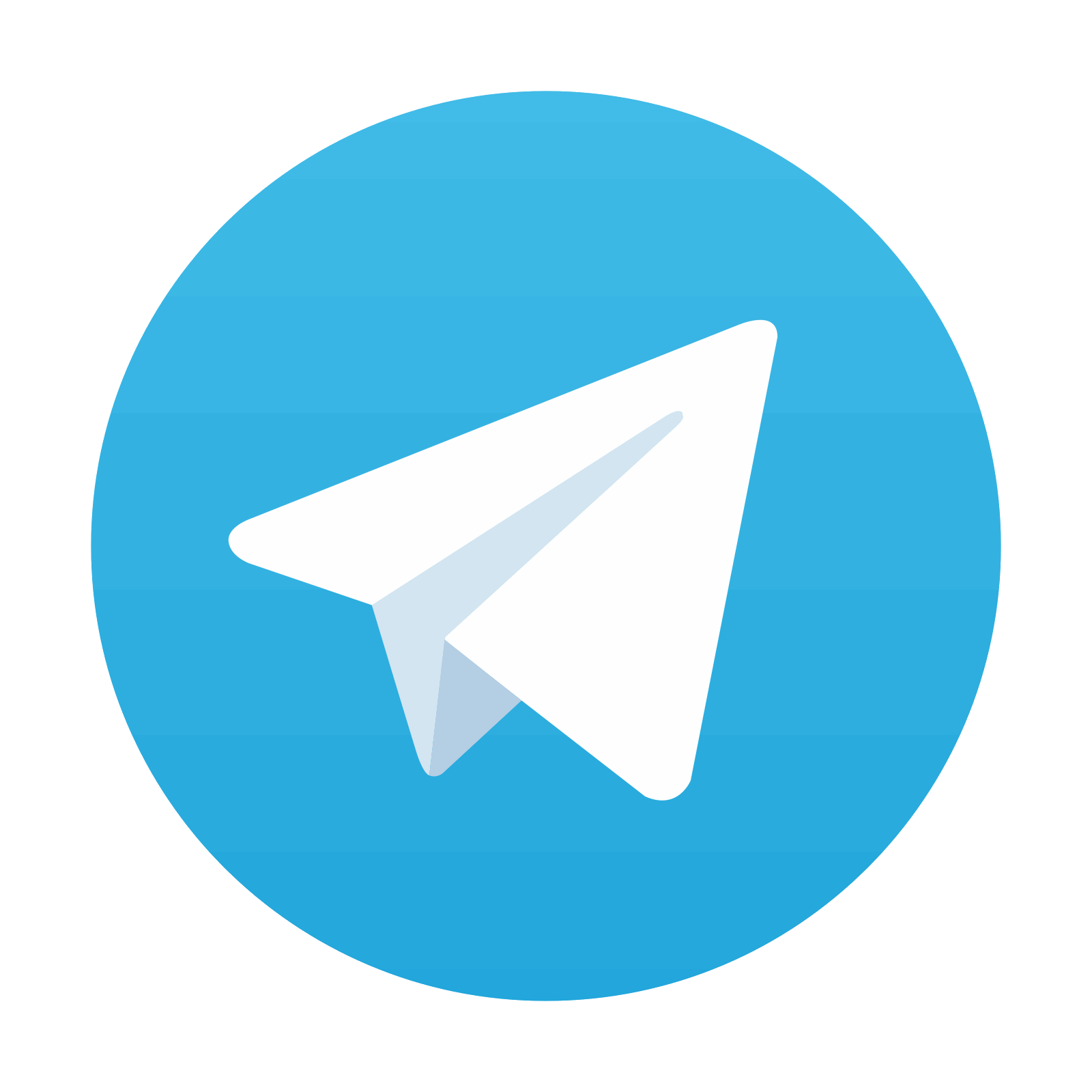
Stay updated, free dental videos. Join our Telegram channel

VIDEdental - Online dental courses
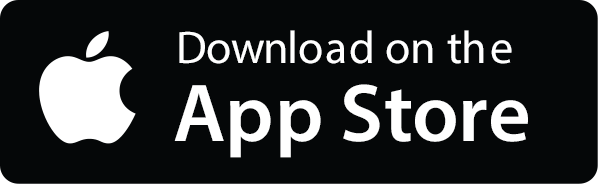
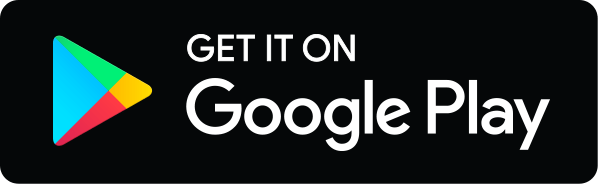