Key Terms
Acid–base reaction Chemical reaction between a compound containing replaceable hydrogen ions (acid) and a substance containing replaceable hydroxide ions (base) that yields a salt and water; for aqueous liquid and powder cements, the liquid is the acid, and the powder is the base.
Atraumatic restorative treatment (ART) Clinical procedure performed without dental burs, air/water spray, or anesthesia that consists of manual excavation of cavitated carious tissues and restoration of the tooth cavity with a fluoride-releasing cement.
Bioactivity Reactive potential of a material to form a layer of hydroxyapatite-like material on its surface in vivo.
Calcium phosphate cements Cement that is used for bone regeneration that consists of powder (di-, tri-, or tetra-calcium phosphates) that is mixed with an aqueous solution from which hydroxyapatite precipitates.
Cavity liner Material to coat the bottom of a cavity preparation to protect the pulp; a liner is applied in a thin layer.
Cement Substance that hardens from a viscous state to a solid union between two surfaces; for dental applications, cements act as a base, liner, filling material, root canal sealer, or adhesive to bind devices and prostheses to tooth structure or to each other.
Cement base Material used to protect the pulp in a cavity preparation by providing thermal insulation and sometimes a medicament, usually thicker and more distant from the pulp than a cavity liner.
Cement thickness Distance between an abutment tooth and cemented prosthesis, influenced by the design of the prosthesis and the viscosity of the cement during seating.
Compomer A term derived from the terms composite and ionomer ; a resin-based composite consisting of a silicate glass filler phase and a methacrylate-based matrix with carboxylic groups; also known as polyacid-modified glass-ionomer cement.
Endodontic sealer Material to prevent any transmittance of fluid or bacteria from the coronal to the apical region, used in conjunction with an obturation material such as gutta-percha.
Film thickness Thickness in μm of a cement 7 min after 150 N have been applied, which is the International Organization for Standardization (ISO) and American Dental Association (ADA) method for determining the ability of a cement to spread under pressure.
Glass-ionomer cement (GIC) Cement that hardens following an acid–base reaction between fluoroaluminosilicate glass powders and an aqueous-based polyacrylic solution; also known as polyalkenoate cement.
Hydraulic cement Cement that requires water to cause the material to set.
Luting agent A viscous cement-like material that fills a gap between two surfaces to prevent dislodgement. The term luting agent originally referred to nonadhesive agents only but now also includes adhesive agents.
Maturation Process of aqueous cement strengthening after setting.
Mineral trioxide aggregate (MTA) A tri- and di-calcium silicate-based cement used for vital pulp therapy and other endodontic indications.
Pulp capping A procedure to treat pulp that has been exposed through caries removal or trauma, by the application of a medicament; indirect pulp capping is the term used when the pulpal exposure is incipient, such as when dentinal tubules are visible in the cavity.
Resin cement A resin-based composite material used for attaching fixed prostheses or orthodontic brackets; these cements are less viscous than resin composite restorative materials.
Resin-modified glass-ionomer cement (hybrid ionomer cement) Cement that combines a glass powder and an aqueous solution of polymerizable groups attached to the polyacrylic acid.
Varnish Material applied to the floor of a cavity; a solution of natural gum, synthetic resins, or resins dissolved in volatile solvent, such as acetone, ether, or chloroform; material applied superficially to a tooth to release fluoride.
Zinc oxide eugenol (ZOE) cement Cement based on the reaction between zinc oxide and eugenol.
Zinc phosphate cement Substance formed by the reaction between zinc oxide powder and phosphoric acid liquid that can be used either as a base or as a luting agent.
Naturally occurring waxes and resins have been used since time immemorial for covering the surface of broken teeth and adhering replacement materials, including bone, animal teeth, wood, and early naturally occurring polymers. However, problems arose with many “natural” materials exposed to the warm, moist, and often acidic oral environment, leading to their gradual failure in vivo. Modern dentistry has evolved to achieve improved clinical results, which include a wide variety of cements created by various chemical reactions. Cements are used for many indications and may be needed for short-term (days to weeks), intermediate-term (weeks to months), and long-term (years) retention of dental appliances (devices).
History of Dental Cements
In the 1800s, zinc oxide (ZnO) powder of good purity was found to react with eugenol at room temperature to form a functional cement. The ZnO–eugenol material was used as an impression material and temporary restoration and was eventually found to be obtundent with the pulp and suitable as a root canal sealer. Zinc oxide was observed to react with phosphoric acid to yield a material stronger than ZnO–eugenol, capable of securing gold alloy restorations to tooth preparations. The high acidity of the ZnO–phosphate cement was unsuitable for pulpal contact but a good insulator for use as a base. In the mid-20th century, ZnO was also used with carboxylic acid to produce a cement that was lower in acidity and capable of bonding to tooth structure.
When the zinc oxide in zinc phosphate cement was replaced with a glass powder, a semitranslucent esthetic filling called silicate cement was created. The longevity of the silicate cement restorations was unimpressive (<4 years) because the silicate cements have high solubility. Gradually, the silicate cements lost anatomical contour and degraded at the margins. Despite the deficiency, the occurrence of recurrent caries adjacent to these cements was rare. Laboratory studies showed that fluoride was released from the glass in silicate cements. The impressive anticariogenic potential of the silicate cements highlighted the ability of fluoride ions (F−) to inhibit demineralization.
The lessons learned from silicate cement and zinc carboxylate cement led to the development of glass-ionomer cements (GICs), which contained a modified glass for the powder and polyacrylic acid for the liquid. Incorporating the polymerization technology of methacrylate resin into the liquid created dual-cured materials: acid–base and polymerization reactions. From this combination, resin-modified glass-ionomer cement (RMGI) evolved. Replacing the liquid component of RMGI with a water-free acidic polymerizable monomer created a light-curable single-component material called compomer, a term derived from the terms composite and ionomer . The goal of these two hybrid materials was to combine the strength of composite with the fluoride release and self-adhesion of glass ionomer in one material.
Around the mid-20th century, new attributes for dental cements were needed for use with gutta-percha points in endodontic therapy. These cements are denoted as endodontic sealers, which must cure in the moist root canal dentin. Furthermore, endodontic materials must ensure that oral bacteria cannot percolate through the root to the periapical bone; otherwise, serious infection persists or occurs. Esthetics are less important for endodontic cements than for supragingival indications. Several dental materials were adapted for endodontic use: zinc oxide–eugenol (ZOE), polyvinyl siloxane, epoxy resins, and hydraulic ceramics (tri-/dicalcium silicate). In the 1990s, the bioactivity and sealing ability of tri-/dicalcium silicate cements became recognized and appreciated in dentistry. This ceramic dental cement is now widely indicated for pulpal and periradicular contact, including endodontic indications.
Varnish has been used for pulpal protection, especially with amalgam in a cavity preparation. The use of varnish has declined with decreased amalgam use. However, surficial varnishes are popular, especially among pediatric dentists. These varnishes are placed on enamel to release fluoride to provide protection against caries and promote remineralization from acids in the oral cavity with calcium ions. Sealants based on resins are also used superficially on teeth to provide protection against caries.
In the 20th century, varnishes included silver ions, silver nitrate, and silver diammine fluoride solutions for arresting caries and reducing sensitivity. The hazard with silver compounds is imparting a black color to the caries, as discussed later.
Most current cements include polymers and ceramic powders. The remainder of this chapter is organized by general indications: pulp protection and enamel protection, fixed prosthodontic device cementation, endodontic restoratives, and sealing.
Indications for Dental Cements
Dental cements are classified according to their major component, as shown in Table 7-1 , which includes their general indications. Several formats are offered for dental cements: powder and liquid, two pastes, or single component. Biocompatibility, durability for the indication, and safety for the patient and dental practitioner are important general cement attributes, all with a “reasonable” price. Some cements achieve their biocompatibility by being mildly cytotoxic, whereas others are bioinert or bioactive, as described later. The biocompatibility of dental cements is discussed in general terms in this chapter. Principles of biocompatibility are discussed in Chapter 17, Biocompatibility .
Powder/Liquid Systems | ||||
Materials | Powder | Liquid | Reaction Type | Indications |
Zinc phosphate | Zinc oxide and magnesium oxide | Phosphoric acid, water | Acid–base reaction | Cementing metallic fixed prostheses |
Zinc oxide–eugenol | Zinc oxide | Eugenol | Acid–base reaction | Pulp protection, temporary cement |
Zinc oxide–eugenol (EBA modified) | Zinc oxide | Eugenol, EBA | Acid–base reaction | Pulp protection, temporary cement |
Zinc polycarboxylate | Zinc oxide and magnesium oxide | Polyacrylic acid, water | Acid–base reaction | Metallic fixed prostheses short spans or crowns |
Glass ionomer | Fluoroaluminosilicate glass | Polyacrylic acid, polybasic carboxylic acid, water | Acid–base reaction | Cementing crowns (alumina, zirconia metal), metal posts and core restoration |
Resin-modified glass ionomer (hybrid ionomer) | Fluoroaluminosilicate glass, chemical- and/or light-activated initiator(s) | Polyacrylic acid, water-soluble methacrylate monomer, water, activator | Light- or chemical-activated polymerization and acid–base reaction | Metal, lithium disilicate, alumina or zirconia prostheses, * metal posts/core |
Fluoroaluminosilicate glass, metallic oxides, sodium fluoride, chemical- and/or light-activated initiator(s) | Dimethacrylate/carboxylic monomers, multiple functional acrylate monomers, water, activator for chemical activation | Polymerization by light- and chemical-activation and acid–base reaction | ||
Fluoroaluminosilicate glass, nonreactive filler, reactive monomers | Nonreactive filler, methacrylate-modified polyalkanoic acid, water-soluble methacrylate monomer, water | Light-activated polymerization and acid–base reaction | ||
Epoxy | Methenamine, titania, silver | Bisphenol A, epichlorohydrin resin | Chemical crosslinking polymerization of epoxide | Root canal sealing |
Tri-/dicalcium silicate | Tri-/dicalcium silicate and radiopaque powder | Water-based liquid | Hydration with liquid | Pulpal protection, periradicular contact, endodontic sealing |
Two-Paste Systems Materials | Base Paste | Catalyst Paste | Reaction Type | Indications |
Compomer | Fluoroaluminosilicate glass, metallic oxides, sodium fluoride, chemical- and/or light-activated initiator(s) | Dimethacrylate/carboxylic monomers, multiple functional acrylate monomers water, activator (for chemical cure) | Light or chemical activation of polymerization and acid–base reaction | Cavities in deciduous teeth, cervical cavities in adults, anterior proximal restorations in adults, small load-bearing restorations in adults, temporary |
Resin cement | Methacrylate monomers, fillers, light-activated initiator(s) | Methacrylate monomers, fillers, activator (for chemical cure) | Polymerization by light and chemical activation, or chemical activation only | Ceramic veneers, Maryland bridges, lithium disilicate, alumina, zirconia, cores and posts |
Polymethyl methacrylate polymer beads | Liquid 1: Methacrylate monomers Liquid 2: Catalyst | Chemical-activated polymerization | ||
Disalicylate | Butyl ethylene glycol salicylate resin, bismuth oxide | Resimpol natural resin, 13% “MTA” (tri-/dicalcium silicate and tricalcium aluminate?), titania and silica | Chemical-activated polymerization | Endodontic sealing |
Disalicylate | Glycol disalicylate, titanium dioxide and barium sulfate, titania, zinc oxide | Ethyl toluene sulfonamide, calcium hydroxide, zinc oxide, zinc stearate | Chemical-activated polymerization | Pulp capping |
Epoxy | Bisphenol-A epoxy resin, bisphenol-F epoxy resin, calcium tungstate, zirconium oxide, silica, iron-oxide pigments | Dibenzydiamine, aminoadamantane, tricyclodecane-diamine, calcium tungstate, zirconium oxide, silica, silicone oil | Chemical-activated polymerization | Endodontic sealing |
Single ComponentMaterials | Paste or liquid | Reaction Type | Indications | |
Compomer | Methacrylate monomer, acidic monomer, fluoroaluminosilicate glass, initiator | Light-activated polymerization | Cavities in deciduous teeth, cervical cavities in adults, anterior proximal restorations in adults, small load-bearing restorations in adults, temporary | |
Varnish | Resins, organic solvent (acetone); chloroform ether may include silver, fluoride, or calcium compounds | Evaporation of solvent | Pulp protection, surficial ion release for caries prevention or remineralization | |
Inert | Methacrylate monomers, initiator, ceramic fillers | Light-activated polymerization | Cementing ceramic restorations or orthodontic brackets | |
Tri-/dicalcium silicate | Methacrylate monomers, initiators, tri-/dicalcium silicate, barium zirconate | Light-activated polymerization | Pulp-capping, pulpotomies | |
Tri-/dicalcium silicate | Organic liquid, tri-/dicalcium silicate, radiopaque powder | Hydration in vivo | Pulpotomies, endodontic sealing | |
Calcium hydroxide | Iodoform, silicone, calcium hydroxide | Nonsetting | Primary tooth pulpectomy | |
Tri-/dicalcium silicate | Tricalcium silicate, salicylate resin, titanium oxide, calcium tungstate | Nonsetting | Primary tooth pulpectomy |
Other characteristics are designed for a cement’s unique indications, such as viscosity. For pulpal protection or surficial tooth sealing, a fluid layer is usually suitable. A medium-viscosity cement is used for cavity liner or cement-base indications. For adhering an appliance, a cement must have a sufficiently low viscosity to flow along the interfaces between the tooth tissue and a restorative. A low-viscosity cement is also needed to enable an endodontic sealer to fill complex root canal anatomy. Orthodontic bands require a low-viscosity cement, but orthodontic brackets require higher-viscosity cements to keep orthodontic brackets from drifting before the cement is cured. Base/liner materials should flow and set within, but not out of, the cavity preparation. Viscous cements are used for filling perforations, for root-end filling, or for covering pulps after a pulpotomy. Whether low or high viscosity, a low-contact-angle material is useful for wetting the surface of enamel or dentin or a prosthetic device.
Cements must harden (set) within a reasonable time that depends on the indication, ranging from minutes to days. Strength requirements vary from a compressive strength of 35 MPa for pulp capping and base materials to 50 MPa for aqueous cements for the adhesion of devices. The strength should be appropriate for the indication and increase during maturation. For instance, the tri-/dicalcium silicate cements mature over about 1 month. All cements undergo a chemical reaction in vivo. Varnishes set by evaporation of the solvent to form a coherent protective layer. Two-part cements begin setting/hardening when the components are mixed. Single-component polymer-based cements set through polymerization activated by visible light. Dual-cured resin cements use both chemical and light-curing mechanisms to maximize polymerization. The hydraulic bioactive cements set by reaction with water that is either included in two-part systems or absorbed from the tooth. The exception is the nonsetting materials used with primary teeth after a pulpectomy, where a cement should not interfere with exfoliation.
Long-term retention of devices is important for crowns and bridges. However, temporary cements are sometimes needed, for instance, when transient devices are placed during prosthetic treatments. Dental cements are crucial for orthodontic brackets and bands, but the durability of these materials can be less than other cements because orthodontic treatments usually last about 2 years. Sealants and cements to reduce sensitivity (by occluding exposed dentinal tubules) are not expected to last long term.
Performance Criteria for Dental Cements
Dental cements must solidify into a biocompatible mass in the oral cavity and function over time in vivo by resisting hydrolysis (degradation by water). Properties of dental cements vary, but those that are often compared include the mixing time, working time, setting time, film thickness, compressive strength, and vulnerability to acid. Requirements for dental cements (base/liner and luting materials) have been codified in International Organization for Standardization (ISO) Standards 9917-1 and 9917-2 (Dentistry−Water-Based Cements) and ISO 6876:2012 (Root Canal Sealing Materials), where the test methods for these properties are described. These standards apply to water-based luting cement, bases, linings, restorations, core buildup materials, and root canal sealing. See Table 7-2 for the requirements. Except for root canal sealers, cements should set in less than 8 minutes (min) and resist acid erosion.
Solubility and Disintegration of Cements
Cements are often placed supragingivally and exposed to a variety of acids produced by microorganisms or as components of foods and drinks. ISO 9917-1 describes the erosion test of impinging a 0.1-M solution of lactic acid/sodium lactate (pH = 2.74) on water-based cements. The depth of erosion after 24 hours (h) is measured as mm/h. The results show that the rate of erosion decreased from conventional GIC to hybrid GIC to compomer. The solubility procedure described in ISO 4049 for dental composites uses the weight (mg) and volume (mm 3 ) of the specimen before immersion in water and the weight of the specimen after 5 days of immersion, and then desiccation. The solubility is calculated by dividing the weight changes by the volume. ISO 6876 for endodontic sealers measures the weight of the specimen before 24 h of immersion in water, and the weight percentage of residue is reported.
Testing conditions have been shown to significantly influence the solubility, such as varying the pH, the electrolytic concentration of the storage media, the soaking or impingement of a test medium on the cement, the powder-to-liquid (P/L) ratio of the cement, and the testing duration. For example, lowering the pH and P/L ratio will generally increase solubility. Delaying exposure to a storage medium decreases solubility. Some cements show no solubility (by weight) when concurrent dissolution and water sorption occur. Therefore poor correlation has been found for solubility data from the acidic-erosion and passive-weight-loss tests. In vitro data show that cement solubility differs among categories of materials and products. For the cements that rely on water to set, the solubility increases from glass ionomer to zinc phosphate and polycarboxylate cements. The solubilities between zinc polycarboxylate cement and zinc phosphate cement were not significantly different when mixed as recommended. However, a reduction in the powder-to-liquid ratio for zinc polycarboxylate reduced the viscosity and produced a cement that disintegrated rapidly. Zinc phosphate cements have a relatively low solubility in water; however, in vivo disintegration occurs with the lactic, acetic, and citric acids present in foods.
With solubility being inversely proportional to the resin content, resin cements are the least soluble of all dental cements, especially hydrophobic resins. The low solubility of resin cement makes this material suitable for cementing all-ceramic or resin-based restorations, where more cement may be exposed compared with metal or metal-ceramic restorations. Polymerizing monomers in glass-ionomer cements (RMGIs) have reduced the solubility compared with GIC.
The durability of some cements has been tested by placing small specimens of the cements in intraoral appliances that can be removed from the mouth to measure the loss of material. In one study, cements were inserted in tiny wells placed in the proximal surfaces of cast crown restorations. These crowns were cemented with temporary ZOE cement. After 1 year, the crowns were removed, and the cement loss was measured. GIC degraded the least, followed by zinc polycarboxylate cement, zinc phosphate cement, and zinc polycarboxylate cement mixed in a lower P/L ratio.
The solubilities of endodontic sealers based on ZOE and early formulations of glass ionomer were near the maximum allowed in ISO specifications (3%). ZOE-based root canal sealers have been used successfully for decades in endodontics, despite their high solubility. Sealers with epoxy or polyvinyl siloxane (PVS) bases have significantly lower solubility among endodontic sealers. The tri-/dicalcium silicate cements do not meet this limit after 24 hours; however, after longer times, these materials become less soluble. Immediately after setting, the tri-/dicalcium silicates have calcium hydroxide on the surface, which is beneficial for bioactivity but appears as solubility. Nevertheless, the tricalcium silicate sealers are highly successful clinically.
The variety of dental cements encompasses many material categories, and the properties vary widely, as discussed herein.
Fluoride-Releasing Materials
The anticariogenic potential of silicate cement confirms the ability of F – to inhibit demineralization and lead to the development of several fluoride-containing restorative materials and cements: conventional glass ionomers, resin-modified glass ionomers, polyacid-modified composites (compomers), and fluoridated varnishes. For the fluorosilicate glass-based materials, fluoride is first dissolved in the matrix from the glass during the acid–base reaction, and then released to the oral cavity. For fluoridated varnishes, the fillers incorporated in the material dissolve partially upon contact with saliva, and then F – is released from the varnishes. The rate of fluoride release depends on the cement matrix, porosity, and nature of the fluoridated filler (i.e., type, amount, particle, and size). Figure 7-1 illustrates the typical behavior of fluoride release from dental materials.

In vitro data have shown that fluoride release from GIC remains detectable for years, although the rate of release is reduced by a factor of 10 within the first few months ( Figure 7-1, A ). The release rate and duration depend on the cement matrix; porosity; and the fluoridated filler’s type, amount, silanation, and particle size. Figure 7-1, B & C illustrate the typical behavior of fluoride release from dental materials.
Factors Affecting Fluoride Release in Vitro
Fluoride-release studies usually are conducted in vitro, where the experimental conditions are controlled and the fluoride release is easier to measure. Also, the intrinsic characteristics of the material—such as formulas, filler compositions, ratios of component mixing, mixing procedure, and curing time—can be studied more easily. For such in vitro tests, the protocols have varied in terms of the composition and pH of storage media, the frequency of the storage solution change, and plaque and pellicle formation.
When the demineralization–remineralization cycling is used in in vitro studies, higher fluoride release is observed in acids. However, ion-enriched (ions other than F − ) storage media, such as artificial saliva, reduce the release of fluoride and surface coatings from human saliva. The presence of esterase or hydrolases in the storage media or on the surface of the material increases fluoride release. Although removal of the outer layer of the material by air polishing or finishing can lead to higher release, toothbrushing has not been found to affect fluoride release.
Clinical Significance
In vitro studies have shown that fluoride-releasing restoratives inhibit the enamel and dentin demineralization produced by acidic gels or demineralizing buffer solutions. The inhibition of enamel demineralization reached up to 7 mm from the edge of the material. These results were confirmed by microradiographic assessments of the lesion depth of artificial caries and the mineral density of enamel adjacent to a conventional and a resin-modified glass-ionomer. For both materials, lesion depth increased, and mineral density decreased, with increasing distance (1 to 3 mm) from the restoration margin.
Fluoride release varies between categories of material and brands and is influenced by the material and the material’s setting mechanisms, the fluoride content, and the testing conditions. Clinical studies have not clearly shown whether the initial burst or long-term release of F ions is clinically more important to prevent caries. Despite the proven effects from in vitro studies, clinical studies conflict as to whether these materials sufficiently prevent or inhibit recurrent caries compared with nonfluoridated restoratives. Further clinical studies, preferably in split-mouth designs, are needed to evaluate the impact of fluoride-releasing restoratives on recurrent caries development and progression, especially in patient groups that have limited access to or low compliance with prophylactic measures.
An in situ study compared the effectiveness of a conventional GIC, an RMGI, a compomer, and a fluoridated composite for resistance to enamel lesion formation using intraoral appliances embedded with these materials. Intraoral appliances were worn by the subjects for 4 weeks without additional application of topical fluorides. The results showed that only one ion-releasing material led to significantly lower lesion depth and mineral loss. No other materials had a preventive effect on recurrent caries. Clinical studies of fluoride-releasing composites often show no differences in the occurrence of recurrent caries.
Fluoride Recharge
Dental restoratives are frequently exposed to exogenous sources of fluoride, such as fluoridated dentifrices, mouth rinses, fluoride gels, and varnishes. These released fluoride ions can be absorbed by the dental restoratives to “recharge” with fluoride. A common recharging method is exposing the restorative to a concentrated fluoride agent such as 0.02% NaF solution (90 ppm) or 1.23% (12,300 ppm) acidulated phosphate fluoride (APF) solution for 4 to 5 min, although treatments up to 1 hour have been reported. Recharging is more effective with acidic fluoridating agents, such as APF. However, APF is known to cause surface damage of some restorative materials. The ability of a restorative material to be recharged and serve as a fluoride reservoir depends on the type and permeability of the material, the frequency of fluoride exposure, and the concentration of the fluoridating agent. Glass ionomers, resin-modified glass ionomers, and compomers are recharged more easily than composite resin-based materials. In vivo fluoride recharging is influenced by saliva and plaque. The higher viscosity of saliva reduces the diffusion of ions in and out of the material. The formation of a surface pellicle is a barrier hampering the recharging process. The release data for recharged materials is significantly higher in the first 24 hours but decreases within several days to the preexposure level ( Figure 7-1, A ), suggesting that the initial release may occur partly by washout of fluoride ions retained on the surface or in the pores of the material. The level of release after recharging depends on the concentration of the recharging medium and the duration of treatment.
What is the benefit of calcium hydroxide in contact with pulpal tissue?
Pulp Protection
Tooth enamel is a translucent crystalline bioceramic but is vulnerable to acids and the repeated stress of a lifetime of chewing. The underlying dentin may be exposed to the oral cavity when the enamel is worn, chemically eroded by acidic foods or cariogenic bacteria, mechanically abraded by food or opposing teeth, fractured, or prepared for restoration. Depending on the remaining dentin thickness, the exposed dentinal tubules provide a communication path to the pulp for acids and microbiological insults. The dentin must be covered to minimize further damage. When metallic material is used to restore the tooth, discomfort may arise from hot or cold nutrition or galvanic contact with metal restoratives on opposing dentition. During restorative procedures, the acidic components of some dental cements may irritate the pulp before setting. Therefore pulp protectants are required when little protective dentin is left after a cavity preparation.
Pulp protectants for cavity preparations approximating the pulp are denoted as pulp-capping materials, varnishes, cavity liners, or base materials. Pulp-capping materials are in direct contact with the pulp, and their biocompatibility is crucial to the pulp’s healing. Varnishes as very thin resin layers used to plug and block the dentinal tubules exposed in a cavity preparation. Cavity liners are thin layers and are used for indirect pulp capping when the remaining dentin is less than 1 mm and are more protective than varnishes. Base materials are placed in thicker layers than liners and are commonly placed farther from the pulp. The base material is needed for insulation from the thermal, chemical, or galvanic irritations that may occur with restorative materials.
Pulp-Capping Materials
Pulp-capping agents are used when the pulp has been exposed and bleeding is frank. The best pulp-capping and cavity-liner materials are bioactive and antimicrobial. Bioactivity, as defined in ISO 22317, is the spontaneous formation of a thin layer rich in Ca and P, similar to hydroxyapatite, on the surface of a material in vivo or synthetic body fluid. Bioactivity occurs by the release of calcium and hydroxide ions that create a high pH (alkaline), thus causing the precipitation of calcium phosphate from the supersaturated body fluids in the vicinity of their surface. Bone morphogenic protein (BMP) and transforming growth factor-beta one (TGF-β1) may be solubilized from the bone or dentine by the high pH and released to stimulate repair. The pulp can heal by forming pulpal odontoblasts on the calcium phosphate layer of the bioactive materials, forming reparative dentin. Such a histological result is shown in Figure 7-2 . Bioactive pulp-capping agents include calcium hydroxide powder and tri-/dicalcium silicate materials.

Calcium Hydroxide
Calcium hydroxide medicaments are available as powder, one-paste, or two-paste products with radiopaque fillers. Calcium hydroxide powder may simply be mixed with water and placed, or some products disperse the powder in a carrier such as polyethylene glycol liquid, such as UltraCal (Ultradent Inc.). Other products may include calcium hydroxide in a resin formula that sets chemically or with light, such as Dycal (Caulk Dentsply Sirona). Calcium hydroxide is sparingly soluble in water and does not set into a hardened cement during the procedure but gradually converts to calcium carbonate by carbon dioxide in the blood and oral fluids over time. Such products must not be left on the margins of the cavity preparation; otherwise, the margin will not be properly sealed. Calcium hydroxide is beneficial because of a high pH, which makes this material antimicrobial. However, calcium hydroxide alone does not set or provide much thermal insulation but is bioinert. Histologically, calcium hydroxide stimulates reparative dentin when placed on the pulp, although the reparative dentin may have tunnel defects.
Why is MTA cement biocompatible and considered bioactive?
Tri-/Dicalcium Silicate
Tri-/dicalcium silicate (tricalcium silicate, for short) products are also known by the names mineral trioxide aggregate (MTA), calcium silicate, biosilicates, or bioceramics. Chemically, the predominant compounds in these cements are tricalcium silicate and dicalcium silicate powders with inert radiopaque ceramic powders. The tricalcium silicate powders are unique ceramic compounds because they set with water and form calcium hydroxide as a reaction product within a hard matrix of hydrated tri- and dicalcium silicate. The calcium hydroxide reaction product renders bioactivity and imparts antimicrobial properties. Furthermore, because the calcium hydroxide is within a matrix, the alkalinity is sustained longer than in calcium hydroxide alone. This type of cement is thermally insulating and seals very well, without shrinkage.
For pulpotomies, hemorrhage should be controlled with a cotton pellet moistened with saline, a dilute solution of sodium hypochlorite (0.5% to 1.0%) before placement, to avoid blood seeping into the cement and ensure removal of enough affected pulp. The consistency of the tricalcium silicate can be fluid or putty-like for pulpal placement. After placement, any restorative material, including ZOE, GIC, hybrid ionomer liner, or flowable compomer or composite, can be placed immediately over the tricalcium silicate without waiting for a full set of tricalcium silicates. Bonding agents do not adhere to tricalcium silicates, and etching should not be performed directly on tricalcium silicates. The precipitation of hydroxyapatite at interfaces with the tissue should prevent the tricalcium silicates from being dislodged. After pulpotomy treatment, the pulp should be periodically assessed for vitality, as an indication of a dentinal barrier forming beneath the tricalcium silicate cement. Tricalcium silicates have been added to the resin matrix as pulp protectant, such as TheraCal and TheraCal PT (Bisco). These products set by light curing or dual curing of the resin, but their bioactivity is lessened by the resin encapsulation of the bioactive component.
The properties and applications of tri-/dicalcium silicate will be discussed in more detail later in the chapter.
Formocresol and Ferric Sulfate
Formocresol and ferric sulfate have been used as protective medicaments for partial or complete pulpotomy procedures, among other techniques, including electrosurgery and laser cautery. Ferric sulfate and formocresol staunch the bleeding pulp, but neither material is bioactive. By far, formocresol has been the most popular among pediatric dentists. Ferric sulfate–treated pulpotomies have led to the unfortunate consequence of root resorption. Calcium hydroxide has not been popular in pediatric dentistry because this material has been less successful with pulpal inflammation and tooth resorption in small, thin primary teeth. The bioactive tricalcium silicate–based products are superseding the use of formocresol or ferric sulfate medicaments for pediatric pulpotomies, with a better success rate for retention before exfoliation.
Pediatric pulpotomies may fail or may not be suitable because of deeper infection of the pulp. For these cases, the pulp is extirpated, and the canal is filled with a nonsetting medicament. The filling material is nonsetting to avoid interference with the primary tooth’s exfoliation. These materials should be radiopaque. Two such materials contain calcium hydroxide in silicone oil or tricalcium silicate powder in salicylate resin.
What is the purpose of using cavity varnishes, and why is copal varnish no longer used as often as it was during the 1960s through 1990?
Cavity Varnishes and Liners
When the remaining dentin thickness is less than 1 mm, irritants must be prevented from reaching the pulp through the dentinal tubules by placing a protective layer. The classic pulp protectant is a cavity varnish, which prevents pulpal irritation by occluding the exposed dentinal tubules.
Varnishes are solutions of synthetic resins or natural gums, such as copal or rosins, in an organic solvent (ethanol, acetone, chloroform, or ether). The varnish is applied as a thin coating on the dentin, which forms a coherent layer as the solvent evaporates in about 30 seconds. At least two layers of varnish should be applied to obtain a complete, continuous coating with few pinholes. Each varnish layer should be thoroughly dried before proceeding. Varnishes are applied with a disposable brush or a small pledget of cotton. For infection control, a used varnish applicator should not be inserted in a varnish bottle; hence, unit-dose containers are common.
Additional cavity-liner products include low-viscosity ZOE, glass ionomer, calcium hydroxide products, and the tricalcium silicates. Calcium hydroxide–containing products are preferred for cavity lining, for the same reason as the pulp-capping materials: bioactivity. Some liners adhere to the tooth, enhancing their ability to seal the dentinal tubules. Glass ionomers are less desirable in the vicinity of the pulp because of their acidity, but resin-modified glass ionomers are used. Varnish is not indicated or needed when adhesive materials are selected for restorations. The resin bonding systems serve the same role as varnish.
Fluoridated Varnishes
Varnishes containing fluoride are applied superficially on teeth. Varnishes reduce sensitivity by occluding dentinal tubules and provide fluoride release. Typically, these varnishes contain 5% sodium fluoride to elute fluoride ions, although others contain calcium fluoride or amorphous calcium phosphate. Varnish films are temporarily effective but must be reapplied for caries prevention at hygiene appointments. Superficial varnishes are often flavored for patient acceptance ( Figure 7-3 , left ).

Silver nitrate has a long history of use to arrest caries. Later, a Japanese researcher developed a 38% silver diammine fluoride solution for arresting caries and reducing dentinal sensitivity ( Figure 7-3 , right ). The silver ions are dissolved in an ammonia solution, which can attack and kill bacteria. The term silver diammine fluoride (SDF) has been accepted in dentistry for AgF • (NH 3 ) 2 . SDF solutions contain twice the fluoride present in a 5% NaF varnish. This solution will arrest caries by precipitating the silver ions but will darken carious lesions with silver precipitates. Darkening of the tooth can be alleviated by using a potassium iodide solution after application of the SDF. This material is convenient to use for the medically compromised or bedridden and primary teeth, despite the discoloration.
Cement Bases
Cement bases are more distant from the pulp and placed at the bottom of cavity preparations. Bases provide thermal or galvanic insulation ( Chapter 3, Clinical Significance of Galvanic Currents ), although eliminating communication to the pulp is also important. Being farther from the pulp allows some acidic materials to be used, such as zinc phosphate cement. Zinc phosphate cement has been an effective base material because this cement is thermally insulating but has a low pH (acidity) and therefore requires another material under the cement to protect the pulp. When mixed as a base, zinc phosphate cement should be kept cool and mixed to a thick, nontacky, putty-like consistency that slows the reaction and minimizes free acid on the surface when placed.
ZOE, zinc polycarboxylate, fast-setting glass ionomers, calcium hydroxide, and tricalcium silicate cements have been used as cavity bases. All these cements contain ceramic powders, which inherently have lower thermal and electrical conductivity than metals ( Table 7-3 ). Heat transfer is a complex phenomenon that depends on the material’s heat capacity, thickness, and density ( Chapter 3, Thermal Properties ).
Cement bases should be strong enough to withstand condensation forces during placement of restorative materials, especially amalgam, and the forces during mastication or bruxing. Table 7-4 shows the compressive strength of representative dental cements over 24 hours. For most dental cements, the strength increases rapidly over 30 min and keeps increasing on the first day. Notably, the tricalcium silicate cements mature by hydration more slowly and gain their strength over 1 month, but especially during the first week. The minimum strength required of base materials to resist masticatory forces has not been determined because of the complexity and the influence of the design of the prepared tooth cavity. However, a study of amalgam restorations placed over a hard-setting calcium hydroxide base showed no evidence of failure, even though the strength of the material was only about 10 MPa after 1 day. For a class I tooth preparation where the base is supported on all sides by tooth structure, less strength is necessary than is required for class II preparations.
During the initial seating of a three-unit fixed partial denture (bridge), the prosthesis fits the prepared tooth perfectly and appears to be retained on the tooth so tenaciously that removal requires a great deal of force. Why is cement required for retention under this condition?
Cements for Luting
The first zinc oxide–based cement for cementing fixed prostheses was not adhesive to the tooth or metal prostheses. The cement functioned by filling the microscopic space between the device and the tooth and hardening to a high cohesive mass, which prevented dislodgement ( Figure 7-4 ). The mechanical retention is obtained by the combination of the microscopically rough tooth and prosthesis surfaces, along with the rigid cement that fills the gaps. This mechanical retention is referred to as luting, where zinc phosphate is the dominating cement. The degree of cement retentiveness for a given restoration relies on many factors: prepared tooth form, height, number of abutments, accuracy of superstructure fit, distribution of the cement, and occlusion. Dental prostheses that are mechanically retained include the following: (1) fixed prosthodontics made of metal, ceramic-veneered metal, or ceramic; (2) temporary restorations; (3) pins and posts used for retention of restorations; and (4) implant superstructures. As discussed in Chapter 6, Luting Agents , luting traditionally refers to the use of nonadhesive materials for retaining prostheses, and bonding refers to the use of adhesive bonding materials. The distinction has faded in the literature; the use of adhesive bonding medium is also referred to as luting.

When a device is seated without a luting agent, point contacts are made between the tooth and the appliance ( Figure 7-5 A & B ). The cement is used to fill the areas that are not in contact. The cement prevents oral fluid from flowing between the surfaces, thereby preventing bacterial invasion. Luting of a device to a tooth requires a cement of low viscosity and low contact angle to the tooth and the appliance to form a continuous film ( Figure 7-5, C ), without the formation of voids at interface ( Figure 7-5, D ). Voids impair the cement’s ability to support and retain a prosthesis.

Porosity in powder-liquid cements is created from incorporating air while mixing powder into liquid. One study with luting agents showed that the method of mixing had no influence on the formation of small pores, but hand mixing yielded more large pores than triturator mixing. Porosity measurement of hand-mixed powder-to-liquid cements showed that polycarboxylate cement had the highest porosity, followed by zinc phosphate and GICs. Higher viscosity generally leads to more pores during mixing. If air bubbles are entrapped, masticatory forces may fracture weak appliances such as veneers. When a void-free layer is formed and hardens, one surface is wedged against another to resist the shearing stresses that might dislodge the appliance.
To choose a cement for attaching a fixed prosthesis, the key characteristics are the working time, setting time, consistency, ease of removal of excess material, and adhesion. Any cement for attachment must flow under pressure and wet (spread over) the tooth and prosthetic surfaces. The internationally accepted requirements for luting cements are shown in Tables 7-2 . Luting cements are required to have a film thickness less than 25 μm when tested according to American National Standards Institute (ANSI)/American Dental Association (ADA) Specification No. 96 and ISO 9917-1 or 9917-2. For endodontic sealers, which are discussed later, the maximum film thickness is 50 μm per ISO 6876. The film thickness essentially reflects the consistency of the cement mixture and is affected by the size of particles and the P/L ratio. The ISO film-thickness test method requires freshly mixed cement to be placed between two optically flat surfaces, and a 150-N vertical load is applied. Seven minutes later, the thickness of the film is measured. Such samples should appear to create continuous films.
ISO 9917-1 Water-Based Cements—Part 1: Powder/Liquid Acid-Base Cements | |||||||
Cement Type | Zn PHOSPHATE | Zn POLYCARBOXYLATE | GLASS IONOMER | ||||
Luting | Liner or Base | Luting | Liner or Base | Luting | Liner or Base | ||
Maximum mixing time (min) | 2.5 | 2 | 2.5 | 2 | 1.5 | 1.5 | |
Final setting time (min) | 8 | 6 | 8 | 6 | 8 | 6 | |
Maximum film thickness (μm) | 25 | nr | 25 | nr | 25 | nr | |
Minimum compressive strength (MPa) | 50 | ||||||
Maximum acid erosion (mm) | 0.3 | 0.4 | 0.17 | ||||
ISO 9917-2 Water-Based Cements—Part 2: Resin-Modified Cements | |||||||
Cement Use | Luting | Liner or Base | Restorative | ||||
Minimum working time (min) | 1.5 | 1.5 | 1.5 | ||||
Minimum setting time (min) | 8 | 6 | 6 | ||||
Maximum film thickness (μm) | 25 | nr | |||||
Minimum flexural strength (MPa) | 10 | 10 | 25 | ||||
ISO 6876 Root Canal Sealing Materials/ADA 57 Endodontic Sealing Materials | |||||||
Property | Requirement | ||||||
Flow (mm) | >17 | ||||||
Working time | To be stated by manufacturer in minutes or hours | ||||||
Setting time | To be stated by manufacturer in minutes or hours | ||||||
Maximum film thickness (μm) | 50 | ||||||
Solubility (%) | <3 | ||||||
Radiopacity (mm of equivalent Al) | >3 | ||||||
Dimensional stability (ADA 57 only) | <0.1% expansion, less than 1% shrinkage |
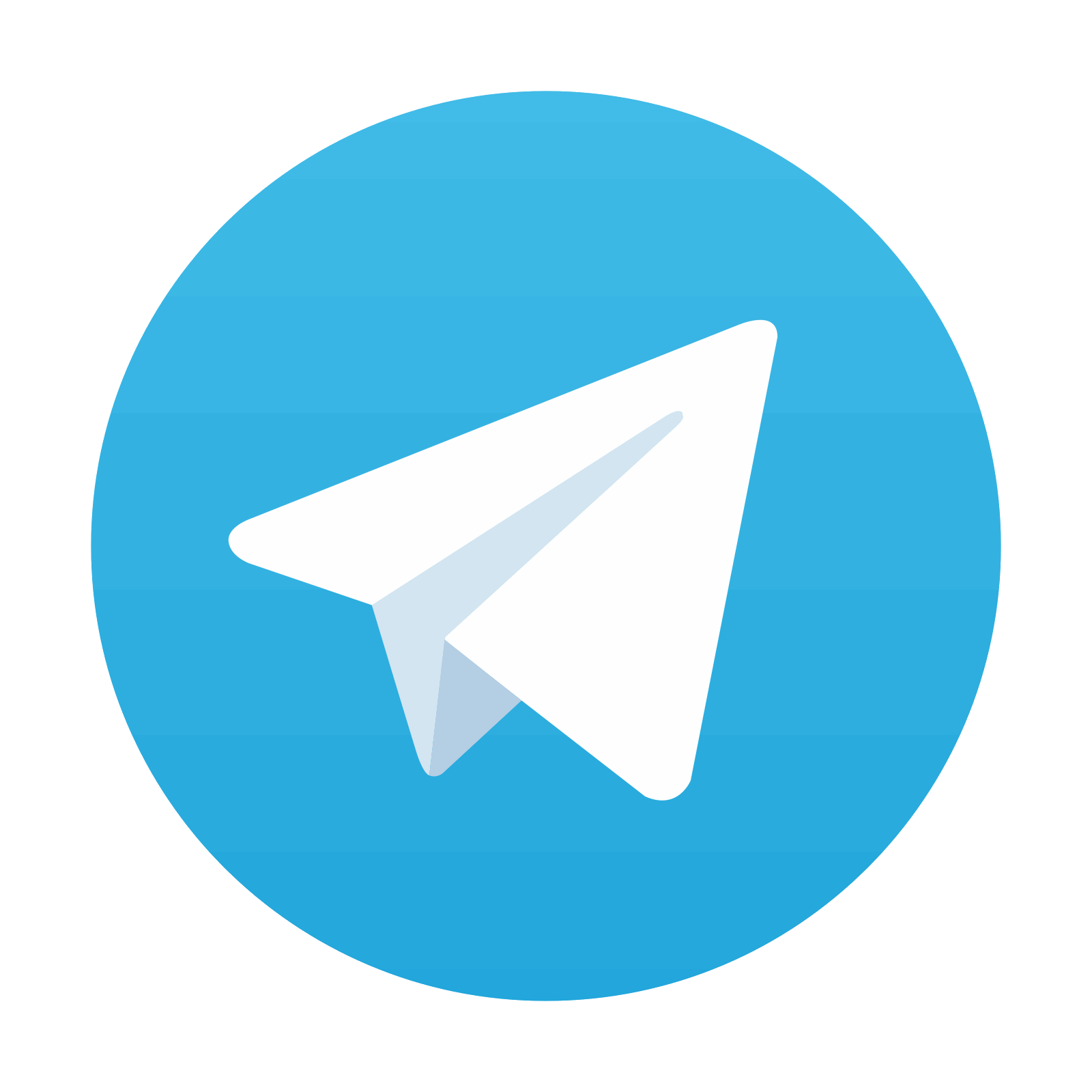
Stay updated, free dental videos. Join our Telegram channel

VIDEdental - Online dental courses
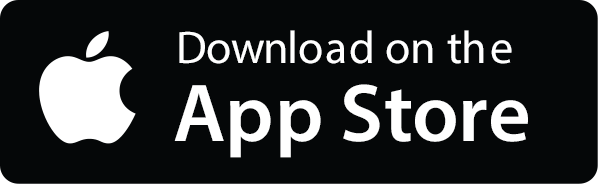
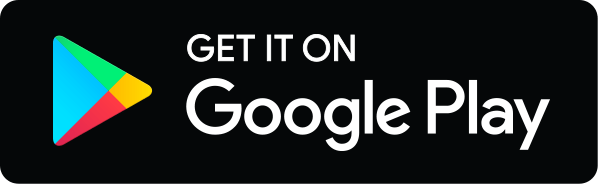
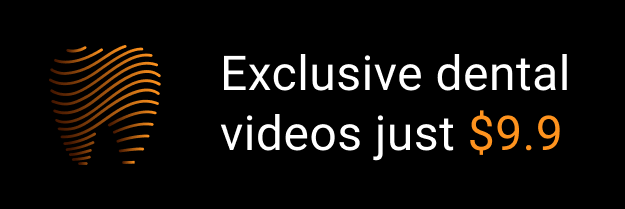