Abstract
Objectives
To evaluate the influence of temperature of evaporation in adhesive systems with different solvents on the apparent modulus of elasticity and mass change of macro-hybrid layers modified by proanthocyanidins (PACs).
Methods
Adhesive resin beams (A) from Single Bond Plus (SB), Excite (EX) and One Step Plus (OS) were prepared after solvent evaporation at 23 °C or 40 °C ( n = 12). Macro-hybrid layers (M) ( n = 12) were prepared using demineralized dentin beams sectioned from extracted human third molars. The demineralized dentin specimens were infiltrated with each one of the three adhesive systems at 23 °C or 40 °C; with or without prior dentin treatment with PACs for 10 min. The apparent modulus of elasticity ( E ) and mass change ( W mc , %) of adhesives beams and resin-infiltrated specimens were assessed in dry and wet conditions after immersion in water (24 h, 1, 3 and 6 months). The E was statistically analyzed by Tukey–Kramer test and the W mc , % by Kruskal Wallis, and Dunn ( α = 0.05).
Results
Solvent evaporation at 40 °C resulted in higher E values for adhesive resin beams at all storage conditions, regardless of the adhesive system ( p < 0.05). Increased mass loss (3 months: −0.01%; 6 months: −0.05%) was observed in One Step resin beams ( p ≤ 0.05). In the macro-hybrid layer models the pretreatment with PACs along with solvent evaporation at 40 °C increased E and decreased the W mc , % (3 months: −2.5; 6 months: 2.75%) for adhesives evaluated over time ( p < 0.05). No significant differences in ratio (resin/dentin) were found for the macro-hybrid layers ( p > 0.05).
Significance
Improved solvent evaporation at higher temperature, and increased collagen cross-linking induced by PACs, enhanced the mechanical properties resulting in highly stable macro-hybrid layers over 6 months storage.
1
Introduction
The clinical success and longevity of dental adhesive restorations depends on the stability of the resin–dentin interfacial components. The infiltration of resin monomers into a mineral depleted dentin matrix, results in the formation of a hybrid layer. Thus, the presence and the quality of the hybrid layer are believed to be essential for adhesion to dentin . However, there is no evidence that water in the intra and inter-microfibrillar compartments of the collagen matrix is completely replaced by resin . The remaining water present in dentin during resin infiltration increases the water absorption of hydrophilic resins , plasticize polymers, and accelerate degradation of the adhesive interface .
The resin co-monomers are dissolved in organic solvents that facilitate monomer diffusion within the collagen fibrils, and assist with the removal of water during solvent evaporation . Therefore, the residual water in the dentin and the organic solvents in adhesive systems need to be adequately volatilized as they can interfere in the mechanical properties of the hybrid layer . Clinical techniques using heat for evaporation of solvents result in higher mechanical properties and decreases interfacial micro permeability . However, despite the fact that these techniques have increased the evaporation of the solvents, there are limited long-term studies evaluating such techniques. Although evaporation of excess solvents can promote a highly cross-linked resin polymer, the hydrophilic nature of the monomer would not change, thus water absorption could plasticize the polymer chains and degrade the adhesive interface at the long-term .
The modification of dentin by plant-derived proanthocyanidins (PACs) modify the structure of collagen by increasing inter- and intra-molecular cross-links , enhancing the mechanical properties of the hybrid layer and increasing the hybrid layer resistant to degradation . The enhanced mechanical properties may also be due to the type of bonds between PACs and collagen, decreasing the hydrophilicity of the dentin matrix . This could decrease the degradation of the adhesive interface caused by long-term water absorption. In this context, flexural tests of macro-hybrid layer models can provide valuable information regarding the quantification of matrix shrinkage and resin uptake, as well as mechanical properties of the hybrid layer after infiltration of the adhesive .
Therefore, this study evaluated the association of tissue biomodification and temperatures for solvent evaporation to minimize the effects of water from the dentin substrate and excess of solvents from adhesives systems on the apparent modulus of elasticity and mass change of macro-hybrid layers over a 6-month storage period. The null hypothesis tested was that the biomodification of dentin matrix associated to warm temperature of solvent evaporation (40 °C) would not affect the mass change and modulus of elasticity of macro-hybrid layers created by different adhesive systems.
2
Materials and methods
2.1
Adhesive systems
Commercially available etch-and-rinse adhesive systems with different solvents were selected: Single Bond Plus [SB – ethanol and water based], 3M/ESPE, St. Paul, MN, USA, One Step Plus [OS – acetone based], Bisco, Schaumburg, IL, USA and Excite F [EX – ethanol based], Ivoclar Vivadent AG, Bendererstrasse, Schaan, Liechtenstein. The composition of the adhesive systems is described in Table 1 .
Adhesive systems | Composition | Batch number |
---|---|---|
Excite F (Ivoclar Vivadent) | 15% HEMA, dimethacrylates, Phosphonic acid acrylate, 25% Bis GMA highly dispersed silicone dioxide, initiators, stabilizers, potassium fluoride, 20% ethanol |
R78651 |
Adper Single Bond Plus (3M ESPE) | 25–35% ethyl alcohol 10–20% BIS-GMA 10–20% silane treated silica 5–15% HEMA 5–10% copolymer of acrylic and itaconic acids 5–10% glycerol 1,3-dimethacrylate <5% water 1–5% UDMA |
N446453 |
One Step Plus (Bisco) | 15–40% BPDM 10–40% HEMA 40–70% acetone photoinitiators |
1200007653 |
2.2
Adhesive resin beam preparation
One mL of each adhesive system was dispensed in a circular-plate and their initial mass was measured using an analytical balance (XP 504DR, Mettler Toledo Inc., Columbus, OH, USA). Each circular plate with the adhesive system was repeatedly weighed after 15, 30, 45 and 60 min until weight was stabilized. To keep traces of solvent in the adhesive, the time of volatilization was set at 30 min (based on pilot studies). The adhesive systems were kept in the dark for 30 min in an oven at 40 ± 1 °C or at room temperature (23 ± 1 °C). After time elaspsed, the adhesives were placed in polyvinyl siloxane molds (0.28 mm × 1.5 mm × 7.0 mm) and were immediately light-cured for 60 s on each side. Photo-activation was performed using a halogen-based unit (Optilux 501, Kerr Corp., Orange, CA, USA) with light intensity of 800 mW/cm 2 . Then, all adhesive resin beams ( n = 12 per group) with final dimensions 0.28 mm thickness × 1.5 mm width × 7.0 mm length were hand-polished with 600-grit silicon carbide paper with a silicone oil emulsion (Sigma–Aldrich, St. Louis, MO, USA) .
2.3
Macro-hybrid layer model
2.3.1
Dentin specimen preparation
Twenty-five extracted human third molars were obtained under a protocol approved by the Institutional Review Board Committee from Piracicaba Dental School, University of Campinas (protocol 068/2014). Teeth were stored in thymol solution 0.1%, at 4 °C and cleaned with periodontal curettes to remove organic and inorganic debris. Afterwards, the root and crown enamel were removed from the teeth, and dentin disks were obtained from mid-coronal dentin using a diamond, wafering blade (Buehler-Series 15LC Diamond, Buehler, Lake Bluff, IL), under constant water irrigation. Serial sections in the mesio-distal orientation were performed, resulting in dentin beams with 0.28 mm thickness ( n = 6 per tooth). Thus, 144 beams were obtained ( n = 12 per group) with final dimensions 0.28 mm thickness × 1.5 mm width × 7.0 mm length and a dimple was made at the margin of the beam for measurements to be performed on the same surface .
2.3.2
Dentin beams demineralization
The dentin beams were immersed in 10% phosphoric acid solution (Ricca Chemical Company, Arlington, TX, USA) for 5 h for complete demineralization . Specimens were thoroughly rinsed with distilled water.
2.3.3
Dentin matrix biomodification treatment
The dentin beams were randomly divided into twelve groups ( n = 12) according to dentin treatment: distilled water (control) or 6.5% PACs in water [95% proanthocyanidin-rich Vitis vinifera (MegaNatural™ gold grape seed extract, California, USA)] . The pH of the biomodification solution was adjusted to 7.2 and the demineralized dentin beams were immersed into the respective solutions for 10 min and rinsed.
2.3.4
Dehydration of the dentin beams
Due to the increased thickness of the macro-hybrid layers, as compared to the hybrid layer clinically created , a slow dehydration and infiltration process is necessary to assure that the resin infiltrates throughout the demineralized dentin beams. The dehydration process was performed with increasing concentrations of ethanol or acetone, according to the solvent present in each adhesive (SB and EX – ethanol; OS – acetone), starting from 50%, 70%, 80%, 90% immersion for 30 min, and two immersions in 100% for 30 min each .
2.3.5
Evaporation temperature and resin infiltration
The dehydration process was followed by immersion of dentin beams in 50% solvent in the dark (according to the solvent present in each adhesive)/50% adhesive system solution at room temperature for 30 min, and two immersions in 100% adhesive system for 30 min each. For the third immersion in 100% adhesive system, the beams were kept in the dark for 30 min at room temperature (23 ± 1 °C) or in an oven (Isotemp, Fisher Scientific, Pittsburgh, PA, USA) at 40 ± 1 °C to enable solvent evaporation. The macro-hybrid layers are thicker than hybrid layers created in clinical procedures , therefore, a passive volatilization of solvents was done (without the use of air jet) for 30 min but with the vial (containing the infiltrated beam) semi open to allow evaporation of solvents.
The resin-infiltrated dentin beams were placed between two mylar strips and immediately light cured as described for adhesive resin beams. The excess of adhesive around the dentin beams was removed using a scalpel blade and the margins and surface were hand-polished using 600-grit silicon carbide grinding paper with silicone oil emulsion (Sigma–Aldrich, St. Louis, MO, USA) .
2.4
Mass change evaluation
Resin-infiltrated specimens were weighed after demineralization of the dentin beams ( M 0 ), immediately following resin-infiltration ( M 1 ), and after specimens were kept in distilled water for 24 h ( M 2 ), 1 month, 3 and 6 months.
Dry mass ( M 0 and M 1 ) was assessed after specimens were individually stored in a desiccator containing anhydrous calcium sulfate at 37 °C for 24 h. The specimens were, then, immersed in distilled water for 24 h, 1 month, 3 months and 6 months in order to assess M 2 , M 3 , M 4 , M 5 , respectively. The excess water was wiped from the specimen surface using absorbent paper and then, they were immediately weighed on an analytical balance (XP 504DR, Mettler Toledo Inc., Columbus, OH, USA).
The resin/dentin matrix ratio ( R ) was assessed using the formula : R (%) = 100 − (( M 0 × 100)/ M 1 ), where M 0 is the dentin matrix mass – after demineralization and M 1 is the dentin beam after the resin-infiltration. In addition, the percent mass change ( W mc , %): was calculated according to the percentage mass gain of the adhesive resin beam or the resin-infiltrated dentin beam after immersion in 24 h of water storage using the formula : W mc (%) = ( M 2 − M 1 )/ M 1
2.5
Apparent modulus of elasticity – 3-point bending flexural method
The modulus of elasticity ( E ) of adhesive resin beams and resin-infiltrated dentin beams was assessed using the non-destructive three-point bending flexural test at a 2% strain , in the EZ Graph machine (Shimadzu, Kyoto, Japan) with 1 N load cell at 0.5 mm/min crosshead speed. The modulus of elasticity was obtained after 24 h storage in dry condition, and after immersion in distilled water for 24 h, 1 month, 3 months and 6 months. E was expressed in MPa (Mega Pascal) and calculated according to a previous study .
2
Materials and methods
2.1
Adhesive systems
Commercially available etch-and-rinse adhesive systems with different solvents were selected: Single Bond Plus [SB – ethanol and water based], 3M/ESPE, St. Paul, MN, USA, One Step Plus [OS – acetone based], Bisco, Schaumburg, IL, USA and Excite F [EX – ethanol based], Ivoclar Vivadent AG, Bendererstrasse, Schaan, Liechtenstein. The composition of the adhesive systems is described in Table 1 .
Adhesive systems | Composition | Batch number |
---|---|---|
Excite F (Ivoclar Vivadent) | 15% HEMA, dimethacrylates, Phosphonic acid acrylate, 25% Bis GMA highly dispersed silicone dioxide, initiators, stabilizers, potassium fluoride, 20% ethanol |
R78651 |
Adper Single Bond Plus (3M ESPE) | 25–35% ethyl alcohol 10–20% BIS-GMA 10–20% silane treated silica 5–15% HEMA 5–10% copolymer of acrylic and itaconic acids 5–10% glycerol 1,3-dimethacrylate <5% water 1–5% UDMA |
N446453 |
One Step Plus (Bisco) | 15–40% BPDM 10–40% HEMA 40–70% acetone photoinitiators |
1200007653 |
2.2
Adhesive resin beam preparation
One mL of each adhesive system was dispensed in a circular-plate and their initial mass was measured using an analytical balance (XP 504DR, Mettler Toledo Inc., Columbus, OH, USA). Each circular plate with the adhesive system was repeatedly weighed after 15, 30, 45 and 60 min until weight was stabilized. To keep traces of solvent in the adhesive, the time of volatilization was set at 30 min (based on pilot studies). The adhesive systems were kept in the dark for 30 min in an oven at 40 ± 1 °C or at room temperature (23 ± 1 °C). After time elaspsed, the adhesives were placed in polyvinyl siloxane molds (0.28 mm × 1.5 mm × 7.0 mm) and were immediately light-cured for 60 s on each side. Photo-activation was performed using a halogen-based unit (Optilux 501, Kerr Corp., Orange, CA, USA) with light intensity of 800 mW/cm 2 . Then, all adhesive resin beams ( n = 12 per group) with final dimensions 0.28 mm thickness × 1.5 mm width × 7.0 mm length were hand-polished with 600-grit silicon carbide paper with a silicone oil emulsion (Sigma–Aldrich, St. Louis, MO, USA) .
2.3
Macro-hybrid layer model
2.3.1
Dentin specimen preparation
Twenty-five extracted human third molars were obtained under a protocol approved by the Institutional Review Board Committee from Piracicaba Dental School, University of Campinas (protocol 068/2014). Teeth were stored in thymol solution 0.1%, at 4 °C and cleaned with periodontal curettes to remove organic and inorganic debris. Afterwards, the root and crown enamel were removed from the teeth, and dentin disks were obtained from mid-coronal dentin using a diamond, wafering blade (Buehler-Series 15LC Diamond, Buehler, Lake Bluff, IL), under constant water irrigation. Serial sections in the mesio-distal orientation were performed, resulting in dentin beams with 0.28 mm thickness ( n = 6 per tooth). Thus, 144 beams were obtained ( n = 12 per group) with final dimensions 0.28 mm thickness × 1.5 mm width × 7.0 mm length and a dimple was made at the margin of the beam for measurements to be performed on the same surface .
2.3.2
Dentin beams demineralization
The dentin beams were immersed in 10% phosphoric acid solution (Ricca Chemical Company, Arlington, TX, USA) for 5 h for complete demineralization . Specimens were thoroughly rinsed with distilled water.
2.3.3
Dentin matrix biomodification treatment
The dentin beams were randomly divided into twelve groups ( n = 12) according to dentin treatment: distilled water (control) or 6.5% PACs in water [95% proanthocyanidin-rich Vitis vinifera (MegaNatural™ gold grape seed extract, California, USA)] . The pH of the biomodification solution was adjusted to 7.2 and the demineralized dentin beams were immersed into the respective solutions for 10 min and rinsed.
2.3.4
Dehydration of the dentin beams
Due to the increased thickness of the macro-hybrid layers, as compared to the hybrid layer clinically created , a slow dehydration and infiltration process is necessary to assure that the resin infiltrates throughout the demineralized dentin beams. The dehydration process was performed with increasing concentrations of ethanol or acetone, according to the solvent present in each adhesive (SB and EX – ethanol; OS – acetone), starting from 50%, 70%, 80%, 90% immersion for 30 min, and two immersions in 100% for 30 min each .
2.3.5
Evaporation temperature and resin infiltration
The dehydration process was followed by immersion of dentin beams in 50% solvent in the dark (according to the solvent present in each adhesive)/50% adhesive system solution at room temperature for 30 min, and two immersions in 100% adhesive system for 30 min each. For the third immersion in 100% adhesive system, the beams were kept in the dark for 30 min at room temperature (23 ± 1 °C) or in an oven (Isotemp, Fisher Scientific, Pittsburgh, PA, USA) at 40 ± 1 °C to enable solvent evaporation. The macro-hybrid layers are thicker than hybrid layers created in clinical procedures , therefore, a passive volatilization of solvents was done (without the use of air jet) for 30 min but with the vial (containing the infiltrated beam) semi open to allow evaporation of solvents.
The resin-infiltrated dentin beams were placed between two mylar strips and immediately light cured as described for adhesive resin beams. The excess of adhesive around the dentin beams was removed using a scalpel blade and the margins and surface were hand-polished using 600-grit silicon carbide grinding paper with silicone oil emulsion (Sigma–Aldrich, St. Louis, MO, USA) .
2.4
Mass change evaluation
Resin-infiltrated specimens were weighed after demineralization of the dentin beams ( M 0 ), immediately following resin-infiltration ( M 1 ), and after specimens were kept in distilled water for 24 h ( M 2 ), 1 month, 3 and 6 months.
Dry mass ( M 0 and M 1 ) was assessed after specimens were individually stored in a desiccator containing anhydrous calcium sulfate at 37 °C for 24 h. The specimens were, then, immersed in distilled water for 24 h, 1 month, 3 months and 6 months in order to assess M 2 , M 3 , M 4 , M 5 , respectively. The excess water was wiped from the specimen surface using absorbent paper and then, they were immediately weighed on an analytical balance (XP 504DR, Mettler Toledo Inc., Columbus, OH, USA).
The resin/dentin matrix ratio ( R ) was assessed using the formula : R (%) = 100 − (( M 0 × 100)/ M 1 ), where M 0 is the dentin matrix mass – after demineralization and M 1 is the dentin beam after the resin-infiltration. In addition, the percent mass change ( W mc , %): was calculated according to the percentage mass gain of the adhesive resin beam or the resin-infiltrated dentin beam after immersion in 24 h of water storage using the formula : W mc (%) = ( M 2 − M 1 )/ M 1
2.5
Apparent modulus of elasticity – 3-point bending flexural method
The modulus of elasticity ( E ) of adhesive resin beams and resin-infiltrated dentin beams was assessed using the non-destructive three-point bending flexural test at a 2% strain , in the EZ Graph machine (Shimadzu, Kyoto, Japan) with 1 N load cell at 0.5 mm/min crosshead speed. The modulus of elasticity was obtained after 24 h storage in dry condition, and after immersion in distilled water for 24 h, 1 month, 3 months and 6 months. E was expressed in MPa (Mega Pascal) and calculated according to a previous study .
3
Statistical analysis
3.1
Apparent modulus of elasticity – E
The mixed models analysis for repeated measures was applied by PROC MIXED of SAS statistical program (SAS Institute Inc., Cary, NC, USA, Release 9.2, 2010). Multiple comparisons were performed by Tukey–Kramer test ( α = 0.05).
3.2
Mass change – W mc (%)
The mass change exploratory data analysis indicated that the data did not meet the assumptions of parametric analysis. Therefore, the mass change percentage was analyzed using Kruskal Wallis ( α = 0.05).
3.3
Resin–dentin matrix ratio
After exploratory data analysis for the resin/dentin matrix ratio, 3-way ANOVA was carried out using the SAS statistical program ( α = 0.05).
3.4
Pearson correlation
The effects of percent mass change on modulus of elasticity were evaluated with the Pearson correlation test ( α = 0.05)
4
Results
4.1
Adhesive resin beams
The E mean values and standard deviations from three etch-and-rinse adhesive beams at 40 °C and 23 °C and different storage conditions are presented in Table 2 . Solvent evaporation at 40 °C resulted in higher modulus values for OS, EX and SB in all storage conditions ( p < 0.05). EX and SB presented higher modulus of elasticity than OS in the different experimental conditions after 1 month of water storage, regardless of the temperature evaluated ( p < 0.05). When the storage conditions were compared for OS, the lowest E was observed after 3 months of immersion in distilled water, regardless of the temperature of volatilization ( p < 0.05). All adhesives evaluated in this study showed a decrease in modulus after 24 h water storage. However, for EX and SB, E increased and no statistically significant difference were observed between the storage conditions of 24 h dry and 6 months of water storage, regardless of temperatures of volatilization ( p > 0.05).
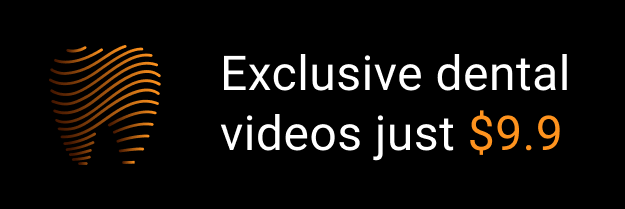