Highlights
- •
We developed experimental resin modified glass ionomer cement liquids (RMGICs).
- •
We tested water-uptake, desorption, dimensional change and other properties.
- •
The experimental cements were tested and compared with commercial and controls.
- •
All experimental materials showed promising results compared to commercials.
- •
New materials seem to solve problems commonly associated with commercial RMGICs.
Abstract
Objective
To investigate water uptake, desorption, diffusion coefficient, solubility and dimensional changes of four experimental RMGICs in deionized water (DW) and artificial saliva (AS), and compare with two commercial RMGICs and control home liquids based on the two commercial materials used.
Methods
Two commercial RMGICs, RelyX Luting (RX, 3M ESPE) and Fuji Plus (FP, GC), two control home liquids and four new liquid compositions (F1, F2, R1, R2) comprising different percentages of the monomer THFM (tetrahydrofurfuryl-methacrylate) with the original monomer HEMA (2-hydroxyethyl-methacrylate) were used in this study. Home and experimental liquids were mixed with the corresponding commercial powder. Disk-shaped specimens (16 mm diameter 1 mm thickness) were immersed in DW/AS at 37 °C ( n = 6) and weighed at regular time intervals. Percentage weight change with time was recorded. At 24 weeks, disks were desorbed in an oven at 37 °C to minimum weight.
Results
All new compositions showed lower water uptake and dimensional (volume) changes than the commercial products in both DW and AS. On desorption, FP showed higher weight loss compared to materials in the same group in both solutions ( p < 0.0001), with the exception of F2 in DW ( p = 0.283). RX had higher weight loss compared to R1 and R2 in DW and AS ( p < 0.0001). Fickian diffusion was confirmed for all materials immersed in DW and AS.
Significance
The experimental compositions in this study have shown promising results when tested in both DW and AS with lower water uptakes and volume changes than commercial materials. This may lead to wider applications than current commercial materials.
1
Introduction
Glass ionomer cements (GICs) were introduced by Wilson and Kent in 1972 and were first called glass polyalkenoate cements containing a degradable glass and a polymeric acid (poly(alkenoic acid)), e.g. poly(acrylic acid) . The term GIC has been adopted by clinicians although it does not describe accurately their chemical composition . GIC (also called conventional GIC) is best defined by McLean as ‘a cement that consists of basic glass and an acidic polymer, which sets by an acid–base reaction between these components’.
Conventional GICs possess many advantages such as good chemical adhesion to tooth substance , fluoride release and caries inhibition , do not undergo polymerization shrinkage nor produce an exotherm during setting . However, a number of limitations are associated with GICs as they are more susceptible to wear and abrasion, they possess low flexure strength and low modulus of elasticity, resulting in them being brittle and more ‘prone to fracture’ . Another disadvantage of GICs is their moisture sensitivity especially during the setting reaction and more importantly within the first 24 h of application (maturation phase) . If water is lost, then the setting reaction will be disrupted and this will subsequently affect the cement’s physical and mechanical properties .
Different approaches have been suggested and tested to overcome GIC’s weaknesses. One proposal included preparation of novel glasses with the addition of ‘dispersed-phases of crystallites’. These materials included the addition of large amounts of reinforcing crystals, e.g. corundum (Al 2 O 3 ), rutile (TiO 2 ), baddeleyite (ZrO 2 ) and tielite (Al 2 TiO 5 ) . These modifications showed improved flexural strength compared to conventional GIC, but with the other problems, e.g. sensitivity to moisture remaining the same .
A second suggestion was incorporation of different fibers (alumina, glass, carbon and silica) in the cement powder to enhance the flexural strength. However, in order to enhance the strength efficiently, the fibers needed to be added in large quantities, which adversely affected the handling properties .
Addition of metal fibers to conventional GIC was patented by Sced and Wilson . The benefit of this material is the increased flexural strength of the set cement. Simmons introduced the ‘miracle mixture’ in 1983, which involved the addition of amalgam alloy powder to the cement. Although these new compositions resulted in a set cement with improved flexural strength and abrasion resistance, they lacked in esthetics and they had lower bond strengths to enamel and dentin than conventional GICs, which led to more micro-leakage . Modifying the particle size and distribution of the GIC glass powder was found to improve mechanical properties and reduce the early sensitivity to moisture, but the esthetics and the translucency of the set cement were compromised .
All the above compositions and improvements did not solve two important problems associated with conventional GIC, sensitivity to moisture and ‘lack of command cure’ . In order to solve these problems, the incorporation of a resin system into the GIC cement was introduced, to provide a material that incorporates the advantages of a resin (e.g. improved strength) with the improved properties of GICs . This was first mentioned in a patent application in 1988 and it was introduced as a new development by Mitra in 1989 . Since then these cements have been further developed into a range of what we now know as resin modified glass ionomer cements (RMGICs). This type of cement incorporates two setting mechanisms: the conventional acid–base reaction and polymerization of the resin monomer, where polymerization can be either light or chemically activated . These materials have the same composition as conventional GICs, with the incorporation of poly(acrylic acid) or co-polymer of poly(acrylic acid) and other alkenoic acids, but the difference in RMGIC is the addition of a polymerizable resin monomer, commonly 2-hydroxyethyl methacrylate (HEMA). HEMA also works as a ‘co-solvent’ in order to keep all the components in one phase .
HEMA, as a hydrophilic monomer, plays an important role in the water uptake of RMGIC from the oral environment. A ‘mild hydrogel’ behavior has been associated with these cements . According to literature, hydrogels based on HEMA monomer are linked with high water uptake, which was reported to reach 80% by mass . Consequently, a higher amount of HEMA in the material correlated to higher water sorption and associated swelling of the RMGIC resin matrix . Different problems, e.g. plasticization and weakening of the cement have been linked to the water uptake of RMGICs . Cattani-Lorente et al. reported that RMGIC samples immersed in water, or in a humid environment for 24 h to three months, showed lower physical properties (flexure strength and hardness) compared to those stored in dry conditions; therefore, they correlated the decrease in the strength to the water uptake of RMGICs . A decrease in compressive and flexure strength following immersion in distilled water for 24 h and 150 days was also reported by Piwowarczyk and Lauer .
The aforementioned weaknesses of RMGICs have been attributed to the high water uptake of the RMGIC and this is reported to be correlated with the HEMA monomer due to its hydrophilic nature. With increased water sorption (e.g. in a clinical situation, where the restoration is not coated), the polymer matrix tends to be more ‘flexible’ and the physical properties of the material are adversely affected . The release of residual unreacted HEMA from the material has reduced biocompatibility where HEMA has been associated with both apoptosis and cell necrosis. The effects on cells were tested in vitro and the damage was seen in the cells that were exposed to the monomer . As an attempt to overcome the cytotoxicity of the material, Xie et al. introduced HEMA free RMGIC where they replaced HEMA with acrylate and methacrylate derivatives of amino acids. The authors reported that the new cement demonstrated improved mechanical properties compared to commercial products but did not seem to demonstrate the water uptake behavior of the new cement.
One of the possible alternative monomers to be used in RMGICs is tetrahydrofurfuryl methacrylate (THFM). THFM is a heterocyclic, hydrophobic monomer that has been patented for different potential biomedical applications such as temporary crown and bridge materials , fluoride releasing biomaterials , and as a tissue repair material . This monomer was also mentioned in patents concerning RMGICs . Mitra included it in the description and as one of the various choices that could be used in RMGICs, but did not include any examples or evidence of utilizing or testing the monomer. Anstice et al. included several examples of using THFM in RMGIC but without reporting on water uptake or desorption data. Moreover, they utilized THFM with different percentages of other monomers, and replaced all the HEMA in the cement.
THFM was studied with poly(ethyl methacrylate) (PEM), forming a low shrinkage, room temperature polymerizing system . The molar volume of THFM itself is high compared to methyl methacrylate (MMA), and this contributes to its low polymerization shrinkage . This characteristic was advantageous for potentially improving the fit of hearing aids .
Materials comprising room-temperature polymerizing PEM/THFM presented with a low polymerization exotherm and adequate mechanical properties for potential clinical use . Earlier water uptake data from distilled water for this system was reported to be ∼34% over two years, which was reduced to <3% in solutions like artificial saliva (AS) and phosphate buffered saline (PBS) . The mechanism of the water absorption process is reported to be in two stages; an initial Fickian stage until the matrix is saturated (<3%), followed by a second stage involving clusters/droplets being formed at hydrophilic/water soluble sites (e.g. clustering of the furfuryl rings or droplets formed around water soluble additives or impurities , indicating that the water uptake process is osmotically driven. On replacing part of the THFM monomer with HEMA (50:50) in the PEM/THFM room temperature polymerizing system resulted in an increased polymerization exotherm. Also its water absorption behavior was modified, due to suppression of the secondary uptake process (clustering) in the PEM/THFM system , and this was accompanied with increased diffusion coefficients.
Hence, the aims/objectives of this study were to:
Develop experimental RMGIC liquids by replacing the monomer HEMA partially with different percentages of THFM, but using them with the powder of commercial products.
Investigate water uptake, desorption, solubility and dimensional (volume) changes of commercial, home and experimental RMGICs.
2
Materials and methods
2.1
Materials
Two chemically cured RMGICs were included in this study, namely Fuji Plus (GC Corporation, Tokyo, Japan) and RelyX Luting (3M ESPE, St Paul, MN, USA). The two cements were supplied as separate powder and liquid components. The powder in both cements contained fluoroalumino-silicate glass and a redox system consisting of potassium persulfate and ascorbic acid capable of initiating polymerization chemically in the absence of light. The liquid in the two materials consisted mainly of water, acrylic acid, 2-hydroxyethyl methacrylate (HEMA) and tartaric acid ( Table 1 ).
RMGIC | Composition | CAS number | Percentage of components (by weight) | Powder: liquid mixing ratio |
---|---|---|---|---|
FP powder | Fluoroalumino-silicate glass | Not listed | 95–100 | 2 |
FP liquid | Distilled water | 7732-18-5 | 20–30 | 1 |
Poly(acrylic acid) | 9003-01-4 | 20–30 | ||
HEMA | 868-77-9 | 25–35 | ||
Urethanedimethacrylate (UDMA) | 72869-86-4 | <10 | ||
Tartaric acid | 87-69-4 | 5–7 | ||
RX powder | Fluoroalumino-silicate glass | Not listed | >98 | 1.6 |
Potassium persulfate | 7727-21-1 | ≤0.2 | ||
RX liquid | Water | 7732-18-5 | 30–40 | 1 |
Copolymer of acrylic and itaconic acids | 25948-33-8 | 30–40 | ||
HEMA | 868-77-9 | 25–35 | ||
Ethyl acetate | 141-78-6 | <2 | ||
Tartaric acid | Not listed | Not listed |
Two additional control (home) liquids were prepared in house based on the commercial liquids as given in the manufacturer’s safety data sheets (MSDS). Since it was not possible to determine the exact compositions of each product from the manufacturers, different percentages were tried and tested to match the commercial liquids. Home liquids were formulated and characterized using Fourier Transform Infrared Spectroscopy (FTIR) and compared with the commercial liquids. The final compositions of the home liquids are presented in Table 2 . Commercial and home liquids were stored in a cool dry place between 4 and 6 °C.
RMGIC | Composition | CAS number | Percentage of components (by weight) |
---|---|---|---|
FP in-house liquid | Distilled water | 7732-18-5 | 30 |
Poly(acrylic acid) | 9003-01-4 | 30 | |
HEMA | 868-77-9 | 31 | |
Urethanedimethacrylate (UDMA) | 72869-86-4 | 4 | |
Tartaric acid | 87-69-4 | 5 | |
RX in-house liquid | Water | 7732-18-5 | 35 |
Poly(acrylic acid) | 9003-01-4 | 30 | |
HEMA | 868-77-9 | 29 | |
Ethyl acetate | 141-78-6 | 1 | |
Tartaric acid | 87-69-4 | 5 |
Four experimental liquid formulations were prepared, two based on each of the commercial products (RX, FP), where HEMA was replaced with either 70%/30% THFM/HEMA in F2 and R2 or 50%/50% THFM/HEMA in F1 and R1. Attempts were made to replace all the HEMA in the liquid composition with 100% THFM but, as THFM is a hydrophobic monomer, it showed phase separation on mixing with water; thus there was a need for the THFM to be combined with HEMA to avoid this separation.
2.2
Immersion media
Deionized water (DW) and artificial saliva (AS) were used as immersion media. AS used was Orthano Saliva supplied by A.S. Pharma Ltd., UK. (components are given in Table 3 ; pH of AS was 6.5).
Component | Amount (g/100 ml) |
---|---|
Mucin gastric | 3.5 |
Xylitol | 2.0 |
Methyl parahydroxybenzoate | 0.1 |
E.D.T.A.-disodium | 0.05 |
Menate pipertae aetheroteum | 0.005 |
Spearmint oil | 0.005 |
Benzalkonium chloride | 0.002 |
Sodium fluoride | 0.00042 |
Na + | 0.08 |
K + | 0.07 |
Ca 2+ | 0.025 |
Mg 2− | 0.015 |
HPO 4 2− | 0.022 |
Cl − | 0.12 |
2.3
Methods
2.3.1
Sample preparation
Twelve discs were prepared for each material, six immersed in DW and six in AS. Mixing and preparing the samples was performed according to the manufacturer’s instructions and the manufacturers recommended powder: liquid mixing ratio by weight (2:1 g:g for FP group and 1.6:1 for RX group) ( Table 1 ). The components were mixed on a glass slab with a stainless-steel spatula at room temperature. Two discs were prepared at a time using polytetrafluoroethylene (PTFE) molds with internal dimensions of 16 mm ± 0.1 mm diameter and 1.0 ± 0.1 mm depth. The molds were placed on a glass microscope slide covered with an acetate sheet and were slightly over filled with the mixed materials; another acetate sheet and glass microscope slide was placed on top of the material while applying pressure manually to remove excess material. This assembly was clamped together with a bulldog clip in order to keep the samples in place and eliminate entrapment of air bubbles. Samples were left to set in an oven (Carbolite, Camlab, Cambridge, UK) at 37 ± 1 °C for 1 h. Following removal from the oven and molds, each specimen underwent visual examination to check for internal porosities or defects and any with porosities or imperfections were discarded. Samples were finished by hand using dry silicon carbide abrasive paper P600 (Buehler, IL, USA) to remove any irregularities on the periphery of each disk and the debris was blown away with a dust blower. Any samples with porosities or imperfections were discarded.
2.3.2
Water uptake
Following 1 h from the start of mixing, samples were weighed on an electronic balance (Explorer, Ohaus Europe GmbH, Nänikon, Switzerland) to an accuracy of ±0.0001 g. Each sample was then immersed in individual numbered screw top bottles filled with 100 ml of DW or AS at 37 °C. At regular time intervals following immersion, samples were removed from the bottles, blotted on blotting paper to remove excess water and weighed. Care was taken so the whole process did not take more than 20–30 s to avoid water loss. Several readings were taken on the first day and then at regular time intervals thereafter up to 24 weeks.
At time ‘ t ’, the percentage weight change was calculated for each specimen using Eq. (1) .
where W t is the weight at time t ; W 0 is the weight before immersion in solution. The mean weight change (%) and standard deviation (SD) was calculated then plotted against time 1/2 (seconds) in order to create the weight change profile for each material.
2.3.3
Desorption, solubility and diffusion coefficient
After 24 weeks, samples were removed from the solutions, blotted, weighed and then transferred to an oven (Carbolite, Camlab, Cambridge, UK) maintained at 37 ± 1 °C. Again, they were weighed at regular time intervals, as for water uptake, until a constant minimum weight was reached ( W d ).
The percentage weight loss after desorption was also calculated using Eq. (1) but replacing W t with W d and then, as for water uptake, plotted against time 1/2 (seconds).
The solubility of the material was calculated by subtracting the weight after desorption ( W d ) from the initial specimen weight ( W 0 ) (Eq. (2) ). This is equivalent to the total mass of components leached from the material.
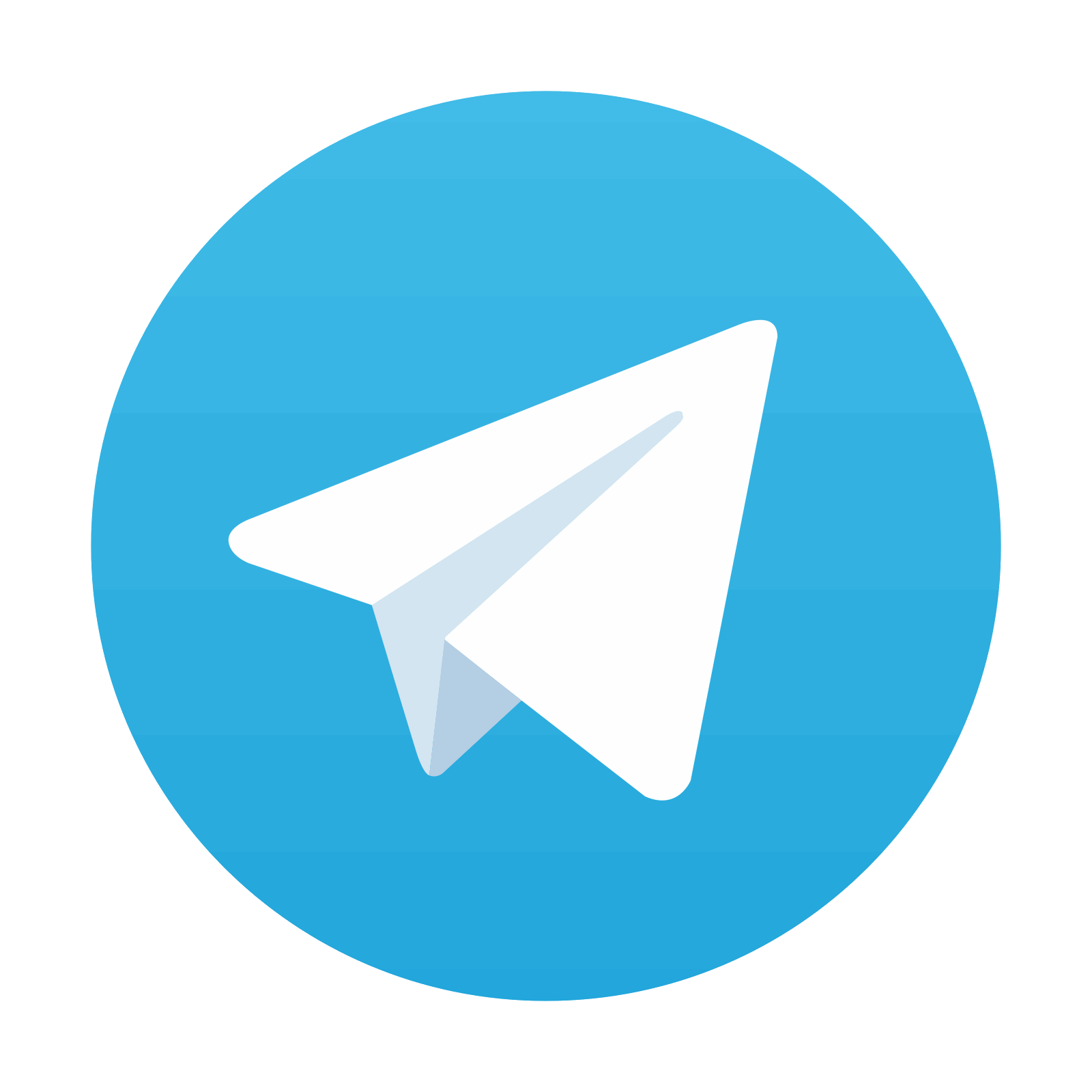
Stay updated, free dental videos. Join our Telegram channel

VIDEdental - Online dental courses
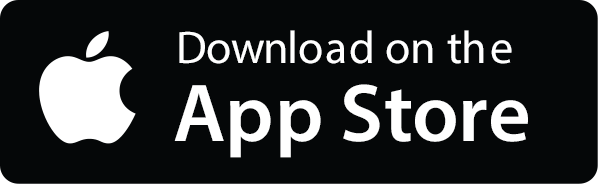
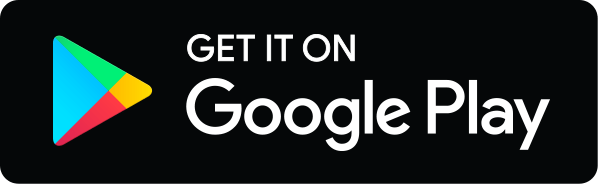