Abstract
Objective
To develop polymeric hydrogel delivery systems for iontophorseis transfer of large molecules across buccal (porcine) mucosa.
Methods
Three hydrogels (PVA, HPMC and PVA/HPMC) were prepared as stable gels (7 mm diameter/1.5 mm thick). Quantitative (8 and 36 h) assessment of porcine buccal mucosa and the three hydrogel delivery systems, using a diffusion cell in vitro model, was carried out by UV/vis spectroscopy with three model agents (3 and 10 kDa dextrans and 12 kDa parvalbumin). Passive and iontophoresis parameters were obtained. Experimental and theoretical data were compared.
Results
Iontophoresis (30 min, 1–8 h) significantly enhanced the delivery of all model agents across four single systems (hydrogels and buccal mucosa) and three sandwich systems (hydrogels on top of buccal mucosa), as confirmed by time lag factor/enhancement ratio (TLF/ER) data. The diffusion coefficients of model agents across buccal mucosa (×10 −13 m 2 s −1 ) were ∼100 times lower than across single hydrogels (2.97–4.80 × 10 −11 m 2 s −1 ). Solubility values of all agents across hydrogels were similar, but lower across buccal mucosa. Permeability of parvalbumin was highest across PVA, and for both dextrans across PVA/HPMC. In sandwich systems TLFs were similar for all hydrogels, but significantly lower, and ERs significantly higher, than tissue alone. Experimental and theoretical TLF data were in reasonable agreement.
Significance
The in vitro data show that iontophoresis enhanced the delivery of large molecules across polymeric hydrogel systems and buccal mucosa. This creates the opportunity of new approaches to drug delivery and opens pathways to further research for delivering therapeutic agents topically and systemically.
1
Introduction
Ionotophoresis enhances the rate of movement of ionic compounds across membranes by the application of an externally applied electric potential . This non-invasive method can be used to achieve both a local effect and significant levels of drug in the systemic circulation whilst avoiding the hepatic first pass clearance . Therefore local delivery of large macromolecules, such as peptides and protein drugs, may become possible using this approach . In an earlier paper we demonstrated that iontophoresis caused enhanced transmucosal transport of model species in an in vitro porcine buccal mucosal model system , thus expanding the use of oral mucosa for topical and systemic delivery of a range of therapeutic drugs. The crucial next step in developing a clinical application that takes advantage of this effect is to determine if it is possible to incorporate the target compounds in a delivery system, and achieve accelerated mucosal transport using iontophoresis.
A drug in solution is not readily amenable to incorporation into an iontophoretic device. To circumvent this semi-solid formulations, particularly gels, have been used extensively as drug delivery iontophoretic patches. Prerequisites of such a dosage form include optimal mechanical properties, good electro-conductivity, bioadhesion and acceptable viscoelastic properties that are imperative for patient compliance and clinical efficacy . Here, we describe the accelerated delivery of model species from polymeric hydrogels and across buccal mucosa by the application of iontophoresis.
Iontophoretic delivery systems based on hydrogel formulations can be designed as a unit dose-type, drug-loaded patch that permits daily or weekly dosage replacement . These formulations are used widely in transdermal iontophoretic drug delivery due to their electro-conductivity, adhesiveness and biocompatibility . However, if they could be adapted for use on buccal mucosa, the higher permeability of the latter compared to skin might be expected to result in enhanced uptake. Thus iontophoretic buccal mucosal patches would have potential for local therapy and also raise the possibility of using the site to achieve higher systemic levels of a therapeutic agent than could be obtained with skin patches.
The release of drugs from a hydrogel delivery system involves absorption of water into the polymer matrix and subsequent diffusion of the drug as determined by Fick’s Law. Kim et al. reported that factors affecting drug release from hydrogels include the drug loading method, the overall hydrophobic/hydrophilic balance, the osmotic effect of dissolved drugs, and polymer chain segmental stiffness. Hydrogels are water-soluble matrices that swell in aqueous media, and release a drug through spaces in the polymeric network by dissolution and disintegration. To prevent dissolution and breakdown of the hydrogel in the medium, they are normally crosslinked. Examples are poly (acrylic acid) crosslinked with poly(vinyl pyrrolidone) (PVP) or poly(ethylene glycol), to form a tighter network and claimed improved wettability .
Release of a range of therapeutic agents from hydrogels via iontophoresis has been reported. These include luteinising hormones from poly(hydroxyethyl methacrylate), enoxacin from poly(vinyl pyrrolidone) and diclophenac sodium from poly(vinyl alcohol) (PVA) . Fang et al. observed high levels of enoxacin and diclofenac sodium from methylcellulose and PVP hydrogels via transdermal iontophoresis delivery. Later they reported that hydrogel formulations including PVP and hydroxypropyl methycellulose (HPMC) have much more significant effects on iontophoretic delivery of nalbuphine in comparison with passive diffusion . Hence the range of hydrogels can be tailored for delivering numerous specific drugs and proteins.
We have previously shown that iontophoresis enhanced the delivery of 3 and 10 kDa dextrans and 12 kDa parvalbumin across buccal mucosa, confirmed by a reduction in the time lag factor (TLF: 0.12–0.16) and an enhancement of the flux (Enhancement Ratio-ER: 31.5–37.8) . In this earlier study the model agents were applied directly to the surface of the buccal mucosa. The aims of the present study were:
- 1.
To formulate three polymeric hydrogel delivery systems based on poly(vinyl alcohol) (PVA), hydroxypropyl methycellulose (HPMC) and PVA/HPMC binary systems.
- 2.
To investigate the passive/iontophoretic transfer of the model agents used in the earlier study across these hydrogel systems, to confirm that they can be used as delivery vehicles. Their performance was compared with that of porcine buccal mucosal tissue.
- 3.
To determine if iontophoresis can enhance the release of these model agents from hydrogels and their subsequent transfer across buccal mucosa in vitro.
A mathematical model was used to analyse iontophoresis of single systems, so that theoretical and experimental values of ER and TLF could be related to ν (a parameter proportional to the potential difference across the membrane) and the accelerating voltage ( V : potential difference).
2
Materials and methods
2.1
Materials
Samples of porcine buccal mucosa (Chealmeats, Brentwood, UK) were stored at −70 °C until use . Three fluorescently-labeled model compounds with different molecular weights were obtained from Molecular Probes, Inc., Eugene, OR, USA: Tetramethyl rhodamine labeled 3 kDa dextran (1 mg/ml in HEPES buffer ; pH 7.4; Melford Laboratories UK); Fluoresceinated 10 kDa dextran (1 mg/ml); Alexa Fluor ® 488 conjugated parvalbumin (12 kDa; 1 mg/ml). PVA, HPMC and glutaraldehyde were obtained from Sigma–Aldrich Ltd., UK. Silver/silver chloride electrodes were prepared according to Green et al. .
2.2
Methods
Single system studies refer to delivery of model agent across either buccal mucosa or the hydrogels, namely PVA, HPMC, PVA/HPMC ( Fig. 1 ). To elucidate the transport of each model agent through the systems (without being trapped in the polymer network), the donor solution containing each model agent was placed in direct contact with the surface of each system.

Sandwich system studies refer to delivery of model species from hydrogel systems and subsequently across buccal mucosa ( Fig. 2 ). This is a reasonable approximation to the in vivo situation.

2.2.1
Hydrogel sample preparation: single systems
2.2.1.1
PVA system
HEPES buffered saline (100 ml, pH 7.4) was heated (above 90 °C) for 45 min. PVA powder (1 g) was added to give a concentration of 1% (w/v). The mixture was stirred continuously (15 min. at 90 °C) to obtain a homogenous aqueous solution. After 24 h at room temperature (RT), the solution was cross-linked with 100 μl of 0.2% (v/v) glutaraldehyde (2 ml in 100 ml HEPES, pH 7.4), forming a transparent gel.
2.2.1.2
HPMC system
A similar method for the PVA system was followed but with HEPES buffered saline (75 ml, pH 7.4). Cold buffer (25 ml) was added after stirring to ensure complete dissolution. Following cooling (RT) the solution was stored at 4 °C for a further 24 h until gelation occurred.
2.2.1.3
PVA/HPMC 1:1 binary system
The binary system consisted of PVA and HPMC hydrogels, mixed in 1:1 ratio. The polymer mixtures were prepared separately as described above. The prepared PVA and HPMC solutions (50 ml each) were mixed and stirred to obtain a homogenous aqueous gel.
PVA, HPMC or binary system (1 g) was transferred to a polytetrafluoroethylene (PTFE) mold (7 mm diameter, 1.5 mm thick), to form a stable gel at RT (24 h). In the case of PVA, the hydrogel disks were washed thoroughly with distilled water to remove the glutaraldehyde residue, and dried at RT for 6 h.
2.2.2
Hydrogel sample preparation: sandwich systems
The methods described for the three single systems were followed but with the addition of the model active species solution (1 ml and 0.5 ml for binary system) prior to: (i) cross-linking for PVA; (ii) addition of cold buffer for HPMC; (iii) mixing 50 ml of each hydrogel for binary system.
The concentration of the three model agents in all systems was 1 mg/ml.
2.2.3
Permeability studies
The permeation studies were performed as described previously , using a 7 mm diameter Teflon flow-through chamber (“diffusion chamber”; Crown Glass Company, USA).
2.3
Single system studies: hydrogels/tissue
The buccal mucosa (thawed) or hydrogel samples (containing no model agents) were sandwiched between the donor and receptor compartments of the diffusion chamber and checked for leakage. Fluorescently labeled probes were applied to the epithelial/hydrogel surface in the donor chamber ( Fig. 1 ); the receptor compartment was kept completely immersed in HEPES buffer (30 ml receptor solution, pH 7.4) in a petri dish.
2.3.1
Passive diffusion
Samples of receptor solution (1 ml) were collected at predetermined time intervals up to 36 h and analysed by UV/vis spectroscopy (UV4 Spectrometer with a Vision software version 3.42, ATI Unicam Ltd., Cambridge, UK; wavelengths ranging from 190 nm to 900 nm).
2.3.2
Iontophoresis
Iontophoretic delivery was assessed using silver/silver chloride electrodes connected to a Phoresor ® II auto system power supply (Phoresor-850; Iomed Inc., Salt Lake City, USA). All three probes were anionic at pH 7.4, and so the cathode was either suspended in the donor solution containing model agent , either without touching the epithelium, or in direct contact with the hydrogel disks (∼3 mm in depth), while the anode electrode was suspended in the receptor solution. A constant electric current of 0.1 mA was applied. The potential difference ( V , volts) across the diffusion cell was measured at the beginning, mid-point and end of each experiment using a digital voltmeter (Maintask Ltd., UK); electric parameter ( ν ) was also measured. Samples (1 ml) were withdrawn from the receptor chamber at predetermined time intervals up to 8 h and analysed by UV/vis spectroscopy (described above).
2.3.3
Sandwich system studies
2.3.3.1
Passive/iontophoretic delivery
A similar method to passive/iontophoresis diffusion for single systems was followed but with the buccal mucosa secured in the diffusion cell and the hydrogel disk containing the active species placed on top. Buffer solution was placed in the donor chamber to cover the hydrogel/buccal mucosa ( Fig. 2 ).
Passive diffusion and buccal mucosa as a single membrane were used as controls. A total number of nine replicates per cell were used for each active species.
2.4
Analysis of data
2.4.1
Passive diffusion data
The resulting plots were characterized by the time lag, τ . The diffusion coefficient ( D ), solubility ( C 0 ) and the permeability coefficient ( P ) for the four permeants were calculated as previously described .
2.4.2
Iontophoresis diffusion data
In the iontophoretic mode TLF and ER were determined for the permeants .
2.4.3
Theoretical aspects – iontophoresis of single systems
The theoretical basis of iontophoresis was first established by Keister and Kasting and has been described earlier . From this the relevant equations are:
∂ C ∂ t = D ∂ 2 C ∂ x 2 + v L ⋅ ∂ C ∂ x
where C is the concentration of the permeant, D its diffusion coefficient, L is the sample thickness, and
v = z F V R T
where z is the valency of the ion, F the Faraday constant (96485 Coulombs × mol −1 ), and R the universal gas constant (8.3144 J K −1 ), T = temperature in K, and V the applied voltage.
Eq. (1) was derived on the assumption of a uniform electric field across the specimen and was solved using the Laplace Transform method to obtain C as a function of x and t (i.e. coordinates x , t ; Fig. 1 ). Hence the boundary conditions were:
-
model agent concentration on bottom surface of delivery system = 0; C ( x , t ) = 0 at x = L
-
model agent concentration on top surface of delivery system = C 0 ; C ( x , t ) = C 0 at x = 0
-
and: C ( x , t ) = 0 at t = 0
From the equation for C ( x , t ), the flux ( F ) at x = L is given by:
F ( L , t ) = D ∂ C ∂ x x = L
Finally Q ( t ),the quantity of permeant exiting at x = L as a function of time is given by:
Q = A D C 0 e − v L ( 1 − e − v ) t − L 2 / 2 D v 2 ⋅ Coth v 2 − 1 ( v / 2 ) 2
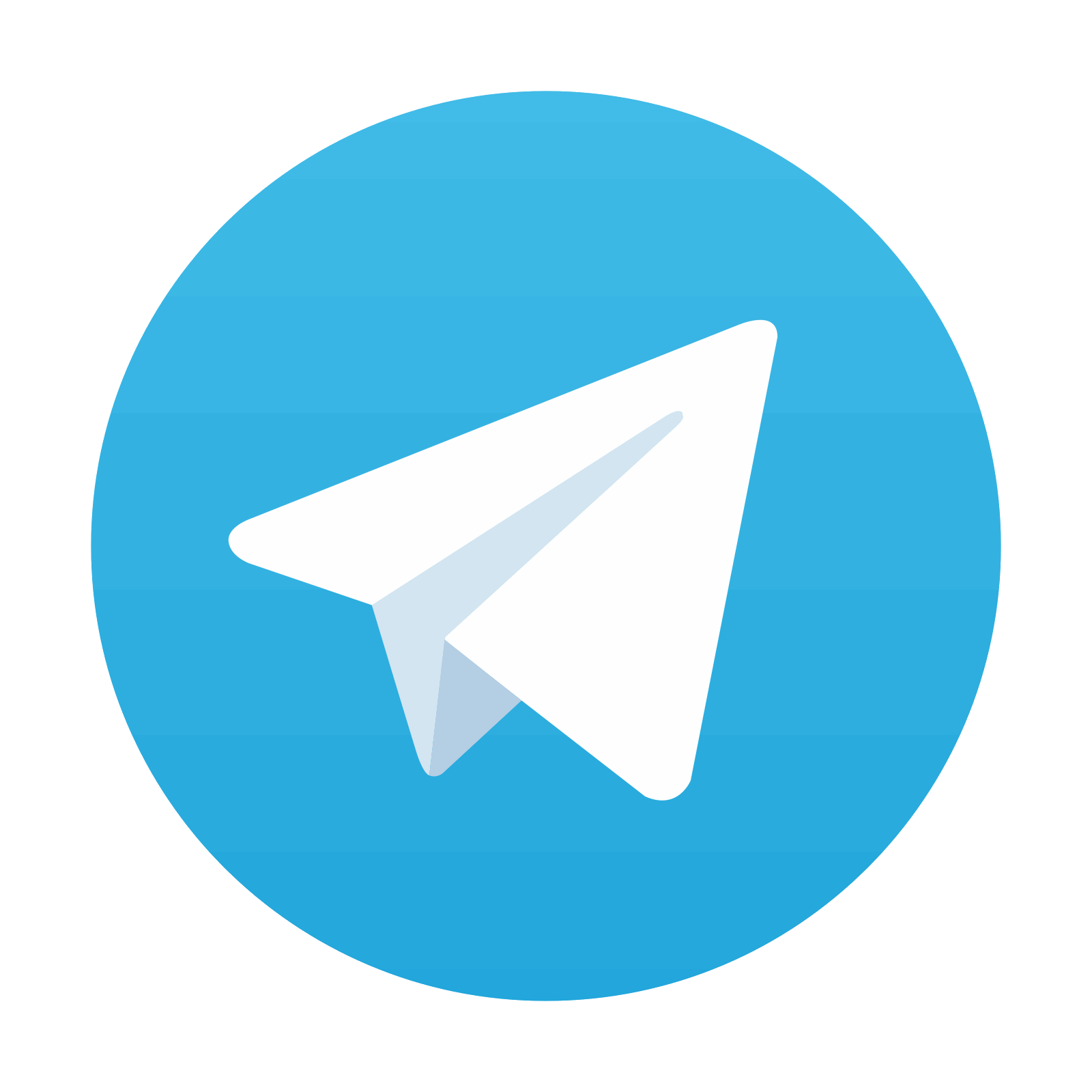
Stay updated, free dental videos. Join our Telegram channel

VIDEdental - Online dental courses
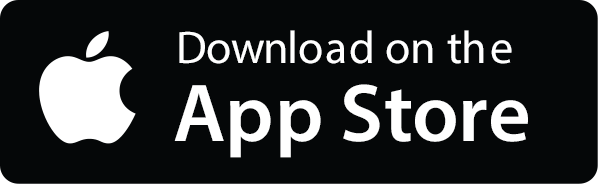
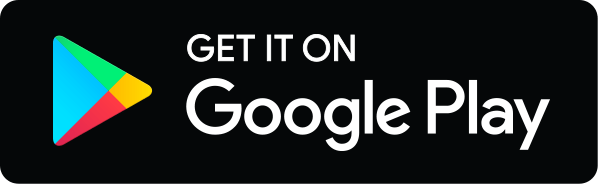