In this chapter:
■ Requirements for restorative materials
■ Overview of current materials for fixed restorations
■ Conclusions
In the past, material selection in fixed prosthodontics was mainly based on metal-ceramics and on a few all-ceramic alternatives. Metal-ceramic restorations were selected in clinical situations with need for high stability (eg, in the posterior region or in the case of multiple-unit fixed dental prostheses), whereas all-ceramic restorations were recommended in single tooth replacement with high esthetic demands, especially in the anterior region. These materials were traditionally processed by manual fabrication technologies such as casting, pressing, or layering1,2. Restorative dentistry with all-ceramic restorations has suffered from a prolonged learning curve. Several of the early systems disappeared shortly after being introduced due to an unacceptable number of mechanical failures3.
Nowadays, clinicians and technicians can choose from a wide range of reliable materials. Digital technologies such as intraoral optical scans and computer-aided design/computer-aided manufacturing (CAD/CAM) procedures have opened up new treatment pathways in fixed prosthodontics. New digital fabrication workflows were defined and in parallel advanced materials were developed and adjusted to the specific requirements of numerically controlled processing such as high-strength ceramics and composites. In these digital workflows, the restorations are fabricated by means of computer-aided milling from prefabricated blanks, increasingly replacing conventional manual processing.
The different materials available today exhibit differences in properties, influencing the esthetics and the long-term performance of the restorations. As multiple alternatives exist for each clinical situation, it is more difficult to select the most appropriate material for the respective clinical situation today than in the past4–6. As a consequence of the transformation in present technology, selection of the restorative material requires understanding of the interaction between material properties and clinical performance7.
After an introduction to the requirements for restorative materials and the behavior of the different material classes used in dentistry, this Chapter will provide an overview of the current material options for fixed restorations and their clinically relevant properties, indications, and limitations.
1.1.2 Requirements for restorative materials
In the oral cavity, restorative materials have to meet three requirements: biocompatibility, longevity, and esthetics.
Biocompatibility
The term biocompatibility implies that the material shall do no harm to the living tissues, achieved through chemical and biological inertness8. As every material potentially dilutes or degrades depending on the environment, the extent of decomposition, and the quality and amount of released substances determine the degree of biological complications. A possible host response might be localized or systemic toxicity, hypersensitivity, or genotoxicity9. The restriction to biocompatible components strongly limits the room for the development of new materials.
Due to the strict regulations for medical devices, manufacturers have to prove biocompatibility of their materials. International standards help the choosing of the appropriate tests and in interpreting the results. Tests must be done with every novel material prior to approval. Biological tests are employed in a sequence, ending up with animal tests9. Furthermore, manufacturers of medical devices are forced by law to perform a systematic post market surveillance of the materials and devices. Measures have to be taken to minimize risk and unexpected side effects must be notified to the authorities. Fortunately, it can be concluded that biological and immunological adverse reactions attributed to dental materials are rare and the reported adverse effects are acceptable9.
On the other hand it is unrealistic to assume that absolute material inertness is attainable and biological behavior is definitely predictable by means of biological tests10. Hence, the biocompatibility of dental materials must always be weighed against their benefit11. Controlled clinical trials are currently still the best way to assess the clinical response to materials. But even these tests have significant limitations. Therefore, practice-based research networks and practitioner databases are increasingly considered as a valuable alternative10.
Longevity
The long-term success of a restoration mainly depends on its mechanical performance. From the technical side the success of a restoration can be controlled by the durability of the material, the nature of the design, the quality of the processing, and the effectiveness of the finishing.
Material
The mechanical behavior of dental materials is mainly characterized by elasticity, flexural strength, fracture toughness, and hardness. These properties are basically given by the type and strength of the bondings between the atoms.
Elasticity is the ability of the material to resume its initial shape after loading, measured in GPa (= 103 N/mm2). Stressing a material beyond its limit of elasticity leads to plastic deformation, a permanent distortion. Brittle materials such as ceramics only show minimal or no plasticity, which means they fracture very soon after reaching the limit of elasticity. The stress where fracture occurs is the flexural strength, measured in MPa (= N/mm2). The resistance against crack growth is called fracture toughness, measured in MPa√m.
Elasticity, flexural strength, and fracture toughness are bulk properties. Hardness in contrast is a surface property, which is defined as the resistance to localized deformation induced by mechanical indentation or abrasion. Harder materials therefore show less risk of surface damage. Flexural strength and hardness are correlated to a certain extent.
The main risk for mechanical failure of restorations are flaws at the surface, which might act as a starting point for microcracks. In case of tensile loading, a microcrack opens and stress develops at the tip of the crack. Stress which exceeds the strength of the material leads to crack propagation. Under cyclic loading − such as mastication − crack growth happens in a micrometer scale. But over time the crack grows significantly. Finally, catastrophic failure occurs when the residual cross-section is too small to withstand the load.
It is important to understand the fracture mechanisms of the different materials. In metals the crack tip is rounded out by plastic flow and thus the risk of fracture is significantly reduced (Fig 1-1-1). In ceramics plastic flow is not possible due to the covalent bonds. The crack tip remains sharp and crack growth is a significantly higher risk than in metals. That is the reason for the well-known brittle behavior of ceramics. To increase strength and in particular toughness, strengthening mechanisms on the microscopic level to impede crack propagation are employed. In brittle materials this might be achieved by internal compression or by particles, which act as obstacles against crack growth (Fig 1-1-2). The objective of such strengthening mechanisms is to stop crack growth or at least to hamper it, like a hurdler who is not as fast as a sprinter.
Figs 1-1-1 Schematic representation of crack propagation in materials. (a) Plastic material (eg, metals). (b) Brittle material (eg, ceramics).
Fig 1-1-2 Schematic representation of crack propagation in particle-reinforced materials under tensile stress (red arrows). When the crack tip strikes a particle, crack propagation is impeded, or at least decelerated.
The term durability includes not only the mechanical characteristics specified above but resistance to wear and aging as well. The degradation of the materials by wear and aging depends on the mechanical properties and also on the susceptibility to the oral environment including humidity, temperature, and loading characteristics. Water for instance may attack the material’s bonds especially at phase boundaries or microcracks, thus promoting degradation.
Design
Several mistakes can be made when designing a restoration. Insufficient dimensioning in crown walls or connectors of fixed dental prostheses is one reason for failures. Instructions of the manufacturers have to be strictly followed. Further, sharp edges increase the risk of failure due to an uncontrolled stress development (Fig 1-1-3). And finally, restorations made by materials, which require a thermal treatment should be designed with an even wall thickness as far as possible to get a homogeneous stress distribution during cooling. That applies especially for veneering ceramics, which must be layered in a uniform thickness and adequately supported by the framework both for metal-ceramic and all-ceramic bilayers.
Figs 1-1-3a to 1-1-3d Insufficient thickness of the crown and sharp edges of the preparation caused fracture of the restoration. (a) Restoration on tooth 47 after cementation. (b) Radiograph after cementation. The insufficient occlusal thickness of the restoration and the sharp edge of the distal preparation are obvious. (c) Fracture of the restoration after 1 year in function. (d) Analysis of wall thickness on the basis of the CAD design.
Processing
A shaping process always requires machining, a thermal treatment such as sintering or pressing or a polymerization process. If not processed properly, defects might be created in the material, thus reducing the strength of the restoration (Fig 1-1-4). The manufacturer’s instructions must be meticulously followed.
Figs 1-1-4a to 1-1-4c Fractured zirconia framework 42 x x 32. (a) Framework after sintering, fracture occurred between 41 and 31. (b) Light microscopy image of the fractured area. The area was cut in the white state in order to separate the two pontics. Thus a crack was initiated, which was not sealed during sintering. (c) Scanning electron microscopy (SEM) of the fractured surface after sintering. The formation of grains at the surface indicates that the fracture occurred before sintering.
Finishing
Materials, if machined, sintered, pressed, or polymerized, must be finished with material specific tools and appropriate speed, feed, and pressure of the tools to avoid damage at the surface. For ceramics, as an alternative a glaze firing (a heat treatment without additional application of glaze) or glazing (a heat treatment with additional application of glaze) can be performed (Fig 1-1-5). However, if the restoration is not handled in a way appropriate to the material, it might occur that subsurface damage is not sufficiently eliminated by the finishing procedure and residual flaws potentially act as an origin for microcracks.
Figs 1-1-5a to 1-1-5d Schematic representation of the effect of polishing, glaze firing, or glazing on the surface quality. (a) Microcracks at the surface after processing. (b) Surface after polishing. (c) Surface after glaze firing. (d) Surface after glazing.
Esthetics
Materials for restoring teeth have to mimic the esthetic appearance of the tooth itself. The tooth is a complex structure of a dentin core, providing the color of the tooth, and a more translucent enamel layer. The replacement of dental hard tissue by a dental material needs to balance color, translucency, refraction and reflection, opalescence, and fluorescence. Some materials show a blending quality, also named the “chameleon effect.” These requirements strongly restrict the choice of materials to ceramics and resins. As a compromise metals may be used when covered by tooth-colored veneers.
Color
Coloring of resins and ceramics is obtained by using inorganic pigments, mostly metal oxides (Fig 1-1-6).
Fig 1-1-6 Pigments used to produce the appropriate shades.
Translucency
When there is no light absorption and no optical obstacle in the material, light passes through a material like a windowpane without being scattered. This effect is called translucency (Fig 1-1-7).
Figs 1-1-7a and 1-1-7b Translucency of different ceramic shades. (a) Dentin layer. (b) Enamel layer.
Refraction and reflection
When light passes through an interface and enters a different material, eg, from air to glass, the direction of light propagation is changed, which is called refraction. Depending on the incidence angle, light might also be completely reflected as if hitting a mirror (Fig 1-1-8). These effects lead to a scattering of the light. Interfaces in a material (ie, particles incorporated for strengthening) add to the optical properties by scattering the light as well (Fig 1-1-9).
Fig 1-1-8 Reflection of light at the ceramic surface. Depending on the surface roughness and the incidence angle, reflection is more or less pronounced.
Figs 1-1-9a to 1-1-9f Refraction of light in a glass-ceramic (Vita Suprinity PC) before and after crystallization. (a and b) Schematic representation of light refraction. In the glassy state (a) the material is translucent. Light passes through the material without being refracted. After crystallization (b) light is scattered at the interfaces between glass matrix and crystals. The light is partially refracted and the material thus appears whitish. The surface is slightly etched with hydrofluoric acid to demonstrate the transition from the glassy state to the typical microstructure of glass-ceramic characterized by a glass matrix and incorporated crystals. (c and d) Microstructure before (c) and after (d) crystallization. (e and f) Appearance before (e) and after (f) crystallization.
Diffraction and opalescence
At obstacles smaller than the wavelength, the light will be refracted and scattered in all directions. By diffraction white light is split into the spectral colors. The short blue wavelength will be more deflected than the long red one. If the light source is behind the observer, mainly the blue light is seen; if the light source is behind the object mainly yellow and red colors are seen (Fig 1-1-10). The effect is visible in the sky: small water drops scatter the light. If the sun is in front of us, we mainly see yellow and red light; if the sun is behind us, we can see the azure blue sky.
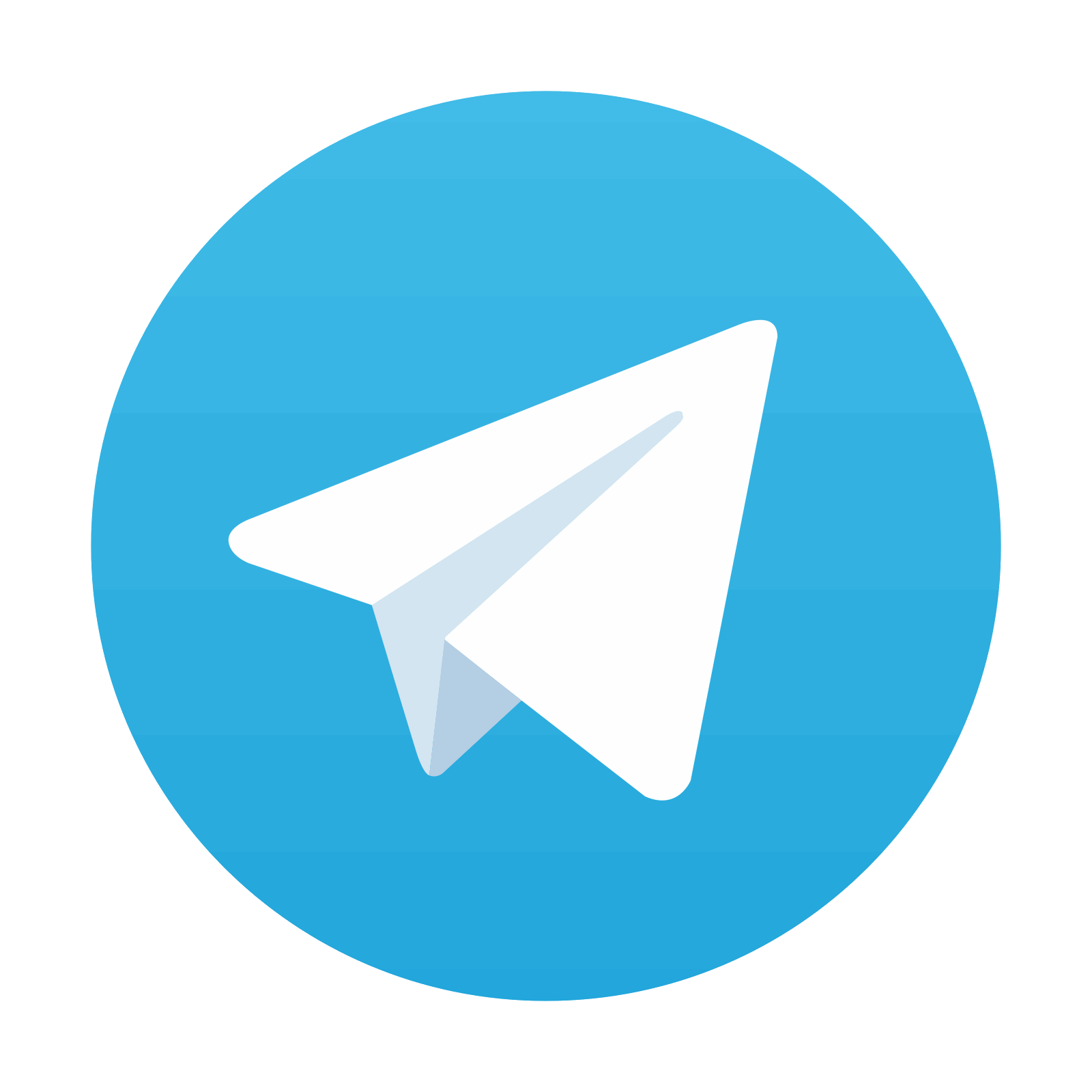
Stay updated, free dental videos. Join our Telegram channel

VIDEdental - Online dental courses
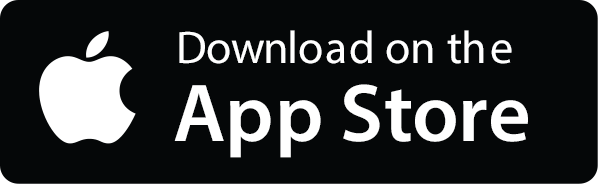
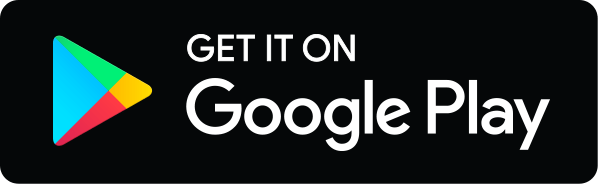