Abstract
Objectives
To determine the creep deformation of several “bulk-fill” resin-composite formulations in comparison with some other types.
Methods
Six resin-composites; four bulk-fill and two conventional were investigated. Stainless steel split molds (4 mm × 6 mm) were used to prepare cylindrical specimens for creep testing. Specimens were thoroughly irradiated with 650 mW cm −2 . A total of 10 specimens for each material were divided into two groups ( n = 5) according to the storage condition; Group A stored dry at 37 °C for 24 h and Group B stored in distilled water at 37 °C in an incubator for 24 h. Each specimen was loaded (20 MPa) for 2 h and unloaded for 2 h. The strain deformation was recorded continuously for 4 h. Statistical analysis was performed using a two-way ANOVA followed by one-way ANOVA and the Bonferroni post hoc test at a significance level of a = 0.05.
Results
The maximum creep strain % ranged from 0.72% up to 1.55% for Group A and the range for Group B increased from 0.79% up to 1.80% due to water sorption. Also, the permanent set ranged from 0.14% up to 0.47% for Group A and from 0.20% up to 0.59% for Group B. Dependent on the material and storage condition, the percentage of creep strain recovery ranged between 64% and 81%. Increased filler loading in the bulk-fill materials decreased the creep strain magnitude.
Significance
Creep deformation of all studied resin-composites increased with wet storage. The “bulk-fill” composites exhibited an acceptable creep deformation and within the range exhibited by other resin-composites.
1
Introduction
Performing a trouble-free direct composite restoration with care and in a reasonable time is a valuable technique that a dentist must have if he or she desires a satisfying and successful practice . Incremental layering has long been accepted as a standard technique for placement of resin-composite in cavity preparations. Typically, this technique consists of placing increments of resin-composite material in thickness of 2 mm or less followed by exposure to light curing from an occlusal direction and then repeating increments until the preparation is filled .
One obvious advantage for this technique is the limitation of the thickness of resin to be penetrated by light, as it has been shown that light energy transmitted through resin-composite decreases exponentially with resin-composite thickness . Therefore, limiting increment thickness to 2 mm or less provides adequate light penetration and subsequent polymerization. Adequate polymerization results in enhanced physical properties , improved marginal adaptation and decreased cytotoxicity of the resin-composite. Inadequate polymerization may result in reduced conversion of the various components of the resin-composite and therefore a higher residual monomer content remains in the material . This residual monomer, in addition to other drawbacks in the materials like poor mechanical properties , increases the cytotoxicity of the resin-composite .
A second reason to use the incremental technique is to decrease the amount of shrinkage occurring during polymerization. The reduction of polymerization shrinkage is beneficial because the developing stress can cause cuspal deformation with resulting sensitivity or microcracks in resin or tooth structure. The stress can also cause adhesive failure at the tooth/resin interface resulting in marginal gaps, microleakage, and secondary caries .
Despite these benefits, the incremental technique has disadvantages. These may include (i) the possibility of incorporating voids or contamination between composite layers, (ii) bond failures between increments, (iii) difficulty in placement because of limited access in conservative preparations, and (iv) the increased time required to place and polymerize each layer . In addition, as the bonding and filling steps are the most crucial, perfect isolation during these steps must be maintained to guarantee a successful restoration. However, when the patient salivates and moves the tongue constantly during the placement of composite layers, this could potentially compromise the accuracy of the filling. Every additional increment increases the likelihood of failure . As reported by Blank and Latta , techniques for internally stratified direct resin restorations are highly dependent on the clinician’s skills, and care must be taken to ensure that the exact thickness and shape of each layer is accurately predicted.
Recent studies have suggested that fewer increments and even bulk-filling could be equally successful . Moreover, some clinicians challenge the concept that the incremental-fill technique produces improved results in the restoration and use instead a bulk placement method in which the entire preparation is filled in one increment and is then exposed to light . However, concern about the results or the lack of a suitable bulk-fill material has discouraged most clinicians from implementing such techniques .
Lately, there is a direction to decrease the number of increments for direct composite restoration and encourage the use of a bulk-fill technique. Several manufacturers have developed “bulk-fill” resin-composites that can be applied to the cavity in thickness of 4 mm with enhanced curing and controlled shrinkage . Consequently, dentists can save themselves and their patients’ significant chairside time, and make the restorative process less stressful and more comfortable.
Several authors recommended that the “bulk-fill” composite materials must possess some important characteristics. These include low polymerization shrinkage, more flowability to allow for better cavity adaptation, easy to dispense with minimal handling, enhanced physical characteristics and improved depth of cure, at least 4 mm. One approach to accomplish this is by being translucent i.e., highly conducive to light transmission. The translucency of tooth-colored restorative materials is considered a crucial optical property, comparable in importance to color, as the translucency will strongly affect the appearance of the composite material . The transmission of the curing light through the composite material is mainly influenced by the size of the filler particles and the matching between the refractive indices of the resin matrix and the filler particles . Matching the refractive indices of filler and matrix is essential for improving the translucency and the optical properties of the resin-composit material . If the filler and matrix have mismatched refractive indices, the filler will increase light scattering in the resin–filler interface and produce opaque materials . The use of filler particles (≤400 nm) has many advantages. One of these advantages is the superior esthetic properties of the resin-composite due to excellent translucency . Also, the pigments can affect the light transmission and translucency of the material. In darker composite shades, there might be light scattering, reduced translucency and attenuation of the curing light which may result in inadequate polymerization .
There are a number of recent studies that evaluated different sets of conventional direct resin-composites placed with bulk-fill method and compared it with the conventional incremental method. This evaluation included polymerization shrinkage , hardness , microleakage , marginal adaptation and interfacial stresses . However, these studies compared conventional hybrid composites used in bulk or in increments. The introduction of resin-composites specifically intended for bulk-fill use renews the argument. These materials need to be thoroughly evaluated and the claims made about their performance substantiated.
As reported by many authors , the creep deformation is a significant aspect of the mechanical performance of polymer-based materials. It has been reported that if a resin-based material has high creep strain, this will adversely affect its resistance to mechanical stress and thus influence the long-term clinical durability of the restorations . Therefore, this study will be conducted to evaluate the creep deformation of some newly introduced resin-composites intended for bulk-fill placement. The specific objectives were to measure: (a) maximum creep strain; (b) maximum creep recovery; (c) percentage of creep recovery; and (d) permanent set for the investigated composites. The null hypotheses tested were: (i) there is no significant difference in the creep deformation and recovery behavior of the composite resins investigated; (ii) the storage condition has no effect on the creep parameters of the resin-composites.
2
Materials and methods
The materials selected in this study, lot numbers and manufacturers’ information are listed in Table 1 .
Code | Product | Type | Manufacturer | Lot no. | Resin system | Filler system |
---|---|---|---|---|---|---|
VB | Venus bulk fill | Bulk-fill | Heraeus Kulzer GmbH, Hanau, Germany | 10028 | UDMA, EBADMA | Ba–Al–F silicate glass, YbF 3 and SiO 2 (65% by wt and 38% by vol) |
SF | Surefil SDR flow | Bulk-fill | Dentsply Caulk, Milford, DE, USA | 1003011 | EBPADMA, TEGDMA | Barium/strontium-alumino-fluoro-borosilicate glass (68% by wt and 44% by vol) |
TE | Tetric EvoCeram bulk fill | Bulk-fill | Ivoclar Vivadent, Schaan, Liechtenstein | PM0213 | Dimethacrylate co-monomers | Ba glass, YbF 3 , oxides and prepolymers (80% by wt and 60% by vol) |
EX | X-tra base | Bulk-fill | Voco, Cuxhaven, Germany | V 45252 | MMA, Bis-EMA | Inorganic fillers (75% by wt and 58% by vol) |
BE | Beautiful flow plus | Flowable | Shofu Inc., Kyoto, Japan | 041008 | Bis-GMA, TEGDMA | Fluoro-borosilicate glass (67.3% by wt and 47% by vol) |
FS | Filtek supreme XTE | Nanohybrid | 3M ESPE, St. Paul, MN, USA | N214152 | Bis-GMA,UDMA, TEGDMA, PEGDMA, Bis-EMA | Zirconia/silica fillers particles (78.5% by wt and 63.3% by vol) |
Stainless steel split molds (4 mm in diameter × 6 mm in length) were used to prepare the specimens. A total of 10 specimens were prepared from each material at room temperature 23 °C. Glass microscope slides were positioned at the upper and lower surfaces of the cylindrical specimen. Each specimen was thoroughly irradiated from multiple directions. Light curing was performed using a visible light curing unit (Optilux 501, Kerr, USA) with light irradiance of 650 mW cm −2 as measured with the incorporated calibrated radiometer. A separating medium (a thin film of petroleum jelly) was placed inside and around the edges of the mold cavity to facilitate removal of the specimens. After curing, specimens were finished flush with the ends of the mold by hand-grinding with 800 grit silicon carbide paper. They were then separated from the mold to be stored. The 10 specimens for each material were divided according to the storage condition into two groups ( n = 5); Group A: stored dry at 37 °C for 24 h and Group B: stored in distilled water at 37 °C in an incubator for 24 h.
A creep measurement apparatus was used to determine the creep of the selected resin-composites ( Fig. 1 ). It consisted of a lever, which was pivoted at one end via a bearing pin, in a vertical pillar bolted to a steel U-section base . A loading pin (1 cm diameter) contained in a reduced friction bearing was located vertically and the specimen was placed on a raised platform, which was mounted in a small water bath, in an axial alignment with the loading pin, so that a force could be directed to the end faces by the application of loading weight to the free end of the lever. A linear variable displacement transducer (LVDT) (type GTX 2500; RDP Electronics, Wolverhampton, UK) was used to monitor the strain changes in the specimens in units of voltage, where the LVDT was mounted on an outrigger clamped to the loading pin. A signal from the LVDT was transferred to an analog-to-digital converter (Pico Technology, Hardwick, Cambridge, UK) and recorded via a computer data recorder (PicoLog data acquisition software).
Each specimen was subjected to a cycle of constant compressive stress of 20 MPa for 2 h followed by 2 h of strain recovery after load removal. The dimensional changes of the specimens were monitored during both stress application and its removal over a total of 4 h. Creep strain data were processed using Sigma Plot Software Version 8.0. A schematic representation of the typical constant load creep behavior is illustrated in Fig. 2 . When a polymer is loaded below its elastic limit, there is an immediate rapid elastic deformation ( R ). This is followed by a slower, time-dependent, viscoelastic deformation ( S ), which is known as creep. When the load is removed, an immediate recovery will take place ( X ). This is also followed by a further time-dependent, viscoelastic recovery ( Y ), which may or may not be complete within the given time limit of a test, and then there would be a permanent set ( Z ) . In this investigation, the following measurements were considered:
- (1)
Maximum creep strain ( R + S ).
- (2)
Maximum creep recovery ( X + Y ).
- (3)
Percentage of creep recovery ([ X + Y / R + S ] × 100).
- (4)
Permanent set ( Z ).
Data were statistically analyzed using a two-way ANOVA followed by one-way ANOVA and the Bonferroni post hoc test, at a significance level of 5%. Linear regression and correlation analysis were performed to investigate correlation between the maximum creep strain of the wet group of the “bulk-fill” composites and the filler loading.
2
Materials and methods
The materials selected in this study, lot numbers and manufacturers’ information are listed in Table 1 .
Code | Product | Type | Manufacturer | Lot no. | Resin system | Filler system |
---|---|---|---|---|---|---|
VB | Venus bulk fill | Bulk-fill | Heraeus Kulzer GmbH, Hanau, Germany | 10028 | UDMA, EBADMA | Ba–Al–F silicate glass, YbF 3 and SiO 2 (65% by wt and 38% by vol) |
SF | Surefil SDR flow | Bulk-fill | Dentsply Caulk, Milford, DE, USA | 1003011 | EBPADMA, TEGDMA | Barium/strontium-alumino-fluoro-borosilicate glass (68% by wt and 44% by vol) |
TE | Tetric EvoCeram bulk fill | Bulk-fill | Ivoclar Vivadent, Schaan, Liechtenstein | PM0213 | Dimethacrylate co-monomers | Ba glass, YbF 3 , oxides and prepolymers (80% by wt and 60% by vol) |
EX | X-tra base | Bulk-fill | Voco, Cuxhaven, Germany | V 45252 | MMA, Bis-EMA | Inorganic fillers (75% by wt and 58% by vol) |
BE | Beautiful flow plus | Flowable | Shofu Inc., Kyoto, Japan | 041008 | Bis-GMA, TEGDMA | Fluoro-borosilicate glass (67.3% by wt and 47% by vol) |
FS | Filtek supreme XTE | Nanohybrid | 3M ESPE, St. Paul, MN, USA | N214152 | Bis-GMA,UDMA, TEGDMA, PEGDMA, Bis-EMA | Zirconia/silica fillers particles (78.5% by wt and 63.3% by vol) |
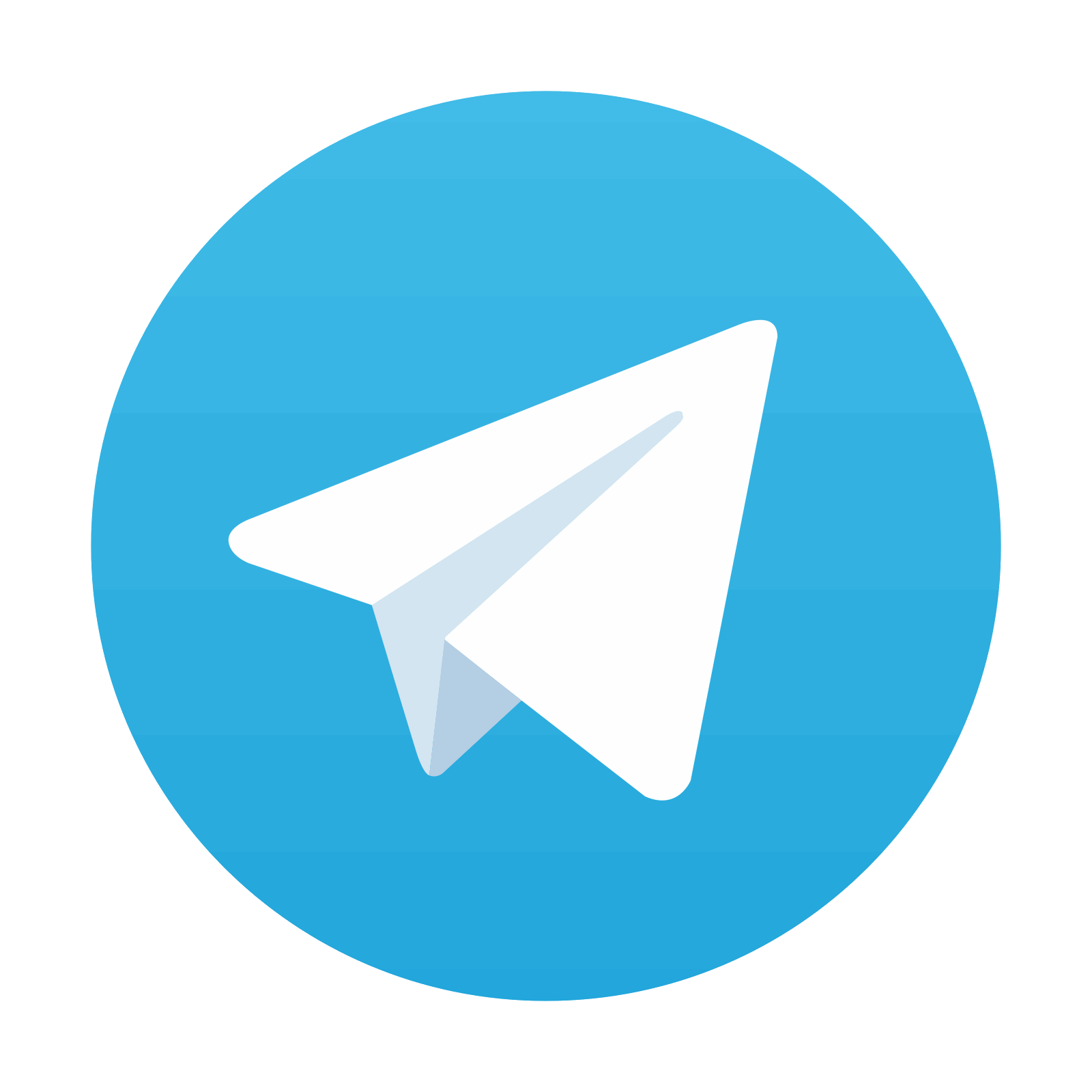
Stay updated, free dental videos. Join our Telegram channel

VIDEdental - Online dental courses
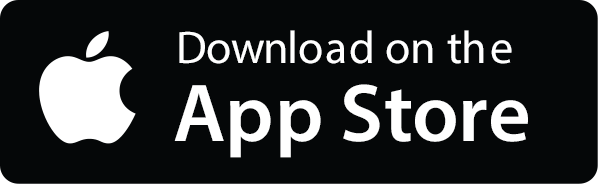
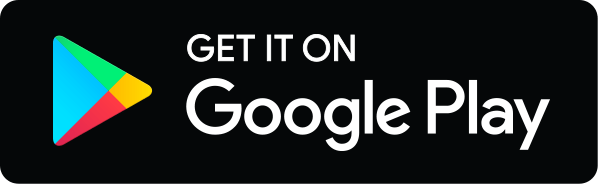
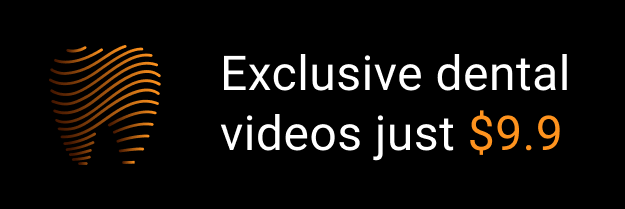