Abstract
Objective
The purpose of our study was to evaluate the effects of surface treatment on the microtensile bond strength (MTBS) of repaired silorane-based composites (Filtek Silorane (Silorane), 3M ESPE).
Methods
The surfaces of the aged Silorane blocks (6 mm × 6 mm × 5 mm) were ground with 320-grit SiC paper. The subsequent surface treatments were: no treatment, bonding agent, acetone, acetone + bonding agent, ethanol, ethanol + bonding agent, silane and silane + bonding agent. Another group made from Silorane without repair served as positive control. New Silorane was bonded onto the treated samples. The MTBS test was performed (1 mm/min) with beam-shaped sticks (1.0 mm × 1.0 mm × 10 mm) before and after fatigue simulation (FS). The Weibull moduli for the MTBS data were also calculated. The fracture modes were examined with an optical microscope. The aged specimens after grinding were observed in a SEM and the percentage of uncovered filler surface in relation to the whole surface was calculated.
Results
There were no significant differences between the no treatment group and the acetone only or ethanol only groups. Bonding agent improved the MTBS and the Weibull modulus. Although the SEM image revealed only 5.1% of uncovered filler surface, silane improved the MTBS. The groups with silane and bonding agent had the highest strength values except two groups and showed a fairly large number of cohesive failures. However, after FS, the differences between almost all groups were insignificant. FS increased the MTBS of almost all groups.
Significance
The application of silane and bonding agent enhanced the initial repair strength.
1
Introduction
Over the years, polymerization shrinkage remains as one of the major drawbacks of composite materials and leads to microleakage which is among the major factors for composite material failures in the oral environment. Silorane-based dental composite materials (Filtek Silorane, 3M ESPE, Seefeld, Germany) are designed to minimize shrinkage and polymerization stress . Silorane derives from siloxanes and oxiranes, which provide the hydrophobic and low-shrinking silorane base of Filtek Silorane. However, despite the continuing development of composite resins with improved properties, several factors, including fracture and discoloration, may still present concerns. As a result, dentists must decide whether to replace or simply repair these restorations. Complete removal of defective composite restorations is not always necessary or desirable . Repairs of composite restorations are frequently accomplished by the addition of new composite to the existing aged composite. Because aged conventional methacrylate-based composite restorations do not retain an unpolymerized surface layer (oxygen inhibition layer) , several techniques are suggested to maximize the composite repair strength. The most common suggestions include increased surface roughness , silane treatment and the application of a low viscosity bonding agent . Silorane-based composites polymerize by a cationic ring-opening process and are nearly insensitive to oxygen. If the cations are permanently present in the silorane and can be used for repair (in a process called “living polymerization”), this might offer an advantage for silorane repairs over other methacrylate-based composite repairs.
When composite materials with small average filler sizes are ground, only a small area of pure glass surfaces is evident when examined under a scanning electron microscope (SEM). This raises the question of whether the silane treatment could be omitted.
Another aspect to consider is the use of the solvent of the bonding agent such as acetone or ethanol. Solvents enhance resin surface wetting capabilities, quickly vaporize and remove water molecules from the surface . As the hydrophobicity of sirolane-based composites or bonding agents impedes penetration into the smallest crack and gaps of the water-saturated surface on the aged composite, these functions of solvents may play an important role in the repair process. Acetone also might solubilize and soften the surface . The most important function of a repair liquid is considered to be that it solubilizes the base material, penetrates it, and finally leads to chemical bonding with the repair material , reported as “liquid etching” . Therefore the application of acetone as a pretreatment may possibly increase the bonding strength. However, the softened layer may also result in reduced bond strength of the dental composite. In addition, solvents such as acetone or ethanol could act as an inhibitor of polymerization for bonding agents or composites .
The purpose of our study was to evaluate how to optimize bond strength for the repair of silorane composite materials, which are a new class of compounds for dental use.
2
Materials and methods
The materials used in this study are shown in Table 1 . The experimental design is outlined in Fig. 1 .
Brand name | Composition | Batch no. | Manufacturer |
---|---|---|---|
Filtek Silorane: shade A3 (silorane-based dental composite) | Resin: polysilorane, filler: 53 vol.%–73 wt%. | 8CN 9ET |
3M ESPE, Seefeld, Germany |
Filtek Silorane: shade B2 (silorane-based dental composite) | Resin: polysilorane, filler: 53 vol.%–73 wt%. | 8BB | 3M ESPE, Seefeld, Germany |
Silorane system adhesive bond (bonding agent) | Hydrophobic dimethacrylate, phosphorylated methacrylates, TEGDMA (triethylene glycol dimethacrylate), silane-treated silica filler, initiators, stabilizers | 8AY | 3M ESPE, Seefeld, Germany |
3M ESPE Sil (silane coupling agent) | Ethyl alcohol, 3-methacryloxypropyltrimethoxysilane, methyl ethyl ketone | 307985 364825 |
3M ESPE, Seefeld, Germany |
2.1
Preparation of aged composites
The method of preparation of aged composites is illustrated in Fig. 1 a. 60 composite resin blocks (6 mm × 6 mm × 5 mm) were prepared from a silorane-based dental composite (Filtek Silorane shade A3, 3M ESPE, Seefeld, Germany) in accordance with the manufacturer’s instructions for use in transparent vinyl polysiloxane matrices. The composite was carefully condensed with a clean filling instrument in order to avoid contamination and/or entrapment of voids. The composite blocks were bulk cured without layering with a halogen light device (Astralis 10, program: high intensity, Ivoclar Vivadent, Schaan, Liechtenstein). Irradiation was performed for 20 s on one composite surface and another five surfaces of the specimens that were in contact with the surface of the mold, in order to ensure uniform and complete polymerization. Each specimen was removed from the mold and further light-cured on each surface for 20 s.
The substrate specimens were boiled in water for 24 h to age the material. The vinyl polysiloxane mold ensured that the exposed surface was parallel to the cutting plane. One surface of each aged specimen was wet-ground (VP100, LECO, Michigan, USA) to obtain a flat surface. 320-grit silicon carbide grinding paper was used to correspond to the roughness obtained by diamond bur grinding . Then the specimens were ultrasonically cleaned for 3 min.
2.2
Bonding procedure
Specimens were randomly distributed into 10 groups and were subjected to 10 different surface treatments before the repair procedure was performed ( Fig. 1 b). Procedures for the surface treatments were as follows:
- •
Group 1: No treatment was applied to the repaired surface. This group served as the negative control group.
- •
Group 2: Silorane system bonding agent (Silorane Bond, 3M ESPE, Seefeld, Germany) was applied, air dried and cured for 20 s with the halogen light device.
- •
Group 3: Acetone (Acetone SAV, SAV LP, Feldkirchen, Germany, batch no. 0000021253) was applied for 1 min and air dried.
- •
Group 4: Acetone was applied, as described in Group 3, followed by bonding agent, as described in Group 2.
- •
Group 5: Ethanol (Silbermann Chemie + Technik, Gablingen, Germany, batch no. 260146) was applied for 1 min and air dried.
- •
Group 6: Ethanol was applied, as described in Group 5, followed by bonding agent, as described in Group 2.
- •
Group 7: Silane coupling agent (3M ESPE Sil, 3M ESPE, Seefeld, Germany, batch no. 307985) was applied for 1 min and gently air dried.
- •
Group 8: Silane coupling agent was applied, as described in Group 7, with a different batch no. of the same brand (3M ESPE Sil, batch no. 364825).
- •
Group 9: Silane coupling agent was applied, as described in Group 7, followed by bonding agent, as described in Group 2.
- •
Group 10: Silane coupling agent was applied, as described in Group 8, followed by bonding agent, as described in Group 2.
The ethanol used in Groups 5 and 6 and the silane coupling agent used in Groups 8 and 10 were prepared as blind pretreatment agents, and after microtensile testing, the composition of the blind pretreatment agents were made known.
Following surface treatment, new composite resin (Filtek Silorane, shade B2) was bonded onto the samples using the matrices (6 mm × 6 mm × 10 mm) ( Fig. 1 c). The composite resin used was the same brand in a different shade to distinguish it from the aged composite and to identify the composite–composite interface . The composite blocks were bulk cured with the halogen light device without layering. Irradiation was performed for 20 s on five surfaces of the new composite that were in contact with the surface of the mold. Each specimen was removed from the molds and further light-cured on each surface for 20 s.
2.3
Thermo-mechanical fatigue simulation
Specimens for each group were stored either in distilled water at 37 °C for 24 h or stored in distilled water at 37 °C for 24 h and then additionally thermocycled for 2000 cycles between 5 and 55 °C with a dwell time of 30 s and transfer time of 5 s ( Fig. 1 d). After thermo-cycling, each sample was mounted in a mechanical loading device (fatigue simulator Muc 2, Willytec, Munich, Germany) that was programmed with a compact processor 6303 pneumatic control unit (B & R Industrial Electronics, Eggelsberg, Austria) and an in-line display. The samples were oriented vertically with the new composite on the top and the aged composite on the bottom. Samples were placed on dies, and they were mechanically loaded for 50,000 cycles at 1 Hz with a load of 50 N in water.
2.4
Unrepaired composite resin blocks
Composite resin blocks (6 mm × 6 mm × 10 mm) were prepared as the positive control group from silorane-based dental composite resin (Filtek Silorane, shade A3) without layering. The composite blocks were bulk cured with the halogen light-curing device. Bulk curing is not the recommended curing protocol for Filetek Silorane. We decided to favor bulk curing over incremental curing because we wanted to avoid any potential influences of layering on the final strength. We tried to compensate the material thickness by extended light curing. Irradiation was performed for 20 s on one composite surface and another five surfaces of the specimens that were in contact with the surface of the mold. Each specimen was removed from the mold, and further light-cured on each side for 20 s. The specimens were stored in distilled water at 37 °C for 24 h.
2.5
Preparation of sections for bond strength test
Each specimen was secured with thermoplastic impression compound (Impression Compound Red Stick, KerrHawe, Bioggio, Switzerland) on an epoxy resin block, which was mounted on a cutting machine (Isomet Low Speed Saw, Buehler, Lake Bluff, IL, USA) ( Fig. 1 e). By means of a water-cooled diamond blade, each specimen was serially sectioned to obtain multiple beam-shaped sticks with dimensions of approximately 1.0 mm × 1.0 mm × 10 mm, according to the non-trimming technique . More than 25 sticks were prepared for each group.
2.6
Microtensile bond strength test
For microtensile testing, each specimen was glued at each end with cyanoacrylate adhesive (UHU, UHU, Bühl, Germany) to a jig designed to transmit purely tensile forces to the specimen when mounted on a tensile testing machine optimized for the microtensile test (MTD-500, SD Mechatronik, Feldkirchen-Westerham, Germany) ( Fig. 1 f). The test was performed at a cross-head speed of 1 mm/min until failure. The load at failure was recorded in N , and the specimen’s fragments were carefully removed from the fixtures with a scalpel blade. The cross-sectional area at the site of fracture was measured to the nearest 0.01 mm with a digital caliper (Mitutoyo ID-U1025, Mitutoyo, Kawasaki, Japan) in order to calculate the bond strength at failure in MPa.
Comparative analysis of the microtensile bond strength was carried out using one-way ANOVA at a significance level of p < 0.05. The data with and without fatigue simulation were separately evaluated with one-way ANOVA. Significant differences between the groups within the same one-way ANOVA test were further analyzed using Scheffé’s method (significance level of p < 0.05). The Weibull moduli ( m ) for the microtensile bond strength data were also calculated. The Weibull modulus is a measure of variability of the results and is found by linear regression on a plot of ln ln(1/(1 − failure probability)) against ln(microtensile bond strength).
The fractured specimens were examined visually with an optical microscope (Stemi SV11, Zeiss, Jena, Germany) at 50× magnification to determine the macroscopic failure modes, which were classified as cohesive (within either the aged or the new composite resin), adhesive or mixed.
2.7
Scanning electron microscope (SEM) analysis
The aged specimens without any surface treatment were polished with 320-grit silicon carbide grinding paper, rinsed with distilled water, air-dried and sputter-coated with gold (Polaron Range SC7620, Quorum Technologies, West Sussex, United Kingdom). The specimens were observed in the SEM (SUPRA 55VP, Zeiss, Oberkochen, Germany) at an accelerating voltage of 10 kV and a magnification of 20,000×. In the digitized SEM image, the percentage of uncovered filler surface in relation to the whole surface was calculated with image processing software (ImageJ, National Institutes of Health, Maryland, USA). Firstly, the visible fillers, which were not covered by matrix, were interactively marked with the mouse tool and the marked area was automatically calculated in the program ImageJ. Secondly, the whole image was marked and the whole area was calculated in the same way. The marked area of all fillers was summarized, and the percentage relative to the whole image was calculated.
2
Materials and methods
The materials used in this study are shown in Table 1 . The experimental design is outlined in Fig. 1 .
Brand name | Composition | Batch no. | Manufacturer |
---|---|---|---|
Filtek Silorane: shade A3 (silorane-based dental composite) | Resin: polysilorane, filler: 53 vol.%–73 wt%. | 8CN 9ET |
3M ESPE, Seefeld, Germany |
Filtek Silorane: shade B2 (silorane-based dental composite) | Resin: polysilorane, filler: 53 vol.%–73 wt%. | 8BB | 3M ESPE, Seefeld, Germany |
Silorane system adhesive bond (bonding agent) | Hydrophobic dimethacrylate, phosphorylated methacrylates, TEGDMA (triethylene glycol dimethacrylate), silane-treated silica filler, initiators, stabilizers | 8AY | 3M ESPE, Seefeld, Germany |
3M ESPE Sil (silane coupling agent) | Ethyl alcohol, 3-methacryloxypropyltrimethoxysilane, methyl ethyl ketone | 307985 364825 |
3M ESPE, Seefeld, Germany |
2.1
Preparation of aged composites
The method of preparation of aged composites is illustrated in Fig. 1 a. 60 composite resin blocks (6 mm × 6 mm × 5 mm) were prepared from a silorane-based dental composite (Filtek Silorane shade A3, 3M ESPE, Seefeld, Germany) in accordance with the manufacturer’s instructions for use in transparent vinyl polysiloxane matrices. The composite was carefully condensed with a clean filling instrument in order to avoid contamination and/or entrapment of voids. The composite blocks were bulk cured without layering with a halogen light device (Astralis 10, program: high intensity, Ivoclar Vivadent, Schaan, Liechtenstein). Irradiation was performed for 20 s on one composite surface and another five surfaces of the specimens that were in contact with the surface of the mold, in order to ensure uniform and complete polymerization. Each specimen was removed from the mold and further light-cured on each surface for 20 s.
The substrate specimens were boiled in water for 24 h to age the material. The vinyl polysiloxane mold ensured that the exposed surface was parallel to the cutting plane. One surface of each aged specimen was wet-ground (VP100, LECO, Michigan, USA) to obtain a flat surface. 320-grit silicon carbide grinding paper was used to correspond to the roughness obtained by diamond bur grinding . Then the specimens were ultrasonically cleaned for 3 min.
2.2
Bonding procedure
Specimens were randomly distributed into 10 groups and were subjected to 10 different surface treatments before the repair procedure was performed ( Fig. 1 b). Procedures for the surface treatments were as follows:
- •
Group 1: No treatment was applied to the repaired surface. This group served as the negative control group.
- •
Group 2: Silorane system bonding agent (Silorane Bond, 3M ESPE, Seefeld, Germany) was applied, air dried and cured for 20 s with the halogen light device.
- •
Group 3: Acetone (Acetone SAV, SAV LP, Feldkirchen, Germany, batch no. 0000021253) was applied for 1 min and air dried.
- •
Group 4: Acetone was applied, as described in Group 3, followed by bonding agent, as described in Group 2.
- •
Group 5: Ethanol (Silbermann Chemie + Technik, Gablingen, Germany, batch no. 260146) was applied for 1 min and air dried.
- •
Group 6: Ethanol was applied, as described in Group 5, followed by bonding agent, as described in Group 2.
- •
Group 7: Silane coupling agent (3M ESPE Sil, 3M ESPE, Seefeld, Germany, batch no. 307985) was applied for 1 min and gently air dried.
- •
Group 8: Silane coupling agent was applied, as described in Group 7, with a different batch no. of the same brand (3M ESPE Sil, batch no. 364825).
- •
Group 9: Silane coupling agent was applied, as described in Group 7, followed by bonding agent, as described in Group 2.
- •
Group 10: Silane coupling agent was applied, as described in Group 8, followed by bonding agent, as described in Group 2.
The ethanol used in Groups 5 and 6 and the silane coupling agent used in Groups 8 and 10 were prepared as blind pretreatment agents, and after microtensile testing, the composition of the blind pretreatment agents were made known.
Following surface treatment, new composite resin (Filtek Silorane, shade B2) was bonded onto the samples using the matrices (6 mm × 6 mm × 10 mm) ( Fig. 1 c). The composite resin used was the same brand in a different shade to distinguish it from the aged composite and to identify the composite–composite interface . The composite blocks were bulk cured with the halogen light device without layering. Irradiation was performed for 20 s on five surfaces of the new composite that were in contact with the surface of the mold. Each specimen was removed from the molds and further light-cured on each surface for 20 s.
2.3
Thermo-mechanical fatigue simulation
Specimens for each group were stored either in distilled water at 37 °C for 24 h or stored in distilled water at 37 °C for 24 h and then additionally thermocycled for 2000 cycles between 5 and 55 °C with a dwell time of 30 s and transfer time of 5 s ( Fig. 1 d). After thermo-cycling, each sample was mounted in a mechanical loading device (fatigue simulator Muc 2, Willytec, Munich, Germany) that was programmed with a compact processor 6303 pneumatic control unit (B & R Industrial Electronics, Eggelsberg, Austria) and an in-line display. The samples were oriented vertically with the new composite on the top and the aged composite on the bottom. Samples were placed on dies, and they were mechanically loaded for 50,000 cycles at 1 Hz with a load of 50 N in water.
2.4
Unrepaired composite resin blocks
Composite resin blocks (6 mm × 6 mm × 10 mm) were prepared as the positive control group from silorane-based dental composite resin (Filtek Silorane, shade A3) without layering. The composite blocks were bulk cured with the halogen light-curing device. Bulk curing is not the recommended curing protocol for Filetek Silorane. We decided to favor bulk curing over incremental curing because we wanted to avoid any potential influences of layering on the final strength. We tried to compensate the material thickness by extended light curing. Irradiation was performed for 20 s on one composite surface and another five surfaces of the specimens that were in contact with the surface of the mold. Each specimen was removed from the mold, and further light-cured on each side for 20 s. The specimens were stored in distilled water at 37 °C for 24 h.
2.5
Preparation of sections for bond strength test
Each specimen was secured with thermoplastic impression compound (Impression Compound Red Stick, KerrHawe, Bioggio, Switzerland) on an epoxy resin block, which was mounted on a cutting machine (Isomet Low Speed Saw, Buehler, Lake Bluff, IL, USA) ( Fig. 1 e). By means of a water-cooled diamond blade, each specimen was serially sectioned to obtain multiple beam-shaped sticks with dimensions of approximately 1.0 mm × 1.0 mm × 10 mm, according to the non-trimming technique . More than 25 sticks were prepared for each group.
2.6
Microtensile bond strength test
For microtensile testing, each specimen was glued at each end with cyanoacrylate adhesive (UHU, UHU, Bühl, Germany) to a jig designed to transmit purely tensile forces to the specimen when mounted on a tensile testing machine optimized for the microtensile test (MTD-500, SD Mechatronik, Feldkirchen-Westerham, Germany) ( Fig. 1 f). The test was performed at a cross-head speed of 1 mm/min until failure. The load at failure was recorded in N , and the specimen’s fragments were carefully removed from the fixtures with a scalpel blade. The cross-sectional area at the site of fracture was measured to the nearest 0.01 mm with a digital caliper (Mitutoyo ID-U1025, Mitutoyo, Kawasaki, Japan) in order to calculate the bond strength at failure in MPa.
Comparative analysis of the microtensile bond strength was carried out using one-way ANOVA at a significance level of p < 0.05. The data with and without fatigue simulation were separately evaluated with one-way ANOVA. Significant differences between the groups within the same one-way ANOVA test were further analyzed using Scheffé’s method (significance level of p < 0.05). The Weibull moduli ( m ) for the microtensile bond strength data were also calculated. The Weibull modulus is a measure of variability of the results and is found by linear regression on a plot of ln ln(1/(1 − failure probability)) against ln(microtensile bond strength).
The fractured specimens were examined visually with an optical microscope (Stemi SV11, Zeiss, Jena, Germany) at 50× magnification to determine the macroscopic failure modes, which were classified as cohesive (within either the aged or the new composite resin), adhesive or mixed.
2.7
Scanning electron microscope (SEM) analysis
The aged specimens without any surface treatment were polished with 320-grit silicon carbide grinding paper, rinsed with distilled water, air-dried and sputter-coated with gold (Polaron Range SC7620, Quorum Technologies, West Sussex, United Kingdom). The specimens were observed in the SEM (SUPRA 55VP, Zeiss, Oberkochen, Germany) at an accelerating voltage of 10 kV and a magnification of 20,000×. In the digitized SEM image, the percentage of uncovered filler surface in relation to the whole surface was calculated with image processing software (ImageJ, National Institutes of Health, Maryland, USA). Firstly, the visible fillers, which were not covered by matrix, were interactively marked with the mouse tool and the marked area was automatically calculated in the program ImageJ. Secondly, the whole image was marked and the whole area was calculated in the same way. The marked area of all fillers was summarized, and the percentage relative to the whole image was calculated.
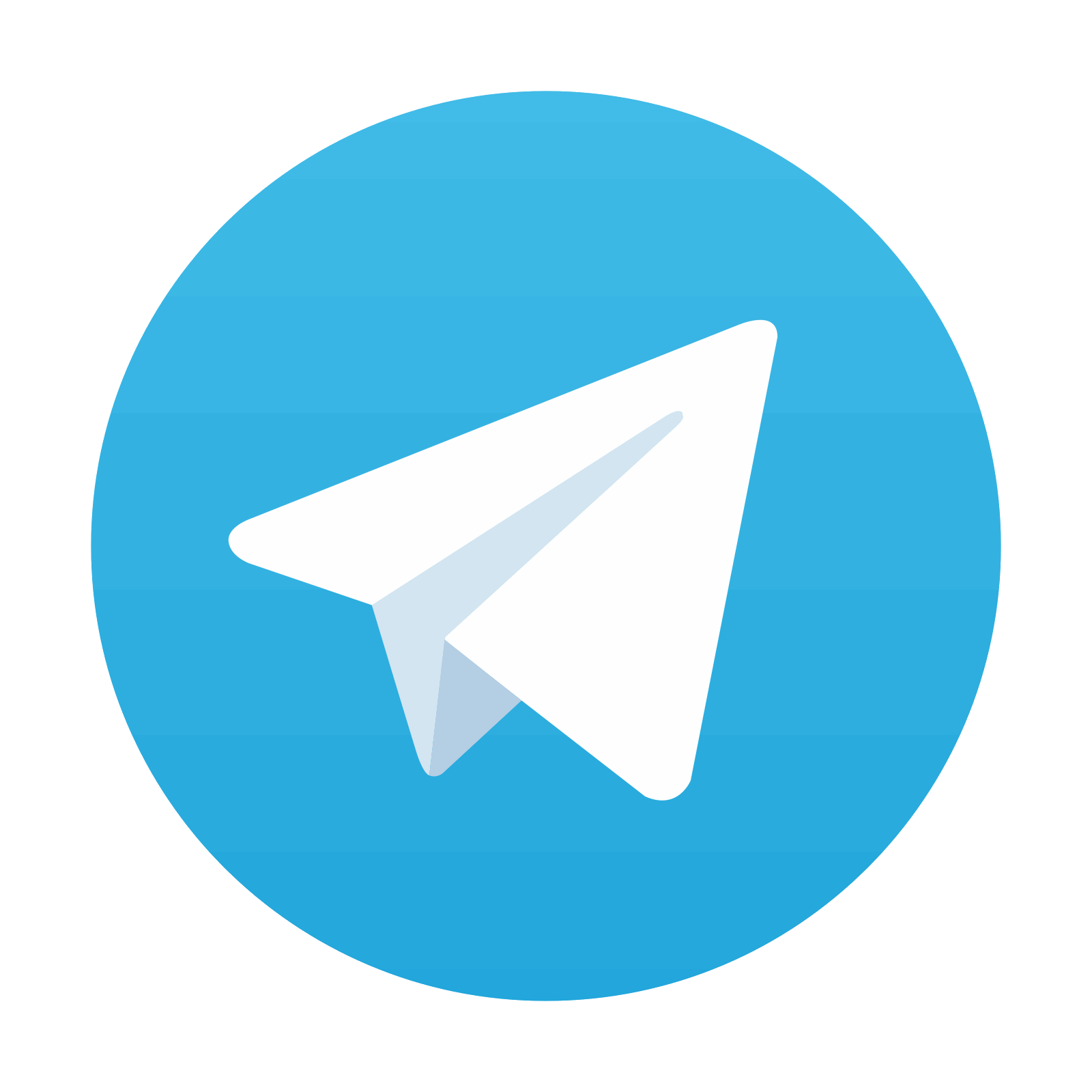
Stay updated, free dental videos. Join our Telegram channel

VIDEdental - Online dental courses
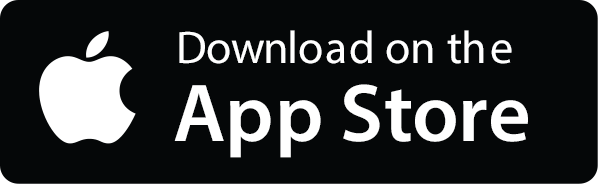
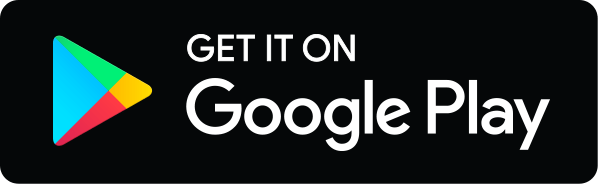