Craniofacial growth and development
Birgit Thilander
Key topics
- Developmental periods and growth in height (stature)
- Standards of growth and development
- Prenatal development
- Postnatal growth and development of the craniofacial complex
- Growth of the nasomaxillary complex
- Growth of the mandible
- Development of the dentoalveolar complex
- Eruption of teeth and development of the dental arches and occlusion
- Development of the dental arches and occlusion
Learning objectives
- To understand the definitions and standards of growth
- To understand prenatal development
- To understand postnatal growth and development of the craniofacial complex
- To understand the development of the dentoalveolar complex
- To understand and have knowledge of the eruption mechanisms of the teeth
Introduction
The fully developed cranium represents the sum of its separate parts, in which growth is highly differentiated and occurs at different rates and in different directions, and is a very complex concept. Knowledge of the normal process provides a basis for correct diagnosis of a malocclusion and is consequently a prerequisite for optimal orthodontic treatment in the individual case. Changes in the soft tissue profile with age follow the growth in the underlying hard tissues and are of importance in treatment planning, due to aesthetics.
The development and growth of the skull comprise phenomena falling within the scope of several disciplines and occurring at several levels. No specific method can therefore be assigned to the study of craniofacial growth. However, both experimental and clinical methods have been applied. Twin studies have been used to elucidate the importance of heredity and environmental factors. Biometric methods (longitudinal, semi-longitudinal or cross-sectional types) are suited for dimensional changes during growth. X-ray cephalometry is an often-used method for longitudinal follow-ups, and our information on human craniofacial growth is to a large extent based on that technique. The most commonly-used methods are experiments on laboratory animals to explain how cranial growth is regulated by studying specific events at the cell and tissue level by using histological, biochemical and histochemical techniques. In recent years, studies at the molecular level have additionally increased our knowledge of bone biology. The outcome from all these methods forms the basis for modern knowledge of craniofacial growth, which can be divided into four components:
- growth mechanism (how new bone is formed);
- growth pattern (change in size and shape of the bone);
- growth rate (speed at which the bone is formed); and
- the regulation mechanism, which initiates and directs those three processes.
Definitions of general growth
Growth, in a biological sense, is usually defined as an increase in size or weight of a tissue, an organ or an individual, and can be described quantitatively by diagrams or curves. The change in growth intensity over time is obvious when expressed as a velocity or rate curve (Figure 3.1), as used to describe the pubertal growth spurt in adolescence. The growth rate differs markedly from one organ or tissue system, even showing periods of regression. Growth in a tissue is genetically controlled but is also influenced by other factors, for example race, sex, nutrition and state of health.

Figure 3.1 Mean growth in body height and velocity for Swedish boys and girls from 5 years of age to 31 years. Girls – dotted lines.
Growth is often used as a synonymous for development which, in a biological sense, is defined as any process of continuous changes, and is therefore a broader term involving both quantitative and qualitative changes. The term ‘differentiation’ is used to describe development from a homogenous entity to increased complexity and specialisation at the cellular and tissue level, which are prerequisites of morpho-differentiation, i.e. the development in shape and size that determines the morphology of organs, systems and the whole body. Disturbance of this process may result in congenital defects.
The stage that the individual has reached in the process of development is usually described by the term ‘maturation’. It is a very broad term, but usually refers to a specific organ or functional system, and is described in relation to specific features. Physical maturation may thus be described in terms of developmental age, which often does not coincide with chronological age. The great individual variation in growth due to genetic, social and nutritional factors makes developmental age a more suitable measure of development than chronological age.
Developmental periods and growth in height (stature)
Based on the average fluctuation in growth and maturation, the following developmental periods up to adulthood are generally recognized. Each of them is characterised by special growth and developmental features, but there are no sharp boundaries between them.
The prenatal stage is classified into ovum period (1st week), embryonic period (2nd–7th weeks) and foetal period (8th–40th weeks). The growth rate, largely determined by maternal factors, is low in the embryo. The foetus, however, has a high growth factor while undergoing morphological differentiation, especially during the 5th month. The weight gain per unit time, on the other hand, is greatest towards the end of the foetal period, and at birth the child will be about 50 to 52 cm long with a weight of round 3,500 g.
During the postnatal stage, the growth rate is related to the individual’s genetic background, and is usually divided into the following four different periods:
- In the infantile period – (1–12 months after birth) the rate of growth declines considerably as the effect of foetal growth fades. A simple rule of thumb is that the child’s height at the age of 2 years is about a half of its final height in adulthood.
- The juvenile period – (early childhood at the age of 1–6 years and late childhood at the age of 7–10 years) is characterized by relatively slow growth with a decline in rate towards a pre-puberty minimum, which in Scandinavia occurs at the age of about 10.5 years in girls and 11.5 years in boys. There are great variations, however, and the range for the pre-puberty minimum is held to be as much as 4 years. A pre-puberty growth acceleration even occurs between 6 and 8 years of age.
- The puberty – (early adolescence) features a growth spurt with a peak height of velocity (PHV) at about 12.5 years in girls and about 14 years in boys. The individual variation in the rate and timing of this peak is again about 4 years for both sexes. Girls reach their peak earlier than boys. The growth rate declines after PHV at about the same speed as during the infantile period (Figure 3.1).
- Growth gradually ceases during late adolescence and is generally completed by the age of 20 years, which is the point in time when growth in stature is less than 0.5 cm in 2 years and adulthood is reached.
Standards of growth and development
To estimate the individual growth and maturation, standards or criteria of normality are needed, to which individual deviations can be related. Standards of growth and development are normally given as mean values obtained in studies of representative groups from a population whose variation is assumed to lie within the normal range. Normal growth in a child should therefore be determined by the fact that it falls within the range of variation of the child’s potential growth in its social environment.
The dentition as well as the jaws will show large variation in developmental level in a group of children, for example classmates, when related to chronological age, which provides only a rough estimate of developmental level. In order to judge deviations from a normal pattern, this variation has to be considered in orthodontic diagnosis and treatment planning. It is of the greatest importance to estimate the biological age of the child, which involves the following different types of developmental ages:
- Morphological age – is a comparison of attained size (height and weight) to normative standards. There is a close correlation between the growth spurt in skeletal height (stature) and various facial dimensions, while it has been proposed that longitudinal recording of body height is a useful aid for the planning of orthodontic treatment. A factor of general interest is the secular trend in mean height in Scandinavian schoolchildren during the 20th century (Brundtland et al., 1980).
- Skeletal age – provides a record of the developmental age of the growing skeleton. Skeletal maturation follows a predetermined pattern, since some bones mature faster than others, and can be assessed by X-raying the hand and wrist. Maturity stages based on hand X-rays can be useful complements to body height measurements and lateral head X-rays.
- Dental age – may also be used to describe physical maturity. While sexual age and skeletal age are closely related in normal growth, while dental and skeletal maturity show weak correlation. Several systems have been developed to estimate the dental age from radiographs (Demirjian and Godstein, 1976). The dental age is above all used in estimating the age of a child with unknown birth date and in forensic medicine.
- Dental stage – is a term introduced by Björk et al. (1964). Instead of age, the children are grouped according to their tooth emergence status. Dental stages are very useful in clinical work, both in diagnosis and in treatment planning.
Prenatal development of the face and jaws
A good knowledge of prenatal development is necessary for a proper understanding of the postnatal growth and the pathogenesis of congenital facial malformations. The germ layers, which form the basis of the embryogenesis of the different organ systems, are:
- The endoderm – gives rise to the epithelial lining in the posterior part of the oral cavity and the entire digestive system, from the root of the tongue downwards.
- The ectoderm – gives rise to the skin and related structures (hair, nails and sweat glands), nervous system, nasal epithelium, the epithelial lining of the anterior part of the oral cavity, and the tooth enamel.
- The mesoderm – gives rise to the mesenchyme (embryonic connective tissue), differentiating into connective tissue, skeleton and smooth muscles (except in the skin), blood and lymphatic tissues.
- The ectomesenchyme – is often described as a fourth germ layer. It derives from the neural crest cells, a population of cells that arises at the boundary of the embryonic neural folds during formation of the neural tube. They separate from the ectoderm on the 21st to 22nd day, after which they undergo intensive migration sub-ectodermally. They give rise to a variety of structures in the facial region, including skeletal and dental tissues. As these migrating cells control normal growth along their paths, deviations from this development can occur at various times and stages in neural crest formation, differentiation and morphogenesis (Ten Cate, 1980).
In the early embryonic period, the germ disc becomes increasingly curved, especially in its cranial and caudal parts where head and tail are established (Figure 3.2). At the same time, a swelling arises at its cephalic part, marking the development of the anterior brain vesicle, the prosencephalon. In this way, the head gains its characteristic forward bend at the vertebral column flexure during the second month. The first external sign of the formation of the sense organs can now be seen in the form of epithelial lens and otic placodes. The head of the foetus develops rapidly from now on, and by the end of the embryonic period, it shows the external features of a human being. During the latter part of this period, a cartilaginous skeleton of the head, the chondrocranium, develops, followed by the first ossification centres in the face, the jaws and the cranial base.

Figure 3.2 At the top, the mesoderm on each side of the neural tube splits into segments (somites) in an embryo at 4 weeks. At the bottom is seen a mid-sagittal section with the primitive oral cavity.
Development of the face and jaws
The cephalic part of a 3-week embryo (3 mm) is dominated by the procencephalon. Above is a depression (stomatodeum) forming the primitive oral cavity (Figure 3.2). On each side of this depression, the branchial or pharyngeal arches start to develop, with bulges on the ectodermal surface and pouches on the endodermal surface. The primitive oral cavity will by this time be surrounded by the first branchial arch (the mandibular arch) and the small maxillary processes developing bilaterally from its dorsal portions. The stomatodeum deepens further, but is still separated from the foregut by the bucco-pharyngeal membrane, which is made up of an outer ectodermal layer towards the stomatodeum and an inner endodermal layer towards the primitive gut. Disintegration of the membrane at the 28th day involves connection between the stomatodeum and the foregut is established. From this it is easy to understand while the epithelia of the oral and nasal cavities and tooth enamel are of ectodermal origin, while the pharyngeal epithelium is of endodermal origin.
The nasomaxillary complex takes shape as a consequence of continuing differentiation and growth of the ectomesenchyme via:
- the medial and lateral nasal processes from the frontal process, which in turn develops from procencephalon; and
- the two maxillary processes, developing as separate entities from the dorsal portions of the 1st branchial arch (Figure 3.3).

Figure 3.3 Drawing of the roof of the stomatodeum.
The medial process grows caudally to join the anteriorly and medially processes, resulting in an incomplete roof to the mouth (the primary palate). The medial nasal process forms the central part of the nose and the central part of the upper lip. The roof of the mouth thus is a horseshoe-shaped structure with its anterior part formed by the primary palate and its lateral boundaries by the maxillary processes. There remains considerable confusion regarding the mechanism of the formation of the primary palate, but evidently a small part is initially formed by an epithelial invagination, whereas the major part is formed by coalescence of the facial processes, apparently through epithelial fusion and ectomesenchymal penetration for consolidation (Ferguson, 1991). Clefts in the primary palate may thus result from failure at various stages of these processes. The 6th week is critical for these clefts (Figure 3.4).

Figure 3.4 A child with a cleft lip.
During the 7th week, two tissue folds (palatal shelves), developing from the maxillary processes, grow in vertical–anterior directions on each side of the developing tongue (Figure 3.5a). About a week later they rise into a horizontal position above the tongue (Figure 3.5b). Fusion of the shelves proceeds in a posterior direction from the 8th to the 12th week (Figure 3.5c). Establishment of the secondary palate thus includes two determinative events: reorientation of the palatal shelves and their subsequent fusion in the midline. Failure in this synchronism involves defective closure and results in a cleft. Combined cleft lip and palate (CLP) may occur uni- or bilaterally (Figure 3.6).

Figure 3.5 Drawings of different stages in development of the secondary palate, anterior section.

Figure 3.6 A palatal cleft (a), a unilateral CLP (b), a bilateral CLP (c).
The 1st branchial arch makes up the mandible during the embryonic period, with a cartilage rod (Meckel’s cartilage) acting as the primary skeleton for the lower face. This cartilage later retrogresses, except dorsally, where it remains as a ligament and as a precursor to the auditory ossification of the ear. In the 6th to 7th weeks, parts of the ossified mandibular corpus can be seen in the shape of thin bone plates in the area of the mental foramen and lateral to Meckel’s cartilage. The ossified corpus and ramus of the mandible are formed by expansion anteriorly and posteriorly, and will remain as a paired structure throughout the foetal period.
Between the 10th and 12th weeks, the ectomesenchyme differentiates into a secondary cartilage in the condylar area, extending cranially and inferiorly towards the expanding mandibular body. The temporomandibular joint (TMJ) has in principle assumed its final form by the end of the 4th month. When compared with other synovial joints in the body, it is late in forming, which may be explained by its origin as a secondary cartilage, while the regulating mechanism of synovial joint development is of muscular origin.
Ossification of the craniofacial skeleton
The first sign of skeletal development is an increased density in the ectomesenchyme in the 2nd foetal month, which differentiates into a hyaline cartilage skeleton, the chondrocranium, mainly comprising the anterior part of the cranial base and the nasal capsule. Several ossification centres appear in these structures, from which the large part of the chondrocranium is ossified during the foetal period. Remnants persist as cartilaginous joints, synchondroses. At the same time, several ossification centres develop in the facial region and, somewhat later, in the soft tissue membrane around the brain. These ossification centres rapidly expand to form the facial skeleton and vault of the cranium, the desmocranium. When intramembraneously formed bones meet, sutures develop.
Facial muscles
The participation of the myotomes (differentiated from the somites) in the development of the muscles in the head region is a subject of controversy. Out of the total of 42 to 44 pairs of myotomes, only 4 (the occipital myotomes) are established in the cephalic region, and one of them regresses. The facial muscles are thought to develop from the ectomesenchyme of the pharyngeal arches and the differentiation to form the separate muscles is extremely complex, as it is characterised by an intense migration in many directions. The muscles of mastication develop from the 1st pharyngeal arch (innervated by the Vth cranial nerve), whereas the mimic muscles differentiate from the 2nd pharyngeal arch (innervated by the VII cranial nerve). Despite their migration, the separate muscles and nerves supplying them remain intimately associated throughout the developmental period. Neuromuscular activity in the orofacial muscles has been observed as early as during the 3rd foetal month.
The anterior part of the tongue develops from the mandibular arch and the posterior part of the second, third, and part of the fourth pharyngeal arches. Establishing the tongue muscles starts during the 7th week, but their origin is uncertain. Comparative anatomical studies and the innervation data indicate that the occipital myotomes participate in its ontogenesis. According to another theory, the muscles of the tongue develop directly from the mesenchyme and are not related to the myotomes. This would explain why so many nerves are involved in its innervation (V, VII, IX and XII cranial nerves).
Postnatal growth and development of the craniofacial complex
At the time of birth, the craniofacial skeleton has undergone almost half of its total growth and the head makes up about a quarter of the body height, whereas in adults it is about one-eighth of the body height. Although this reflects the early development of the final size of the head compared with the rest of the body, the remaining dimensional increase is not equal in all parts of the cranium. While the size of the cerebral cranium will increase by about 50%, the facial skeleton will grow to more than twice the size (Figure 3.7). This difference in proportions is associated with the early development of the brain, whose growth is practically completed by the age of 4 years.

Figure 3.7 Diagram illustrating the proportions in body height at different ages. Note the relation between the head and the body at the different ages.
The facial skeleton increases in all dimensions, that of height being the greatest (∼200%), that of depth somewhat smaller (∼150%), and that of width the smallest (∼75%). Facial width is one of the first three dimensions to reach normal size, and the facial skeleton therefore becomes steadily longer and narrower from birth to adulthood.
Ethnic differences in facial traits exist, and the dentofacial pattern will change during periods of active growth, as has been reported in many studies of cephalometric norms or standards for individuals of varying ethnic groups and ages. This can be illustrated by superimposed tracings from individuals with normal (ideal) occlusion, followed from 5 years up to 31 years of age (Thilander et al., 2005) (Figure 3.8). As can be seen, facial pattern changes exist during the observation period, with growth acceleration between the 13 and 16 years recordings. Obvious changes are noted also in the period between 5 and 7 years of age as well as between 16 and 31 years, and changes in adults older than that have been reported (Bondevik, 2012). Thus, a sound knowledge of growth and development in ‘normal’ individuals are essential in diagnosing each malocclusion. Growth and development of the different parts in the dentofacial entity (cranial base, nasomaxillay complex, and mandible) form the basis for a detailed diagnosis (differential diagnosis) and the treatment planning in patients with any type of malocclusions.

Figure 3.8 Superimposed tracings from individuals of 5 to 31 years of age and with normal occlusion.
Mechanism of bone growth
Bone grows by two fundamental physiologic processes; modelling and remodelling. Modelling is a surface-specific activity that shapes and changes the size and shape of the bone, while remodelling occurs in the bone tissues as reconstruction of bone by turnover of previous osseous tissue (Haversian system) and regulation at the molecular level. The process we are dealing with in facial morphogenesis is growth remodelling, i.e. both modelling and remodelling are included in this term.
Bone growth in the craniofacial complex is under genetic control and is influenced by epigenetic and environmental factors. Developmentally, bone formation occurs either by endochondral ossification with a cartilage model, or by a direct intramembranous formation. Although the starting processes are different, once formed, bones of both origins are modelled and remodelled in the same way. The skeleton is a dynamic and complex mineralized connective tissue with high capacity to adapt to various mechanical and physiological requirements, in which osteoclastic bone resorption and osteoblastic bone formation are highly coordinated. The periosteum is of greatest significance in this process and hence for the change in size and shape of the bones (growth pattern).
The inner cambium layer of the periosteum provides the mesenchymal stem cells and osteoprogenitor cells, required for growth and development of the bone (Roberts et al., 2015). Mesenchymal stem cells are recruited from the circulation, bone marrow or the periosteum to the site for new bone formation. The commitment of mesenchymal stem cells to osteoproginator cells and ultimately to differentiated osteoblasts is regulated by several factors, which are bound to specific receptors, for example bone morphogenetic proteins (BMPs), belonging to the transforming growth factor-ß (TGFß) family, and fibroblast growth factors (FGFs) (Capulli et al., 2014). The fully differentiated osteoblast is characterized by alkaline phosphatase activity and production of proteins, for example collagen type I and more bone-specific non-collagenous proteins. Osteoblasts are seen either at the bone surface or within lacunae in the newly-formed matrix (Figure 3.9). They occur as active cuboidal cells or as inactive flat bone-lining cells (Figure 3.9).

Figure 3.9 Illustration of relocation (drift) of the maxillary palate. Photomicrograph showing cuboidal cells at the resorption area (a), periosteal resorption on the nasal side and deposition on the oral side (b), and photomicrograph showing flat bone lining cells at the deposition area (c).
The remodelling cycle starts with osteoclastic resorption and is subsequently followed by new bone formation at the same site, which occurs in coordinated functional basic multicellular units. The coupling mechanisms between osteoclastic bone resorption and the following bone formation are still not clear.
Osteoclasts are the only cells which can resorb mineralized tissues. New osteoclasts are actively recruited from hematopoietic stem cells of the monocyte-macrophage lineage when a resorption process is to start. The mononuclear osteoclast precursors fuse to form multinucleated cells with a short life span. Osteoclasts adhere tightly to the bone surface and form a sealing zone, in which they, via a specialized ruffled border of the cell membrane, release proteolytic enzymes and hydrogen ions to degrade and resorb the organic and inorganic components of the bone. The activation of the osteoclast is crucially dependent on macrophage colony stimulating factor (M-CSF) and receptor activator of nuclear factor kappa-B ligand (RANKL), which is produced by osteoblasts in response to various factors, for example hormones, prostaglandins (PG) and pro-inflammatory cytokines. It has been recently discovered that osteocytes produce large amounts of RANKL and thus support osteoclastic bone resorption.
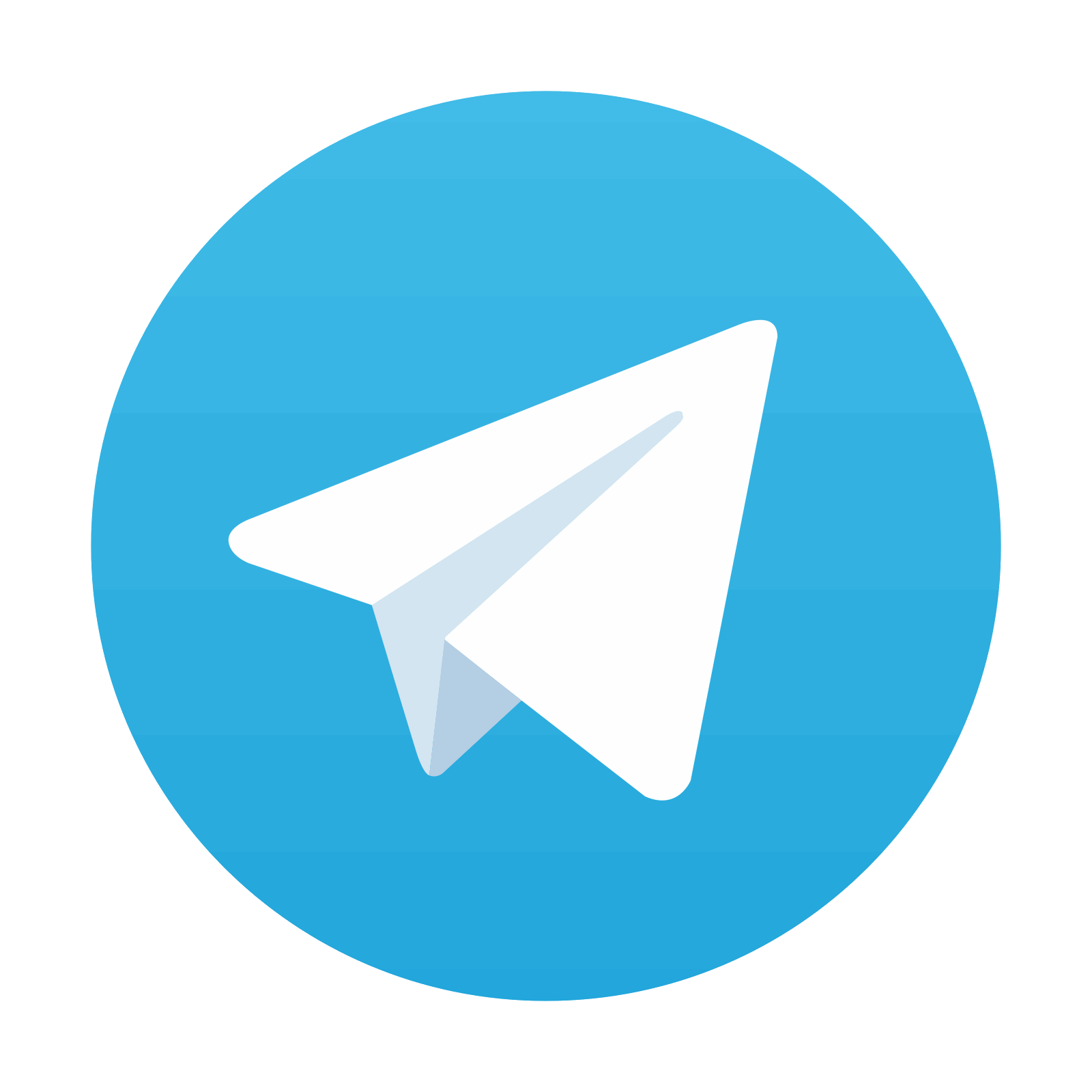
Stay updated, free dental videos. Join our Telegram channel

VIDEdental - Online dental courses
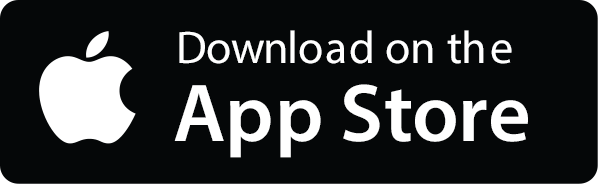
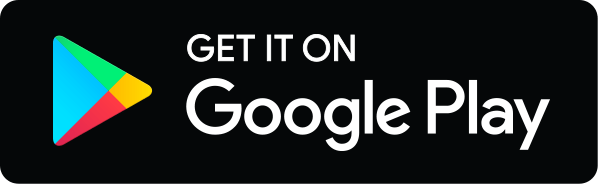