Introduction
The purposes of this study were to determine factors favoring successful mini-implant placement and to evaluate root proximity as a possible risk factor for failure of osseointegration-based mini-implants during orthodontic treatment.
Methods
Three-dimensional cone-beam computed tomography images were used to examine 50 sandblasted, large-grit, and acid-etched surface-treated mini-implants (C-implant, Seoul, Korea) placed in 25 patients. The images were analyzed for 3-dimensional position of the mini-implant (placement angle and depth) and any contact with root surfaces or maxillary sinuses.
Results
There were no remarkable differences in horizontal placement angles in the axial plane and placement depths of the mini-implants, but the vertical placement angle was significantly higher on the left side (24.5° ± 11.0°) compared with the right side (11.8° ± 11.6°). The horizontal mini-implant placement angle had a greater inclination tendency toward the maxillary first molar, and 11 mini-implants with root proximity showed mesiobuccal contact with the maxillary first molar root. Only 1 failure in 15 mini-implants with root proximity and 1 failure in 35 without root proximity were observed on the images.
Conclusions
Root proximity alone was not considered a major risk factor for osseointegration-based mini-implant failure.
Achieving a high success rate for mini-implant placements is critical in the integration of temporary anchorage devices into clinical orthodontic treatment. Higher success rates improve the reliability of anchorage stability in planning orthodontic biomechanics, obtain better treatment results, prevent unexpected side effects that delay treatment, and avoid the use of complex appliances. For mini-implants, the usual placement site is the interradicular space between the maxillary second premolar and first molar, since it has the widest space between adjacent roots in the buccal maxillary area.
Several authors have developed and suggested new surgical guide techniques for precise mini-implant placement in interradicular spaces. Choi et al suggested using a custom-made wire guide for preventing root damage. Estelita et al also developed a modified radiographic positioner for ensuring exact transfer between the periapical radiographic location and the intraoral drill trajectory position, thereby improving clinical outcomes. However, these surgical guides require additional steps involving x-rays of the patient taken at several times to check placement position. Also, at the present time, most surgical guide systems with 2-dimensional (2D) radiographs have technical limitations for reproducing the precise placement position in the 3 planes of space. If the mini-implant placement is technique sensitive because of complex anatomy, such as an expanded sinus or alveolar bone loss, a precise surgical guide fabricated by using cone-beam computed tomography (CBCT) data should be used. Despite its exceptional accuracy, a CBCT guide has some limitations: complex fabrication process, high cost, and higher radiation dose compared with 2D radiographs. Most clinicians generally place mini-implants without a surgical guide and use only panoramic radiographs or periapical films for presurgical treatment planning to estimate interradicular space. In patients who have sufficient interradicular space and no visible complex anatomic structures, it is common for clinicians to carefully place mini-implants in the predicted position with a visual reference guide and only clinical judgment without surgical guides.
Many risk factors have been identified that can affect successful placement of conventional mini-implants in interradicular spaces. Of these adverse conditions, root proximity is a major factor for screw failure in orthodontic anchorage. Recently, some authors reported that the stability of mini-implants after placement can be affected by many factors, including location and timing of force application. Liou et al advocated that mini-implants should be placed in an edentulous area if the patient does not have enough interradicular space or there is less than a 1-mm gap between the mini-implant and the root.
In addition to conventional smooth-surface mini-implants, sandblasted, large-grit, and acid-etched (SLA) mini-implants have been introduced as firm anchorage systems that achieve partial osseointegration throughout the treatment period. This mini-implant system can work as an independent orthodontic appliance to implement anchorage without assistance from posterior anchorage teeth as set up in a conventional anchorage reinforcement system. With this partial osseointegration mini-implant system, research data by Kim et al showed that these devices resisted fracture during removal even after 6 months of nonloading and that the removal torque value (RTV) required to remove the SLA mini-implant was clinically acceptable. Although many mini-implant placements have been considered successful based on clinical stability, no studies have documented root proximity and stability of osseointegration-based mini-implants.
The actual position of a mini-implant placed by a clinician using 2D radiographs without a surgical stent has not been evaluated, and there are no scientific analyses on the preferred area of root proximity for mini-implants. Therefore, the purposes of this study were to place SLA surface-treated mini-implants in the posterior maxilla by using panoramic radiographs only, to compare quantifiable parameters such as placement angles and placement depths for efficient and stable mini-implants, and to investigate correlations of root contact and maxillary sinus penetration to mini-implant failure by using a CBCT craniofacial imaging system. Additional information gained from the CBCT data will demonstrate its diagnostic usefulness in the placement of mini-implants.
Material and methods
We enrolled 25 patients (7 men, 18 women; average age, 26 years) seeking orthodontic treatment in the Department of Orthodontics, Uijeongbu St Mary’s Hospital, in Uijeongbu, Korea. They volunteered to receive orthodontic treatment with maxillary mini-implants and agreed to have a CBCT scan after mini-implant placement. They also consented to have the removal torque measured with a digital device after treatment. These patients all had mini-implants placed between the maxillary second premolar and first molar with a panoramic radiograph as the surgical planning tool and CBCT scans taken after the mini-implants were placed. There were 15 Class I malocclusion and 10 Class II malocclusion patients. No patient had severe periodontitis, severe crowding, missing teeth, or abnormality in the maxilla. One female patient ( Table I , number 16) had an impacted maxillary canine. The mean treatment time was 15.3 months.
RTV (Ncm) | ||||||
---|---|---|---|---|---|---|
Subject | Sex | Age (y) | Malocclusion Class | Treatment period (mo) | Right | Left |
1 | F | 34 | I | 15 | ||
2 | F | 16 | II | 15 | ||
3 | F | 33 | I | 13 | 10.81 | 17.92 |
4 | F | 23 | I | 15 | 9.41 | 16.55 |
5 | F | 33 | II | 14 | ||
6 | F | 20 | I | 15 | ||
7 | F | 46 | II | 13 | ||
8 | F | 20 | I | 13 | 26.56 | 22.16 |
9 | F | 42 | II | 16 | ||
10 | F | 23 | I | 14 | ||
11 | F | 40 | I | 14 | ||
12 | F | 25 | I | 14 | 20.42 | 23.38 |
13 | F | 25 | I | 14 | ||
14 | F | 23 | II | 18 | ||
15 | F | 24 | II | 17 | ||
16 | F | 18 | I | 22 | 3.94 | 13.48 |
17 | F | 27 | II | 15 | 19.86 | 32.94 |
18 | F | 25 | I | 19 | † | † |
19 | M | 20 | II | 15 | 19.20 | 12.16 |
20 | M | 23 | I | 13 | 10.44 | † |
21 | M | 25 | II | 14 | † | 28.97 |
22 | M | 24 | I | 17 | † | † |
23 | M | 18 | I | 19 | † | † |
24 | M | 23 | I | 15 | 23.47 | 44.08 |
25 | M | 30 | II | 14 | 19.70 | 23.02 |
SLA mini-implants (diameter, 1.8 mm; length, 8.5 mm; C-implant, Cimplant Company, Seoul, Korea) were used for this study ( Fig 1 ). These implants have a 2-component design consisting of an upper head part and a lower screw part. The threads have a smooth cutting edge like a conventional prosthodontic dental implant. The lower 6.5 mm is surface treated and embedded in the bone, whereas the upper 2 mm has a smooth surface in contact with the soft tissues. Since the main archwire can pass through the 0.8-mm diameter hole of the head part, this mini-implant can be used as an independent anchorage unit without fixed appliances on the posterior teeth ( Fig 2 ). The retention portion of the SLA mini-implant has a higher osseointegration potential compared with smooth titanium mini-implants and therefore better resists the rotational tendency during heavy dynamic loads. Clinically, SLA mini-implants showing complete stability after 12 months of continuous load were considered successful. All SLA mini-implants used in this study functioned as independent appliances for 3-dimensional (3D) anterior retraction and involved no posterior teeth for anchorage.
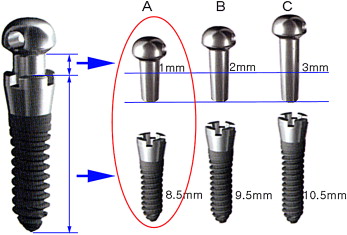
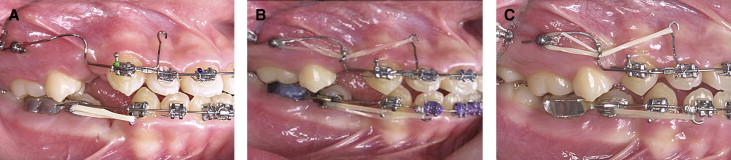
The maximum interradicular distance was measured on the panoramic radiograph by using a dental explorer, and the attached gingiva on the placement site was perforated by a tissue punch under local anesthesia to anesthetize the gingiva (less than a quarter of a 1.8-mL volume). A pilot hole was created with a 1.5-mm diameter guide drill (Stryker Leibinger, Tuttlingen, Germany). The surgical site was monitored for bleeding, and then the screw part of the mini-implant was placed. After a 4-week healing period, the head part was connected by tapping without a second surgical procedure. The detailed surgical procedure for the placement of C-implants has been given in previous articles.
The postsurgical scans were taken with a CBCT device (PSR 9000N, Asahi Roentgen, Kyoto, Japan) with dosimetry parameters of 10 mA, 80 kV, and 30-second scan time. The panoramic computed tomography mode (0.15 mm 3 voxel size) with a field of view encompassing the entire maxilla was selected. The patient was instructed to rest the chin on the machine’s chin-support cup, and the occlusal plane was aligned parallel with the positioning beam. The radiographic output was adjusted to 40-mm thickness and 36-mm width to accommodate the maxilla within the range of the positioning beam. A median beam line was used to center the face, and a head-holding rod was used to hold the head in a stationary position.
The CBCT data were saved as a digital imaging and communications in medicine (DICOM) file by using the Picture Archiving Communication System (Infinit, Seoul, Korea) at Kyung Hee University Dental Hospital. The V-works software program (version 5.0, CyberMed, Seoul, Korea) was used for analyzing the DICOM data to generate quantitative measurements.
For the frontal CBCT plane, an axial plane that passed through the mini-implant was constructed. After comparison with the facial planes that passed through the maxillary second premolar and the mini-implant, the vertical placement angles of the left and right sides ( Fig 3 , A ) were measured. The absence or presence of maxillary sinus penetration was also noted.
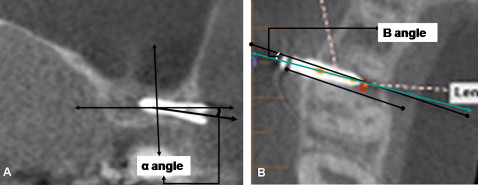
To measure the plane parallel to the long axis of the mini-implant, after orientation of the axial plane intersecting the frontal plane, the depth of cortical bone penetration was measured ( Fig 3 , B ). The horizontal mini-implant placement angle between the maxillary second premolar and first molar was measured on both sides.
To evaluate root proximity and maxillary sinus penetration with the axial and sagittal planes of the CBCT images, the proximity of the root structure to the mini-implant was checked. Sagittal and frontal plane images were used to identify any maxillary sinus penetration of the mini-implant. Root proximity was defined as contact of the mini-implant surface with the neighboring root or overlay to the periodontal ligament of the root in the CBCT images. The mini-implants in this study were divided into 4 groups according to root proximity or sinus penetration: group 1, the screw showed root proximity only on 1 side; group 2, the screw showed root proximity on both sides; group 3, the screw had no root contact but penetrated the sinus; and group 4, the screw had both root proximity and sinus penetration ( Fig 4 ).
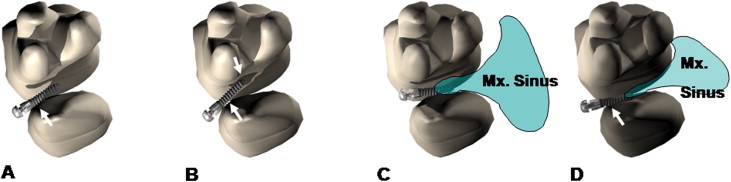
When the mini-implants were removed after treatment, RTV was measured by 2 experienced clinicians (K.S.H. and C.K.R) using an established protocol with a digital torque sensor accurate to ±0.01 Ncm (STS-31, Lorenz Messtechnik, Alfdorf, Germany; D-1700, Emobile Tech, Seoul, Korea). The peak maximum torque value was recorded as the RTV. When there was sufficient friction to inhibit separation of the upper head part, RTV was not measured. Torque values of 20 implants from 10 patients were obtained.
Statistical analysis
To test for intraoperator variability, CBCT data sets from 8 patients were randomly selected and remeasured by using the same defined parameters 2 weeks after the initial data analysis. Measurement errors were calculated with the Pearson correlation coefficient test.
Contact with root surface, placement depth of the mini-implant, and horizontal mini-implant placement angle between the maxillary second premolar and first molar in the axial CBCT plane parallel to the mini-implant’s long axis, vertical placement angles of the mini-implant in the frontal CBCT plane, and RTV after treatment were recorded and compared by using paired t tests between the left and right sides.
With the CBCT images, the relationship of root contact or maxillary sinus invasion with clinically observed mini-implant failure was compared and calculated with respect to the mean success rate.
Since there were more women in this study, no sex comparison was performed. Statistical analyses were done with SAS software (version 8.0, SAS Korea, Seoul, Korea).
Results
The raw data of age, sex, total treatment time, and RTV are presented in Table I . Mean RTV after treatment showed 19.9 Ncm with a noticeable statistically significant difference between the right and left sides (6.38 ± 7.23 Ncm on the right side vs 23.47 ± 9.74 Ncm on the left; P = 0.0812).
Eight of the 25 patient data sets in this study were randomly selected and remeasured 2 weeks later. Remeasurement results showed highly reproducible data analysis with the Pearson correlation analysis (correlation coefficient, 0.86-0.99; P <0.01).
On the frontal CBCT images, the placement angulations of the left and right mini-implants were measured and compared by using t tests. There was a statistically significant difference between the right and left vertical placement angles (α angle) ( P = 0.0002) ( Table II ). The mean vertical placement angles of the mini-implants on the frontal plane were 11.8° for the right side and 24.5° for the left side. A larger angle on the left side reflected a greater placement inclination of the mini-implant and therefore established more cortical bone contact.
Left | Right | ||||
---|---|---|---|---|---|
Variable | Mean | SD | Mean | SD | P value |
Cortical bone penetration (mm) | 6.79 | 0.49 | 6.76 | 0.50 | 0.8601 |
Placement angle (°) | |||||
α | 24.5 | 11.0 | 11.8 | 11.6 | 0.0002 ∗ |
β | 4.4 | 10.2 | 4.9 | 10.1 | 0.8479 |
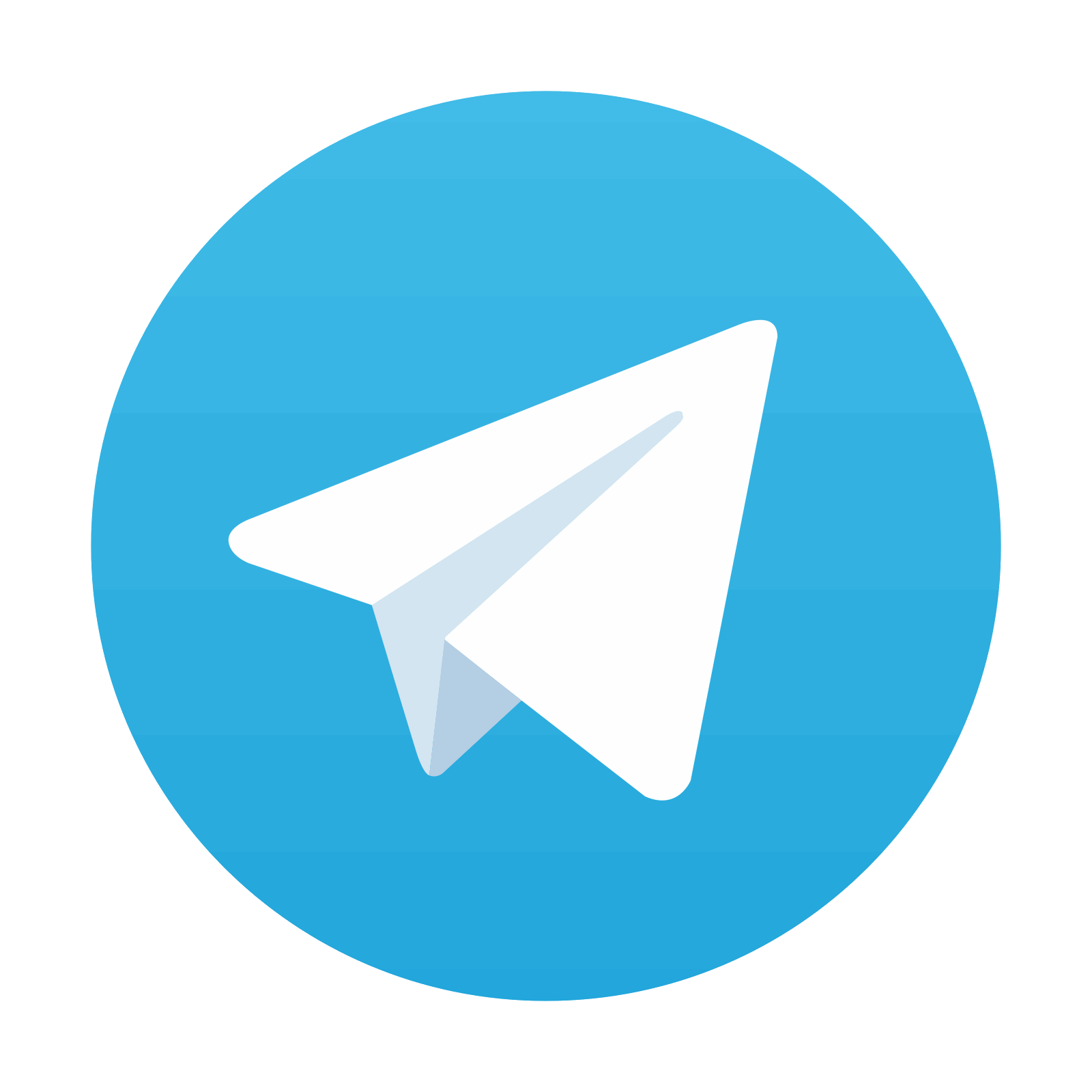
Stay updated, free dental videos. Join our Telegram channel

VIDEdental - Online dental courses
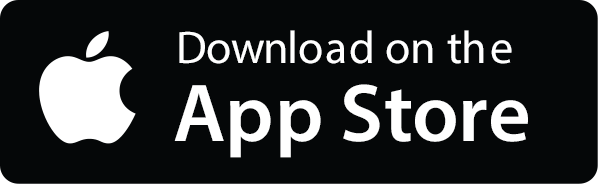
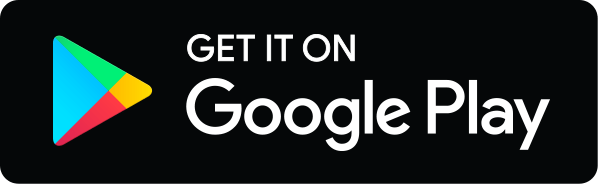
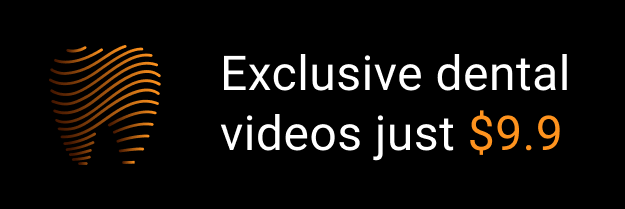