Computer-aided design/computer-aided manufacturing (CAD/CAM) technology is used to design and manufacture products using digital technologies. The term CAD/CAM implies that an engineer can use the system for designing a product and for controlling manufacturing processes. CAM procedures use manufacturing methods, the goal of which is to convert existing CAD data directly and fast without manual detours or forms in the workflow. For example, once a design has been produced with the CAD component, the design itself can control the machines that construct the object. This technology is widely used today in many different industries.
CAD/CAM implementation is rapidly changing production cycles in industry. Mass production, based on customized design, is now possible, easy, and efficient for the decentralized production of products in different locations. The development has reached the point at which individual users can produce complex pieces and parts in a small office environment. In 2006, an open source project, “RepRap,” was developed. RepRap was a free, self-replicating, desktop three-dimensional (3D) printer that anyone could build given time and materials, capable of printing plastic objects. The first version of RepRap, released in 2008, could manufacture approximately 50% of its own parts. Further versions of RepRap are currently being developed.
Additive manufacturing
Additive manufacturing, of which rapid prototyping is a subset, augments the traditional removal and assembly methods of manufacturing. This technology overcomes traditional restrictions in manufacturing, with significant commercial and technological implications. The huge potential of this technology led to the rapid development of rapid prototyping, first by Magnus in 1965 and then by Swainson in 1971. The technology for printing physical 3D objects from digital data was first developed by Charles Hull in 1984. 3D CAD models were fabricated using the sequential, or additive, layering of solidified photopolymers to reconstruct the 3D shape. After this, in 1987, the first stereolithography device was developed by 3D Systems. Selective laser sintering (SLS) was then introduced by Electro Optical Systems (EOS, GmbH, Krailing, Germany) in 1990. In 1991, three new technologies were released: fused deposition modeling (FDM) by Stratsys, and solid ground curing and cubital and laminated object manufacturing by Helisys. These technology breakthroughs set the stage for the commercial integration of additive manufacturing within manufacturing industries .
Several different additive fabrication processes are commercially available or are being developed. However, each process uses the same basic steps:
- 1.
Create CAD model
For all additive processes, CAD software is used to create a 3D model of the object.
- 2.
Convert CAD model into a .stl file format.
The STL format has become the standard file format for additive processes. The STL format represents the surfaces of the 3D model as a set of triangles, storing the coordinates for the vertices and normal directions for each triangle.
- 3.
Slice STL model into layers
The software slices the STL model into very thin layers along the xy plane. Each layer will be built on the previous layer, moving upward in the z direction.
- 4.
Build the object, one layer at a time
The machine builds the object from the STL model by sequentially forming layers of material on top of previously formed layers. The technique used to build each layer differs greatly among the additive process, as does the material being used ( Fig. 1 ).
- 5.
Postprocessing of the object
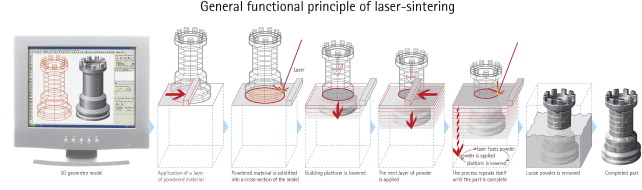
After being built, the object and any supports are removed from the machine. If the object was fabricated from a photosensitive material, it must be cured to attain full strength. Minor cleaning and surface finishing, such as sanding, coating, or painting, can be performed to improve the object’s appearance and durability ( Fig. 2 ).
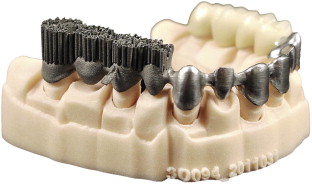
The choice of a particular technology is usually dependent on the required materials for printing, accuracy, material finish, material strength, cost, and the speed of construction. In dentistry, the possibility of product sterilization in a dental office is an important consideration for materials and products to be used.
Additional successfully commercialized technologies include fused deposition modeling, which works by additive layering of a thermoplastic material; selective layer sintering, in which a carbon dioxide layer is used to bind powders to produce solid structures; 3D printing, using inkjet printing technology to deposit a liquid binder accurately; and polyjet or polyjet matrix printing, which allows multiple materials to be deposited simultaneously before solidifying with ultraviolet lasers.
The different production technologies used are as follows:
Liquid-Based Processes
These additive technologies, such as stereolithography, use photocurable polymer resins and cure only selected portions of the resin to form each layer of the object. Other processes are based on jetted photopolymer and ink jet printing.
Solid-Based Processes
Solid-based processes use a variety of solid nonpowder materials. Most solid-based processes use sheet-stacking methods, in which very thin sheets of material are layered on top of one another. The shape of the layer is then cut out. The most common sheet-stacking process is laminated object manufacturing, which uses thin sheets of paper. FDM uses solid strands of polymer, which are extruded and deposited into layers.
Powder-Based Processes
In powder-based processes, such as SLS, direct metal laser sintering (DMLS), and 3D printing, only a selected portion of powdered material is melted or sintered to form each object layer. The use of powder enables objects to be fabricated using different materials like polymers, metals, or ceramics. The mechanical properties of these objects are normally very good and stable.
Applications
3D printing techniques have been used for a range of applications from product design, manufacturing, building biologic tissues, and large-scale housing production. Inkjet technology is ready for deployment in manufacturing processes and has already been used to deposit adhesives that bond chips to substrates in the production of memory chips. Scientists and engineers have experimented with using inkjet technology and 3D printing to print simple electronics and prototypes of other products.
Research is underway to increase the range of materials that can be used in 3D printing technologies. Recent progress has been made in printing glass and complex plastics ; however, the ability to print electronic components is still limited. This area is of significant interest. Advances in nanoscale electronic components, such as nanocapacitors and logic gates, would circumvent the issues with depositing larger components. Research is also underway for the 3D printing and assembly of nanostructures, both for functional components and to act as scaffolds .
3D printing techniques have also been scaled up to allow construction of large structures. The first 3D-printed building was constructed by D-shape, a company that uses granular sand to generate artificial sandstone structures with reduced construction times and costs.
The cost of commercial 3D printers has decreased from $500,000 in 1999 to approximately $10,000. Personal fabrication may have significant implications for product customization and new models of distributing and manufacturing. Inkjet technology would significantly reduce the need to transport finished goods around the world, causing substantial effects on the transportation industry and a reduction in fuel use. Home-fabricated goods would have a small carbon footprint, provided the raw materials used to make them were produced locally. The technology could be used to make a vast range of products, from domestic goods to simple and cheap solar energy–powered devices.
Applications
3D printing techniques have been used for a range of applications from product design, manufacturing, building biologic tissues, and large-scale housing production. Inkjet technology is ready for deployment in manufacturing processes and has already been used to deposit adhesives that bond chips to substrates in the production of memory chips. Scientists and engineers have experimented with using inkjet technology and 3D printing to print simple electronics and prototypes of other products.
Research is underway to increase the range of materials that can be used in 3D printing technologies. Recent progress has been made in printing glass and complex plastics ; however, the ability to print electronic components is still limited. This area is of significant interest. Advances in nanoscale electronic components, such as nanocapacitors and logic gates, would circumvent the issues with depositing larger components. Research is also underway for the 3D printing and assembly of nanostructures, both for functional components and to act as scaffolds .
3D printing techniques have also been scaled up to allow construction of large structures. The first 3D-printed building was constructed by D-shape, a company that uses granular sand to generate artificial sandstone structures with reduced construction times and costs.
The cost of commercial 3D printers has decreased from $500,000 in 1999 to approximately $10,000. Personal fabrication may have significant implications for product customization and new models of distributing and manufacturing. Inkjet technology would significantly reduce the need to transport finished goods around the world, causing substantial effects on the transportation industry and a reduction in fuel use. Home-fabricated goods would have a small carbon footprint, provided the raw materials used to make them were produced locally. The technology could be used to make a vast range of products, from domestic goods to simple and cheap solar energy–powered devices.
Limitations of 3D printing technologies
Although 3D printing technologies offer critical advantages over traditional manufacturing processes, the technologies have inherent limitations. In their current form, 3D printing processes are limited for mass production purposes. An injection molding machine, however, is capable of making several similar parts in less than a minute. Although 3D printing processes will continue to increase in speed, they are unlikely to be able to create objects as fast as molding technologies. The bottleneck lies in the fundamental physics of the processes; scanning a material with a laser (and cure the material, and recoat each layer) at a speed comparable to that of injection molding is impossible.
Nevertheless, this limitation is only valid for the production of several thousand of a common object. Because tooling must be created for each unique object one wishes to injection-mold, 3D printing is the preferred process when custom parts or low-volume production runs are needed. Moreover, if production is decentralized, the production may be performed near the source of demand around the world, rather than at one factory producing thousands of the same item. The same printers can also be instantly reprogrammed to produce different products as demanded.
Most 3D printing processes use polymers that are weaker than their traditionally manufactured counterparts. The strength of the objects produced is also not uniform in many 3D printing machines. Parts are often weaker in the direction of the build because of the layer-by-layer fabrication process. Obviously, different machines can often have varying properties.
3D printing technologies applicable to the medical industry
The following are the predominant technologies currently used in 3D printers:
-
Stereolithography
-
FDM
-
Multi jet modeling
-
SLS
-
3D printing.
Stereolithography
Stereolithography (a compound from the words stereo , from the Greek stereos, meaning “hard, physically” and also spatially, and lithographie , from the Greek lithos , meaning “stone,” and graph meaning “letters”) is a technical principle of rapid prototyping.
The process begins with a 3D model of the object, usually created with CAD software or a scan of an existing object ( Fig. 3 ). Specialized software slices the model into cross-sectional layers, creating a computer file that is sent to the stereolithography machine. The manufacture begins in a bath filled with the basic monomers of the photosensitive plastic. This light-hardening plastic, such as epoxy resin, is hardened by a laser in thin layers . The standard layer thickness ranges from 0.05 to 0.25 mm. A building platform is immersed in the tank filled with liquid photosensitive resin. A laser beam is projected onto selected regions of the resin surface. When the laser hits the resin, the monomer solidifies from a photochemically induced reaction. After the laser beam has scanned all regions of the layer to be solidified, the object is coated with a fresh layer of liquid resin. This function is typically achieved by lowering the object on the building platform and recoating the surface using a wiper blade. Because a solid model in a liquid is being developed, supporting structures are necessary. In large construction projects, overhanging objects must be removed from these supporting structures.
One key advantage of stereolithography is that it is currently the manufacturing method with the highest geometric resolution and very high surface quality. Recently developed resins allow for the fabrication of objects with mechanical properties comparable to many engineered polymers. After production, the stereolithography object can be modified with a large number of molding techniques, such as investment casting or silicone molding.
Stereolithography has several disadvantages. The necessary support structures must be removed manually or machined, usually leaving a surface finish inferior to unsupported surfaces. Because the monomer shrinks during polymerization, the buildup of internal stresses can lead to warpage later. Because of the development of advanced resins and building strategies, this problem has been minimized recently. Stereolithography can only process photopolymers or powder filled photopolymers, clearly limiting the number of available materials and certain material groups entirely. The materials can contract during production, resulting in a finished product that may show variations in volume and dimension.
Application
The production of prototypes (concept, geometry, opinion, working models) used in mechanical engineering, particularly in automotive manufacturing and medicine, uses similar product development procedures to stereolithography. An increasing trend is the direct production of final products using stereolithography (“rapid manufacturing”). An example of its use in the medical field is the production of individual housings for hearing aids and of surgical guides in dentistry .
FDM
FDM is a manufacturing method in the range of rapid prototyping. In this process, a plastic or wax material is extruded through a nozzle that traces the planned object’s cross-sectional geometry layer by layer. The nozzle contains heaters that keep the plastic at a temperature just above its melting point so that it flows easily through the nozzle and forms the layer. During the cooling that follows, the material solidifies immediately after flowing from the nozzle and bonds to the layer below. Once a layer is built, the platform lowers and the extrusion nozzle deposits another layer . The layer thicknesses depend on application, usually 0.017 to 1.25 mm, with a wall thickness at least approximately 0.2 mm. A range of materials are available, including acrylonitrile butadiene styrene (ABS; a common photoplastic material), polyamide, polycarbonate, polyethylene, polypropylene, and investment casting wax ( Figs. 4–6 ).
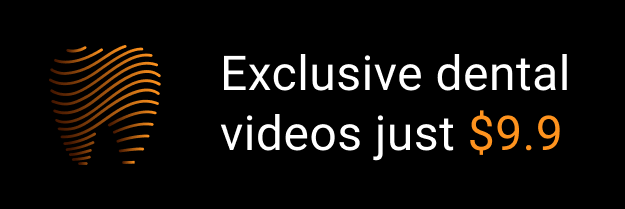