Chapter 3
Computer‐Aided Manufacturing (CAM)
Mayra T. Vasques, Gabriel S. Urbano, Ivan O. Gialain, Jacqueline F. Lima, Fábio Andretti, Ricardo N. Kimura, Danilo M. Bianchi, Dionir Ventura, Fabricio L. Gebrin, Adriano R. Campos, and Arthur R.G. Cortes
Three‐dimensional (3D) printing, also known as additive manufacturing and rapid prototyping, is relatively new in dentistry. In addition to equipment and software, 3D printing demands profound knowledge, which requires a learning curve to achieve mastery. This chapter addresses the main types of 3D printing technology used in dentistry and the most important characteristics and materials used.
In general, additive manufacturing works by layering agglutinating particles through different processes until the desired 3D object is obtained. Unlike subtraction processes used in milling machines, where the material removed may not be reused, this technology saves material and produces little waste because it adds only the necessary material. Usually, the object is printed from a STL or OBJ file format, but there are several variations according to software and equipment. These files are originated from images acquired from scanning and cone beam computed tomography (CBCT) and are processed in computer‐aided design (CAD) software. After obtaining the final STL file, the software performs the slicing to be sent for printing on the 3D equipment.
Three‐dimensional printing has become more affordable in recent years and has quickly evolved for use in dentistry. This generated great competition among equipment manufacturers and a race for more affordable technology developments to serve different fields. This ultimately leads to equipment with more reasonable prices, increasing the demand for different materials and encouraging input industries in dentistry to develop high‐performance intra‐ and extraoral materials for virtually all specialties. In addition, 3D printers have become essential equipment in dental laboratories and are quickly arriving at offices and clinics.
Three‐dimensional printers indicated for dental use include FDM (fused deposition modeling), SLS (selective laser sintering), and SLA (stereolithography) technologies – the latter is the most used today.
The FDM 3D printer, also known as a filament printer, is the most straightforward technology, with the most affordable equipment and supplies, and available in various brands and models. It uses thermoplastic materials that generate objects by material deposition through an automated extrusion process at high temperatures, with layer thicknesses ranging from 100 to 300 μm. This type of printer is also known as FFF (fused filament fabrication). It uses a wide range of materials such as ABS (acrylonitrile butadiene styrene), ASA (acrylonitrile styrene acrylate), PLA (polylactic acid), PETG (polyethylene terephthalate glycol), and nylon, in the form of filaments sold in rolls and ready to be introduced into the machines. Despite its cost‐effectiveness, its use in dentistry is limited to producing models that do not require high definition and complexity, such as study models and biomodels, hollow models for study in orthodontics, and tools such as photoretractors, holders, and stock trays. The most used materials for printing these objects are ABS and PLA, as they have reduced cost, ease of handling, and a range of colors and settings for the vast majority of FDM printers on the market.
Selective laser sintering (SLS) technology uses high‐power laser to aggregate particles by sintering. Sintering can be defined as a thermophysical process activated by a laser source, which makes a set of particles of a particular material to be joined, arriving at the desired structure with high mechanical resistance. The materials used for this type of 3D printing are found in powder in various polymeric compounds, metals, and ceramics. These materials include nylon, PEEK (polyether ether ketone), metals such as titanium, and ceramics. In dentistry, this method can manufacture structures for fixed dentures, implant dentures, removable partial dentures, dental implants, and dental crowns. Advantages of this type of process, such as no need for supports, low waste rate, excellent mechanical properties of materials, and high precision, make this technology highly promising. However, the high value of equipment and supplies, the need for specialized labor for maintenance, the large size of the equipment, and the high complexity of the machines’ operation mean that it is not currently used in dentistry and dental laboratories.
Among all the additive manufacturing technologies, the most used today in dentistry is SLA, which we will emphasize here. By definition, stereolithography is a 3D printing technology that uses photochemistry, that is, chemical components cured by a light source, layer by layer, whereby light causes monomers and oligomers to cross‐link to form polymers constituting the 3D body.
Different SLA technologies stand out today in dentistry, the main ones being laser (light amplification by stimulated emission of radiation), DLP (digital light processing), and LCD (liquid crystal display) (Table 3.1). All typically use light sources with wavelengths that can range from 380 to 405 nm.
Table 3.1 Main types of technology and printers used in dentistry, their synonyms, advantages, and disadvantages.
Technology | Types of 3D printer | Synonyms | Benefits | Disadvantages |
---|---|---|---|---|
SLA | Laser | SLA | High definition, repeatability, print table size, low distortion | Specialized maintenance, equipment price, high spare parts price, slow print speed for large quantities of objects, unique formulation resins, generally closed for configurations |
DLP | Projector printers | High definition, repeatability, excellent speed, low distortion, equipment durability, printing table size, greater variety of resins | Specialized maintenance, equipment price, high price of spare parts, some equipment closed for configurations | |
LCD | MSLA | Low price, cheap spare parts, open configuration for all types of resins, good speed, printing table size, larger variety of resins, small and light | They break more often and have more distortion in the corners of the screen | |
FDM | FDM | FFF | Low price of inputs and equipment, recyclable materials | High print time, poor definition for most dental indications, lack of registered materials for intraoral use, constant maintenance, energy consumption |
Laser printers are commonly referred to as just SLA as they were the first affordable desktops on the market. However, the correct nomenclature would be SLA‐laser. They are characterized by using an ultraviolet (UV) laser beam and a system of motors and mirrors that move in combination to obtain the design of each layer. Due to this feature, they are usually slower when creating several objects, as they need to move across the entire layer, unlike DLP and LCD printers that project an entire layer at a time. The print results are excellent, and definition depends on the laser focus size and speed. They have high prices, maintenance costs, and replacement parts with high values.
SLA‐DLP 3D printers use DLP technology initially developed in 1987 by Larry Hornbeck of Texas Instruments, based on optical micro‐electromechanical technology composed of digital micromirror devices. Initially, this technology was used for other purposes such as front projectors for cinemas, home cinemas, projectors for classrooms, and televisions. In the 1990s, DLP technology started to be implemented in additive manufacturing. The first DLP printers used mercury lamp projectors and, after industrial improvement, miniprojectors that use LED UV (light‐emitting diode ultraviolet). The equipment ranges from medium to high prices, delivers excellent definition and finish, and the printers currently offer improved repeatability, safety, and low maintenance, but with high‐value replacement parts. Their reservoirs also use PDMS silicone or fluorinated ethylene propylene (FEP) film on the bottom.
SLA‐LCD 3D printers utilize a LCD and LED UV light source to generate the print layers. LCDs were not initially designed for this use and have been used in TV monitors, cellphones, displays, clocks, radios, and other equipment since their creation in the mid‐1960s. The idea of a LCD based on TFT (thin‐film transistor) was conceived by Bernard Lechner of RCA Laboratories in 1968. The light source is located inside the printer, right below the LCD. When projecting the image of each layer, the LCD releases the passage of light and polymerizes the resins. The number of LEDs and lenses varies according to brand and model. The models differ mainly according to the type of LCD used, ranging from monochrome, color, size in inches, and different resolutions (full HD, 2, 4, and 8 K). These devices have attractive prices and low maintenance costs, so they are usually the first choice of those wanting to enter the world of 3D SLA printing. However, they are more fragile and because of the LCD’s location just below the reservoir, they tend to break quite often.
SLA resins are liquid composites mainly composed of oligomers and monomers, but they need other additives to work in each type of equipment, such as UV blockers, inhibitors, dispersants, pigments, photo activators, and other types of fillers. The formulation of each resin depends exclusively on its indication and the equipment that will be used. The choice of formulation components is directly linked to each need and physical characteristics, such as the equipment’s wavelength, flexural strength, tensile strength, impact resistance, cycling, viscosity, biocompatibility, color, odor, etc. As an example, we can mention the resin used for making temporary and definitive crowns, which uses loads similar to the composite resin in the office, such as glass or ceramics. Resins for SLA printers in dentistry are divided into two large groups, biocompatible and nonbiocompatible, and we can see their types and indications in Table 3.2.
Most of the components of a 3D SLA resin are thermoset elements derived from petroleum which, unlike thermoplastics, are not recyclable in their solid state (after printing). This factor represents a significant risk to the environment since 3D printing is generating more and more residues that will remain in the ecosystem for long periods. In addition, in liquid form, contamination is highly harmful to aquatic environments after washing or disposing of the bottles, especially for mixed polarity resins, so‐called “water‐soluble,” as they disperse more easily in water, offering more significant toxicity. Regarding sustainability, companies that produce inputs must think about how to reuse solid materials and create biodegradable materials, and the end‐users must become aware of responsible disposal. Some companies are already developing sustainability projects in this sector, such as Makertech Labs, the first Brazilian company in 3D resins, which receives material from its largest customers, transforms it into powder in grinders, and inserts it into civil construction materials, reducing the environmental impact.
Table 3.2 Types of materials used in resin and filament 3D printers, and their indications in dentistry.
Materials | Indications (dentistry) |
---|---|
Biocompatible resins | Surgical guides, occlusal splint, retainers, temporary and extended‐use teeth, orthodontic bonding guides, total and removable denture bases, individual trays, mouthguards |
Nonbiocompatible resins | Study models, die‐cast models, working models, biomodels, artificial gingiva for models, elements for metal casting, elements for lithium disilicate injection |
Filaments | Models and biomodels for study, hollow models for orthodontics, retractors for photography, positioners, and stock trays |
3.1 Fused Deposition Modeling (FDM)
3.1.1 Basic Knowledge
Fused deposition modeling technology, also known as FFF, is an additive manufacture procedure where a thermoplastic material (presented as a filament) is heated into a semisolid state to form, layer by layer, a 3D geometry. The FDM printing process involves generic components, such as the printing bed, axes motors (X, Y, and Z), extruder motor, and heated extruder.
The basic principle of FDM printing is that thermoplastic filament heats inside the extruder, and the extruder motor pushes the filament through the extruder’s nozzle. The extruder, nozzle, and cooling fans move through the X, Y, and Z axes. Four motors control the material deposition (X, Y, Z, and extruder) over a printing bed that heats. The material is superimposed in layers, fusing the new layer over the last. After cooling time, all layers are fused, forming a 3D object.
While preparing for printing, one should carefully place all parts over the printing bed or previously printed layer. If there are any overhanging parts, preprocessing software may create additional supports that postprocessing will remove.
Most FDM printers and filaments are cost‐effective compared with other 3D printing technologies, making this method very suitable for dental offices, especially for beginners in the digital workflow. Other advantages of this technology are the relatively simple operation and low postprocessing time.
On the other hand, the main disadvantage of FDM is the lower printing resolution (compared with SLA and DLP, for example), which is around 100 μm on the Z‐axis. Even though some high‐resolution FDM printers can achieve a 20–50 μm Z‐layer resolution, these printers are more expensive. Other disadvantages are dimensional accuracy and reproducibility. Since FDM technology uses thermoplastic material (Table 3.3), autoclave sterilization may not be achievable for most materials available, but low‐temperature sterilization methods can be used.
The majority of FDM printings for dental purposes use ABS and PLA materials, but other materials, such as TPU (thermoplastic polyurethane), PC (polycarbonate), PETG, and other polymers can be used. The main advantages of ABS are cost (cheaper than PLA), machinability (the final part can combine additive and subtractive manufacturing procedures), and that it can be recycled. On the other hand, PLA is biodegradable (usually made from corn starch), needs lower printing temperature, and has lower shrinkage.
Some biopolymers and other filaments are in development, and further studies may demonstrate different applications in dentistry, including flexible materials. Other filament materials may be available for FDM usage, but they are mainly used for artistic or engineering purposes.
In implant dentistry, FDM printers are mostly used for fabricating study and work biomodels (Figure 3.1), individual impression trays, prototypes for bone regeneration scaffold guides, and surgical guides.
Table 3.3 Materials used for filament 3D printing.
Material | Indication | Advantages | Drawbacks |
---|---|---|---|
ABS | Fabricate biomodels, impression trays, surgical guides | Stiff and durable material, low cost, recyclable | Produces toxic gases while printing, high printing temperature, warps easily |
PLA | Biodegradable, low warping, low temperature to print, easy to print | Water absorption, low impact strength, may clog the nozzle | |
PETG | Smooth finishing, food‐safe, moisture resistance | Low wear resistance, high printing temperature, not so many options as PLA and ABS | |
PC | Thermal resistance, mechanically strong | More challenging to print, high printing temperature, higher pricing | |
TPU | Simulate soft tissue in biomodels | Flexibility, wear resistance, chemical resistance (oils) | More challenging to print, high printing temperature, higher pricing |
3.1.2 Step‐by‐Step Procedure
The workflow with an FDM printer has four main processes: calibration, preprocessing, printing, and postprocessing.
3.1.2.1 Calibration
- Each printer has its own calibration process, but in this step‐by‐step guide, a low‐cost FDM printer (Anycubic) will be used.
- After assembling the printer (if needed), check that the frame is squared and all belts are correctly tensioned. If something is wrong, the printing will not have dimensional accuracy.
- All parts of the printer must be cleaned and lubricated (where needed) to prevent excess friction between components and to allow the correct function of all cooling fans.
- Tray leveling: there are usually four knobs under the printing tray that regulate the bed height to be parallel to the X and Y movement. Some printers have an auto tray leveling feature that helps to ensure correct tray leveling. The most common technique used to level the bed is to put the nozzle in various points over the bed (at the same Z distance) and regulate the distance until a regular piece of paper fits between the nozzle and the bed. This technique is straightforward (but not very accurate) for adjusting bed height, but more advanced techniques use equipment and tools, such as a feeler gauge or dial indicator. If the nozzle is too far away from the bed, the print may detach from the print bed and if the nozzle is too close, the filament may create a print line with excess material on the sides (elephant’s foot effect).
Figure 3.1 (a) Calibration of the filament 3D printer. (b–d) Dental model manufacture.
- The nozzle and filament tube must be clear of any clogging. If there is any filament clogging, it can affect correct extrusion and cause print failure. There is a specific needle to clear blockages, and this procedure must be done with the extruder at printing temperature.
- All axes’ motors must be calibrated. A printing blank is printed to check X, Y, and Z calibration (usually a calibration cube with known dimensions), and the printed cube must be measured. If there are any discrepancies, the steps/mm option must be adjusted. For print smaller than the 3D file, the steps/mm should be increased using a simple proportion calculation, and this adjustment must be made for all three axes.
- The nozzle must be at printing temperature to calibrate the extruding motor, and a mark with a permanent marker should be made at a 110 mm distance from the extrusion motor’s entry. After making the mark, order the printer to extrude a smaller distance than the mark (100 mm). The remaining distance between the mark and extruder’s entry should be 10 mm. Following the same instruction as for X, Y, and Z, the extruder’s steps/mm should be calibrated.
- Each material must be printed at a specific temperature, but the printing temperature may slightly change even among batches of the same material and manufacture. For improved results, test printings are available to test several printing temperatures in one print, and the user can choose the best for each situation. Usually, manufacturers advise the temperature range to print with each material.
- There are more advanced calibration processes, but these are the initial steps to print with an FDM printer.
After the FDM printer is calibrated, the next step is to adjust the geometry in CAD software. In this example, open‐source software will be used (Autodesk Meshmixer®, version 3.5.474) to prepare a maxillary arch study biomodel. These are the steps for the preprocessing phase.
- Import the STL file created from intraoral scanning.
- Since the file may have undesired geometries, it must be cleaned. This process is achieved by selecting the sections that are not going to be printed and deleting them.
- After cleaning the unwanted sections, the model must be transformed into a closed volume since the 3D printer software recognizes closed meshes. To close the geometry with a flat surface, the boundaries can be smoothed and extruded (by selecting the border triangles and using “smooth boundary” and “extrude” tools), and lastly creating a closed mesh with the “make solid” tool.
- Export the printer‐ready STL file and open the slicing software. In this example, the Ultimaker Cura slicer (version 4.6.1) was used, and the model was rotated to create a need for support material.
- Choose the print parameters adjusted for the printed and filament material and save the G‐code file. For this example, a PLA filament was used with nozzle temperature of 200 °C, layer height of 200 μm, and 20% infill (a solid infill would take more time and material but not increase print quality). The total time for printing is approximately 1 hour and 55 minutes, using 12 g (or 4.12 m) of filament.
3.1.2.2 Printing and Postprocessing
When the G‐code file is ready, it must be sent to the printer, either via a micro‐SD card or using a wi‐fi connection. The printer should automatically adjust the bed and nozzle temperatures and print the object without any issues. After printing time is over, the supports must be removed using clippers, and the final model is ready.
3.2 Liquid Crystal Display (LCD)
3.2.1 Basic Knowledge
Liquid crystal display is a vat photopolymerization technology known as masked stereolithography apparatus (MSLA). It consists of an array of LEDs and an LCD screen that acts as a dynamic mask, allowing the light to reach the resin through its off pixels, forming the design of the printing layer. LCD is part of the second generation of SLA which uses the projection of an entire layer of light to cure the resin instead of a single laser beam, making the technology significantly faster than the original SLA [1].
In this equipment, the resin vat is placed over the LCD surface with the FEP film in direct contact. As the LED is the weakest light source in vat 3D printing, it must be as close as possible to the polymerizing resin. The light power is an essential characteristic because it can damage the LCD pixels. Another factor is the risk of resin leakage from the vat which may permanently damage the LCD surface [2].
The lifetime of the LCD is extended by reducing the polymerization of each layer to the minimum required to make the material rigid and maintain the object dimensions without distortions. Considering the photoinitiators, the use of specifically formulated resins also favors the performance of LCD printers. LCD printers can use most of the materials used by DLP printers, but the light exposure time has to be higher to obtain the same results. Due to this characteristic, it is mandatory to finish the polymerization in a UV light chamber to achieve the best materials properties [3].
The LCD resolution is an essential factor for accuracy of the printed object, considering it is formed by square pixels, making actual curves impossible to reproduce. A high‐resolution screen minimizes the geometric effect; another way to improve the object surface is the anti‐aliasing that changes to average edge boundary pixels. The cooling system is another primary focus for equipment longevity, and appropriate LED cooling reduces the damage caused to the LCD screen, allowing the best air circulation [4, 5].
The most significant advantage of LCD is its lower price, as the light source is cheaper compared to other types. LCD technology is often the first choice for beginners. The equipment parts are shown schematically in Figure 3.2.
3.2.2 Step‐by‐Step Procedure
As with any dental procedure, 3D printing requires a step‐by‐step process to carry out the project. This section describes how 3D printing is done on the most common LCD printers on the market.

Figure 3.2 Schematic presentation of the parts of an LCD printer.
Liquid crystal display printing popularized 3D printing with resins. With the emergence of numerous manufacturers, 3D printing became efficient and cheap, enabling many dentists and technicians to venture into the world of digital dentistry. The operation of these printers is as simple as their price is affordable. Briefly, LCD is a screen with a UV light filter, which turns on or off as requested by the software.
At the bottom of the printer is a UV light source emitted to the LCD above it. At this time, the LCD is off, not letting the light through. As soon as it reads the file that it was asked to print, it turns on the image of the layer that was requested in the file at that instant. In this way, UV light passes through the LCD and polymerizes the region of that slice predefined in the slicing software.
Three‐dimensional printing is composed of five steps: STL file acquisition, settings in software, slicing, object printing, washing/postcure.
3.2.2.1 Acquisition
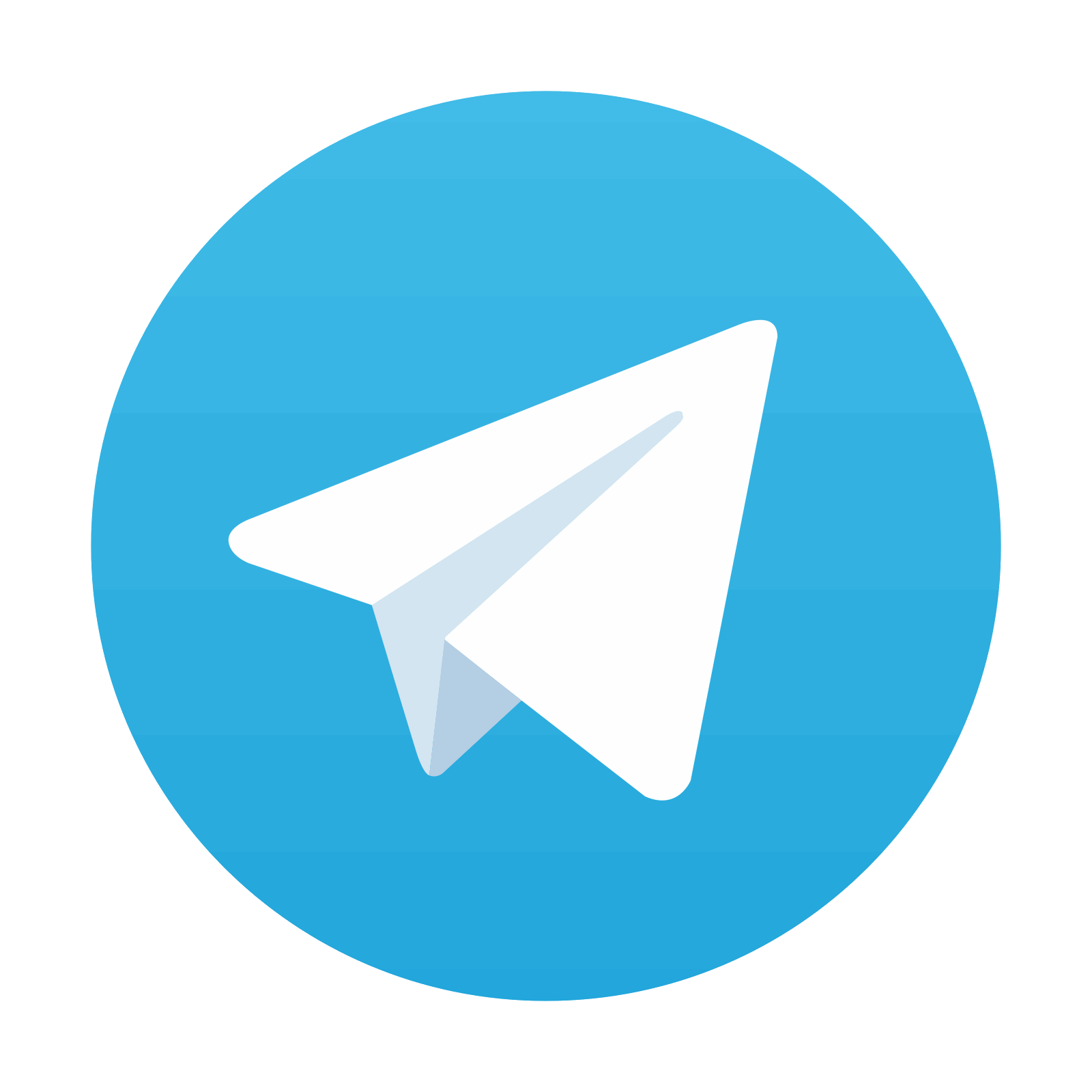
Stay updated, free dental videos. Join our Telegram channel

VIDEdental - Online dental courses
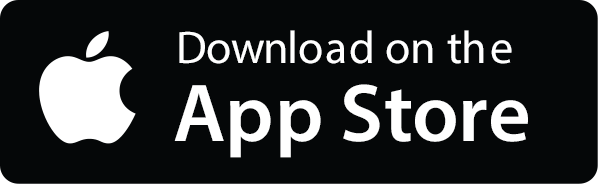
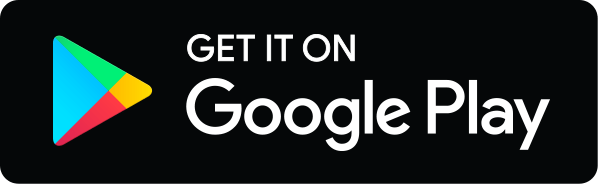