Highlights
- •
Improved composite mechanical properties with higher ELP and collagen content.
- •
Promoted proliferation of hASCs on ELP-collagen scaffolds.
- •
Increased osteogenic differentiation with higher ELP and collagen content.
- •
A promising composite scaffold for GBR application.
Abstract
Objective
Collagen-based scaffolds for guided bone regeneration (GBR) are continuously improved to overcome the mechanical weaknesses of collagen. We have previously demonstrated superior mechanical characteristics of the elastin-like polypeptide (ELP) reinforced collagen composites. The objectives of this research were to evaluate the efficacy of ELP-collagen composites to culture human adipose-derived stem cells (hASCs) and allow them to undergo osteogenic differentiation. We hypothesized that hASCs would show a superior osteogenic differentiation in stiffer ELP-collagen composites compared to the neat collagen hydrogels.
Methods
Composite specimens were made by varying ELP (0–18 mg/mL) and collagen (2–6 mg/mL) in a 3:1 ratio. Tensile strength, elastic modulus, and toughness were determined by uniaxial tensile testing. hASCs cultured within the composites were characterized by biochemical assays to measure cell viability, protein content, and osteogenic differentiation (alkaline phosphatase activity, osteocalcin, and Alizarin red staining). Scanning electron microscopy and energy dispersive spectroscopy were used for morphological characterization of composites.
Results
All composites were suitable for hASCs culture with viable cells over the 22-day culture period. The ELP-collagen composite with 18 mg/mL of ELP and 6 mg/mL of collagen had greater tensile strength and elastic modulus combined with higher osteogenic activity in terms of differentiation and subsequent mineralization over a period of 3 weeks compared to other compositions. The extra-cellular matrix deposits composed of calcium and phosphorous were specifically seen in the 18:6 mg/mL ELP-collagen composite.
Significance
The success of the 18:6 mg/mL ELP-collagen composite to achieve long-term, 3-dimensional culture and osteogenic differentiation indicates its potential as a GBR scaffold.
1
Introduction
Periodontitis is the inflammation of the periodontium surrounding the tooth with progressive loss of alveolar bone resulting in complete loosening of the tooth. Guided bone regeneration (GBR) and guided tissue regeneration (GTR) are widely used techniques that regenerate the various periodontal tissues including the root cementum, periodontal ligaments, and alveolar bone, thereby decreasing the mobility of the affected tooth . GTR uses a resorbable or non-resorbable membrane as a barrier between the epithelial and connective tissues. This membrane prevents the unwanted re-growth of the epithelial and connective tissues . GBR uses a resorbable or non-resorbable scaffold to support the regeneration of the alveolar bone at a bony defect introduced by tooth extraction or disease . The materials used in GBR/GTR must be biocompatible, non-immunogenic, and non-inflammatory. In addition, the GBR/GTR materials must possess adequate strength to allow proper handling for their placement at the defect site and modulus to avoid collapse under the intraoral forces. Finally, the GBR/GTR materials must degrade in vivo at a rate that matches the rate of the new tissue formation .
The current trend in periodontal tissue engineering research is focused toward bone regeneration at targeted sites by combining stem cells, scaffolds, and growth factors. Human mesenchymal stem cells (MSCs) are an attractive source of stems cells for bone regeneration as they have the capacity to regenerate and differentiate into osteogenic lineage . Human adipose-derived stem cells (hASCs) have emerged as an alternative to human bone marrow MSCs (hBMSCs) due to their easy procurement from liposuction patients, rapid in vitro expansion, and high harvest yield . hASCs have a demonstrated ability to differentiate into osteoblastic lineage in vitro as well as in the clinical treatment of bone defects . Therefore, for this research, we have used hASCs to evaluate their osteogenic function in different scaffolds. For GBR applications, scaffolds act as the housing in which the osteoregenerative cells like hASCs can proliferate, differentiate, and mineralize into bone. Successful biocompatible scaffolds should possess adequate mechanical and resorption properties along with suitable osteoconductive and osteoinductive properties. Unfortunately, the currently available synthetic scaffolds prove inadequate for clinical applications. For instance, the non-resorbable scaffolds require a second surgery for their removal, causing economic and physical discomfort for the patient . Synthetic resorbable polymers suffer from early loss of structural and mechanical properties with poor cell response . Natural resorbable polymers such as collagen are osteoinductive and an effective alternative to synthetic polymers due to their biocompatibility and excellent cell affinity . However, they often suffer from rapid hydrolytic and enzymatic degradation and lack sufficient mechanical properties . Crosslinking of collagen using chemicals like glutaraldehyde and 1-ethyl-3-(3-dimethylaminopropyl) carbodiimide hydrochloride can overcome poor mechanical properties and antigenicity but the possibility of cytotoxicity increases . Blending of collagen with other polymers and ceramics has shown to enhance mechanical properties and modulate specific cellular responses . These disadvantages of both non-resorbable and resorbable materials make it clear that an “ideal” material has not been found and have led to approaches that use non-crosslinked collagen composites .
In the design of bio-inspired bone regenerative composites with non-crosslinked collagen, elastin-like polypeptides (ELP) are under investigation as they have amino acid sequences similar to native elastin . ELP, which is a soluble and recombinant form of elastin, offers an attractive solution to the problems faced by non-resorbable and resorbable synthetic materials used previously to prepare collagen-based composites. ELPs display inverse phase transition behavior which allows easy purification . More importantly, ELPs possess excellent mechanical properties . In the past, we prepared non-crosslinked ELP-collagen composites by utilizing the gelation behavior of collagen at 37 °C and showed their superior mechanical behavior and equivalent biocompatibility compared to neat collagen hydrogels . We demonstrated that ELP-collagen composite scaffolds help in the attachment, proliferation, and subsequent differentiation of 3T3-E1 mouse pre-osteoblastic cells .
Mechanical properties such as stiffness or modulus play a crucial role in the success of a scaffold and also influence the commitment of cells to a specific lineage. Studies have shown that osteogenic differentiation is stiffness/modulus dependent in hBMSCs . Huebsch et al. showed that both human and murine MSCs cultured in the presence of mixed osteogenic and adipogenic supplements can be made to undergo either adipogenic or osteogenic differentiation based on the matrix stiffness . Though the effect of matrix stiffness has been well studied with hBMSCs, the lacuna with hASCs led to the development of this study. We aimed to study the effect of scaffold composition and stiffness on the ability of hASCs to commit to osteogenic lineage. We hypothesized that hASCs would differentiate along the osteoblastic lineage in a more rigid ELP-collagen composite compared to the neat collagen hydrogels. Hence, we investigated the efficacy of composites made with varying concentrations of ELP and collagen for hASCs culture to produce viable and differentiated osteoblast-like cells and investigated the influence of mechanical properties like elastic modulus of the composites on the osteogenic differentiation.
2
Materials and methods
2.1
Expression and purification of ELP
Preparation of ELP was performed as described elsewhere . Briefly, Escherichia coli BLR(DE3) bacteria (Novagen, Madison, WI, USA) with synthetic gene for ELP having primary sequence of [Valine-Proline-Glycine-Valine-Glycine] 120 were cultured for 24 h at 37 °C and lysed by sonication. The inverse phase transition behavior of ELP was used for purification by three repeated cycles of solubilization in deionized water at 4 °C, followed by precipitation and centrifugation at 40 °C. The ELP solution was dialyzed and then lyophilized (Labconco Corp., Kansas City, MO, USA).
2.2
Preparation of composites for mechanical testing
Collagen type I (9 mg/mL, rat tail, Invitrogen, Carlsbad, CA, USA) and ELP were mixed at varying concentrations to form four different compositions, namely, H1: 0 mg/mL ELP + 2 mg/mL collagen, H2: 6 mg/mL ELP + 2 mg/mL collagen, H3: 0 mg/mL ELP + 6 mg/mL collagen, and H4: 18 mg/mL ELP + 6 mg/mL collagen ( Table 1 ). To prepare H2 and H4 composites, ELP (6 mg/mL or 18 mg/mL) was first dissolved in sterile DI water and gently mixed with collagen (2 mg/mL or 6 mg/mL, respectively). Next, 1N NaOH and 10X PBS were added. To prepare the control H1 and H3 collagen hydrogels with no ELP, collagen (2 mg/mL or 6 mg/mL) was gently mixed with 1N NaOH and 10X PBS. 350 μL of the final solutions for H1, H2, H3, and H4 was incubated at 37 °C and >70% humidity for 18 h per dog-bone shaped well of a custom-made acrylic mold for mechanical testing.
Composite | ELP (mg/mL) | Collagen (mg/mL) | ELP-collagen ratio |
---|---|---|---|
H1 | 0 | 2 | 0 |
H2 | 6 | 2 | 3:1 |
H3 | 0 | 6 | 0 |
H4 | 18 | 6 | 3:1 |
2.3
Mechanical testing
The dog-bone shaped specimens ( n ≥ 6) were kept wet by immersion in PBS at 37 °C and >70% humidity until they were tested on day 8. The dimensions of the specimens were measured using VHX Digital Microscope (Keyence Corp., Osaka, Japan) and Vernier calipers. Average thicknesses of the H2 and H4 ELP-collagen composites were 0.84 ± 0.30 and 1.47 ± 0.45 mm, respectively and were not statistically different ( p > 0.05) from their respective H1 and H3 control collagen hydrogels (0.76 ± 0.33 and 1.54 ± 0.45, respectively). Average widths of the H1, H2, H3, and H4 composites were 3.25 ± 0.53, 2.89 ± 0.61, 3.32 ± 0.35, and 3.49 ± 0.34 mm, respectively. As expected, the widths of all composites were not statistically different ( p > 0.05) since they were prepared in the custom-made, dog-bone shaped acrylic mold having precise width. Uniaxial testing was performed with ASTM D882 on a Sintech 2/G Materials Testing System (MTS, Eden Prairie, MN, USA) at an extension rate of 0.5 in/min (12.7 mm/min). Tensile strength, elastic modulus, and toughness were calculated using MTS Testworks 4.0 software.
2.4
Cell culture
hASCs were obtained from an unidentified adult female patient who underwent liposuction in accordance with the protocol approved by the University of Mississippi Medical Center Institutional Review Board (IRB Approval # 2012-0004). hASCs were maintained and expanded at 37 °C and 5% CO 2 in 50:50 Dulbecco’s modified Eagle’s medium (DMEM, HyClone Labs, South Logan, UT, USA) and F12 (Invitrogen, Carlsbad, CA, USA) supplemented with 10% calf serum, 1% penicillin-streptomycin, and 1 mM sodium pyruvate with pH adjusted to 7.4. Cells were harvested using trypsin-EDTA at 80% confluence. These cells were seeded within the collagen and collagen-ELP composites as described below.
2.5
Preparation of composites for cell culture
Four compositions (H1, H2, H3, and H4) were prepared as described above, except 10X DMEM containing 50,000 cells was used instead of 10X PBS. 200 μL of the final solution was incubated per well of a 96-well tissue culture polystyrene plate (Costar, Corning Life Sciences, Lowell, MA, USA) at 37 °C and >70% humidity for 18 h. All composites were prepared in triplicate. After 24-h acclimation, the composites were supplemented with an osteogenic cocktail containing DMEM, 50 mM l -ascorbic acid, 10 nM dexamethasone, 10 mM β-glycerophosphate, 10% fetal bovine serum, and 1% penicillin/streptomycin for 3 weeks. Fresh media was fed every 48 h.
2.6
Biochemical analysis
All the assays were performed according to the manufacturers’ protocols. Cell viability within the various composites was assessed on day 22 using a Live/Dead assay (Invitrogen, Carlsbad, CA, USA). The cells were washed with sterile PBS and Live/Dead reagents (4 μM EthD-1 and 2 μM calcein AM) were added. The composites were incubated for 25 min at 37 °C. Observations were made by epifluorescence microscopy using an Olympus IX 81 microscope (Olympus America, Center Valley, PA, USA) equipped with a Slidebook image analysis software. The percentages of live and dead cells were calculated by measuring the fluorescence at 645 and 530 nm, respectively using a FLX-800 fluorescence plate reader (Biotek, Winooski, VT, USA).
For quantification of total protein content, alkaline phosphatase activity, and osteocalcin production, triplicates of H1, H2, H3, and H4 composites were removed from the plates to isolate the cells. The cells were first separated from the composites by digestion using 1 U/mL Liberase (Roche Diagnostics, Indianapolis, IN, USA) in CaCl 2 —Krebs Ringer buffer for 25 min at 37 °C. The cells were then pelleted from the media via centrifugation at 3500 rpm for 5 min, washed with PBS, and spun again at 3500 rpm for 5 min. The cells were then resuspended in 300 μL of PBS, lysed by sonication at 10% amplitude for 30 s, and immediately frozen.
Total protein amount present in the samples of days 1, 8, 15, and 22 were measured using a BCA total protein assay kit (Thermo Scientific, Rockford, IL, USA). 50 parts of reagent A and 1 part of reagent B supplied by the manufacturer were mixed to form the working solution. 25 μL of the lysate was added to 200 μL of the working reagent, mixed on a shaker for 30 s, and incubated at 37 °C for 20 min. The absorbance was measured at 540 nm on ELX-800 absorbance plate reader (Biotek, Winooski, VT).
A colorimetric kinetic determination of alkaline phosphatase (ALP) activity was performed using QuantiChrom ALP assay kit (BioAssay Systems, Hayward, CA, USA) on days 8, 15, and 22. In brief, 25 μL of the cell lysate was transferred into a 96-well plate along with 150 μL of working solution (10% v/v Magnesium acetate and 4% v/v p -nitrophenyl phosphate liquid substrate in assay buffer) and the absorbance was measured at 405 nm at 0 and 4 min on ELX-800 absorbance plate reader. The ALP activity was then calculated as per manufacturer’s instructions.
A sandwich ELISA assay specific for human osteocalcin (OCN) was performed using osteocalcin assay kit (Invitrogen) on days 8, 15, and 22. In short, monoclonal antibodies bound to the human OCN in standards or samples (25 μL), were bound to a monoclonal antibody labeled with horse radish peroxidase (100 μL) for 2 h at room temperature. The surfaces were washed extensively with a wash solution and reacted to a chromogenic solution in the dark at room temperature for 30 min. The reaction was completed with 100 μL of the stop solution and absorbance was measured at 450 nm using ELX-80 absorbance plate reader. The OCN activity was then calculated as specified by the manufacturer.
Next, the osteogenesis quantitation kit (EMD Millipore, Billerica, MA, USA) was used to analyze mineralization on day 22. The cell-seeded composites were washed with PBS and fixed using 10% formaldehyde for 15 min. After further washing, the composites were incubated at room temperature for 20 min in 1 mL Alizarin red stain per well. The excess dye was removed by extensive washing with DI water. Mineral deposits in composites were stained bright red and visualized using VHX Digital Microscope. Quantitative analysis was performed by further incubating the composites in 10% acetic acid for 30 min with shaking, then vigorous vortexing for 30 s, and heating at 85 °C for 10 min. The extracted mixture was centrifuged at 22,000 × g for 15 min and neutralized with 150 μL of 10% ammonium hydroxide. The absorbance of the solution was measured at 405 nm using ELX-800 absorbance plate reader.
2.7
Scanning electron microscopy (SEM) with energy dispersive spectroscopy (EDS)
The cell seeded composites were washed with sterile PBS and fixed in 4% paraformaldehyde for 1 h at room temperature. They were then dehydrated using ascending ethanol series of 50%, 70%, 80%, 90% and 100% ethanol for 10 min each. The composites were then dried in the desiccator overnight. The dried composites were mounted on aluminum SEM stubs using carbon adhesive tape and sputter-coated with gold for 2 min at 9 V. The composites were observed with SUPRA 40 scanning electron microscope (Carl Zeiss, Thornwood, NY, USA) at 5 kV using both the in-lens detector and the Everhart–Thornley secondary electron detector. Images were recorded at least from two different areas on each composite at 5000× and 10,000× magnifications. Energy dispersive spectroscopy was performed on H3 and H4 composites to determine the presence of phosphorus and calcium.
2.8
Statistical analysis
Results have been reported as mean ± 95% confidence intervals. Results were analyzed using ANOVA and Games-Howell post hoc test for unequal variances in SPSS version 23 statistical software. Values with p ≤ 0.05 were deemed significantly different.
2
Materials and methods
2.1
Expression and purification of ELP
Preparation of ELP was performed as described elsewhere . Briefly, Escherichia coli BLR(DE3) bacteria (Novagen, Madison, WI, USA) with synthetic gene for ELP having primary sequence of [Valine-Proline-Glycine-Valine-Glycine] 120 were cultured for 24 h at 37 °C and lysed by sonication. The inverse phase transition behavior of ELP was used for purification by three repeated cycles of solubilization in deionized water at 4 °C, followed by precipitation and centrifugation at 40 °C. The ELP solution was dialyzed and then lyophilized (Labconco Corp., Kansas City, MO, USA).
2.2
Preparation of composites for mechanical testing
Collagen type I (9 mg/mL, rat tail, Invitrogen, Carlsbad, CA, USA) and ELP were mixed at varying concentrations to form four different compositions, namely, H1: 0 mg/mL ELP + 2 mg/mL collagen, H2: 6 mg/mL ELP + 2 mg/mL collagen, H3: 0 mg/mL ELP + 6 mg/mL collagen, and H4: 18 mg/mL ELP + 6 mg/mL collagen ( Table 1 ). To prepare H2 and H4 composites, ELP (6 mg/mL or 18 mg/mL) was first dissolved in sterile DI water and gently mixed with collagen (2 mg/mL or 6 mg/mL, respectively). Next, 1N NaOH and 10X PBS were added. To prepare the control H1 and H3 collagen hydrogels with no ELP, collagen (2 mg/mL or 6 mg/mL) was gently mixed with 1N NaOH and 10X PBS. 350 μL of the final solutions for H1, H2, H3, and H4 was incubated at 37 °C and >70% humidity for 18 h per dog-bone shaped well of a custom-made acrylic mold for mechanical testing.
Composite | ELP (mg/mL) | Collagen (mg/mL) | ELP-collagen ratio |
---|---|---|---|
H1 | 0 | 2 | 0 |
H2 | 6 | 2 | 3:1 |
H3 | 0 | 6 | 0 |
H4 | 18 | 6 | 3:1 |
2.3
Mechanical testing
The dog-bone shaped specimens ( n ≥ 6) were kept wet by immersion in PBS at 37 °C and >70% humidity until they were tested on day 8. The dimensions of the specimens were measured using VHX Digital Microscope (Keyence Corp., Osaka, Japan) and Vernier calipers. Average thicknesses of the H2 and H4 ELP-collagen composites were 0.84 ± 0.30 and 1.47 ± 0.45 mm, respectively and were not statistically different ( p > 0.05) from their respective H1 and H3 control collagen hydrogels (0.76 ± 0.33 and 1.54 ± 0.45, respectively). Average widths of the H1, H2, H3, and H4 composites were 3.25 ± 0.53, 2.89 ± 0.61, 3.32 ± 0.35, and 3.49 ± 0.34 mm, respectively. As expected, the widths of all composites were not statistically different ( p > 0.05) since they were prepared in the custom-made, dog-bone shaped acrylic mold having precise width. Uniaxial testing was performed with ASTM D882 on a Sintech 2/G Materials Testing System (MTS, Eden Prairie, MN, USA) at an extension rate of 0.5 in/min (12.7 mm/min). Tensile strength, elastic modulus, and toughness were calculated using MTS Testworks 4.0 software.
2.4
Cell culture
hASCs were obtained from an unidentified adult female patient who underwent liposuction in accordance with the protocol approved by the University of Mississippi Medical Center Institutional Review Board (IRB Approval # 2012-0004). hASCs were maintained and expanded at 37 °C and 5% CO 2 in 50:50 Dulbecco’s modified Eagle’s medium (DMEM, HyClone Labs, South Logan, UT, USA) and F12 (Invitrogen, Carlsbad, CA, USA) supplemented with 10% calf serum, 1% penicillin-streptomycin, and 1 mM sodium pyruvate with pH adjusted to 7.4. Cells were harvested using trypsin-EDTA at 80% confluence. These cells were seeded within the collagen and collagen-ELP composites as described below.
2.5
Preparation of composites for cell culture
Four compositions (H1, H2, H3, and H4) were prepared as described above, except 10X DMEM containing 50,000 cells was used instead of 10X PBS. 200 μL of the final solution was incubated per well of a 96-well tissue culture polystyrene plate (Costar, Corning Life Sciences, Lowell, MA, USA) at 37 °C and >70% humidity for 18 h. All composites were prepared in triplicate. After 24-h acclimation, the composites were supplemented with an osteogenic cocktail containing DMEM, 50 mM l -ascorbic acid, 10 nM dexamethasone, 10 mM β-glycerophosphate, 10% fetal bovine serum, and 1% penicillin/streptomycin for 3 weeks. Fresh media was fed every 48 h.
2.6
Biochemical analysis
All the assays were performed according to the manufacturers’ protocols. Cell viability within the various composites was assessed on day 22 using a Live/Dead assay (Invitrogen, Carlsbad, CA, USA). The cells were washed with sterile PBS and Live/Dead reagents (4 μM EthD-1 and 2 μM calcein AM) were added. The composites were incubated for 25 min at 37 °C. Observations were made by epifluorescence microscopy using an Olympus IX 81 microscope (Olympus America, Center Valley, PA, USA) equipped with a Slidebook image analysis software. The percentages of live and dead cells were calculated by measuring the fluorescence at 645 and 530 nm, respectively using a FLX-800 fluorescence plate reader (Biotek, Winooski, VT, USA).
For quantification of total protein content, alkaline phosphatase activity, and osteocalcin production, triplicates of H1, H2, H3, and H4 composites were removed from the plates to isolate the cells. The cells were first separated from the composites by digestion using 1 U/mL Liberase (Roche Diagnostics, Indianapolis, IN, USA) in CaCl 2 —Krebs Ringer buffer for 25 min at 37 °C. The cells were then pelleted from the media via centrifugation at 3500 rpm for 5 min, washed with PBS, and spun again at 3500 rpm for 5 min. The cells were then resuspended in 300 μL of PBS, lysed by sonication at 10% amplitude for 30 s, and immediately frozen.
Total protein amount present in the samples of days 1, 8, 15, and 22 were measured using a BCA total protein assay kit (Thermo Scientific, Rockford, IL, USA). 50 parts of reagent A and 1 part of reagent B supplied by the manufacturer were mixed to form the working solution. 25 μL of the lysate was added to 200 μL of the working reagent, mixed on a shaker for 30 s, and incubated at 37 °C for 20 min. The absorbance was measured at 540 nm on ELX-800 absorbance plate reader (Biotek, Winooski, VT).
A colorimetric kinetic determination of alkaline phosphatase (ALP) activity was performed using QuantiChrom ALP assay kit (BioAssay Systems, Hayward, CA, USA) on days 8, 15, and 22. In brief, 25 μL of the cell lysate was transferred into a 96-well plate along with 150 μL of working solution (10% v/v Magnesium acetate and 4% v/v p -nitrophenyl phosphate liquid substrate in assay buffer) and the absorbance was measured at 405 nm at 0 and 4 min on ELX-800 absorbance plate reader. The ALP activity was then calculated as per manufacturer’s instructions.
A sandwich ELISA assay specific for human osteocalcin (OCN) was performed using osteocalcin assay kit (Invitrogen) on days 8, 15, and 22. In short, monoclonal antibodies bound to the human OCN in standards or samples (25 μL), were bound to a monoclonal antibody labeled with horse radish peroxidase (100 μL) for 2 h at room temperature. The surfaces were washed extensively with a wash solution and reacted to a chromogenic solution in the dark at room temperature for 30 min. The reaction was completed with 100 μL of the stop solution and absorbance was measured at 450 nm using ELX-80 absorbance plate reader. The OCN activity was then calculated as specified by the manufacturer.
Next, the osteogenesis quantitation kit (EMD Millipore, Billerica, MA, USA) was used to analyze mineralization on day 22. The cell-seeded composites were washed with PBS and fixed using 10% formaldehyde for 15 min. After further washing, the composites were incubated at room temperature for 20 min in 1 mL Alizarin red stain per well. The excess dye was removed by extensive washing with DI water. Mineral deposits in composites were stained bright red and visualized using VHX Digital Microscope. Quantitative analysis was performed by further incubating the composites in 10% acetic acid for 30 min with shaking, then vigorous vortexing for 30 s, and heating at 85 °C for 10 min. The extracted mixture was centrifuged at 22,000 × g for 15 min and neutralized with 150 μL of 10% ammonium hydroxide. The absorbance of the solution was measured at 405 nm using ELX-800 absorbance plate reader.
2.7
Scanning electron microscopy (SEM) with energy dispersive spectroscopy (EDS)
The cell seeded composites were washed with sterile PBS and fixed in 4% paraformaldehyde for 1 h at room temperature. They were then dehydrated using ascending ethanol series of 50%, 70%, 80%, 90% and 100% ethanol for 10 min each. The composites were then dried in the desiccator overnight. The dried composites were mounted on aluminum SEM stubs using carbon adhesive tape and sputter-coated with gold for 2 min at 9 V. The composites were observed with SUPRA 40 scanning electron microscope (Carl Zeiss, Thornwood, NY, USA) at 5 kV using both the in-lens detector and the Everhart–Thornley secondary electron detector. Images were recorded at least from two different areas on each composite at 5000× and 10,000× magnifications. Energy dispersive spectroscopy was performed on H3 and H4 composites to determine the presence of phosphorus and calcium.
2.8
Statistical analysis
Results have been reported as mean ± 95% confidence intervals. Results were analyzed using ANOVA and Games-Howell post hoc test for unequal variances in SPSS version 23 statistical software. Values with p ≤ 0.05 were deemed significantly different.
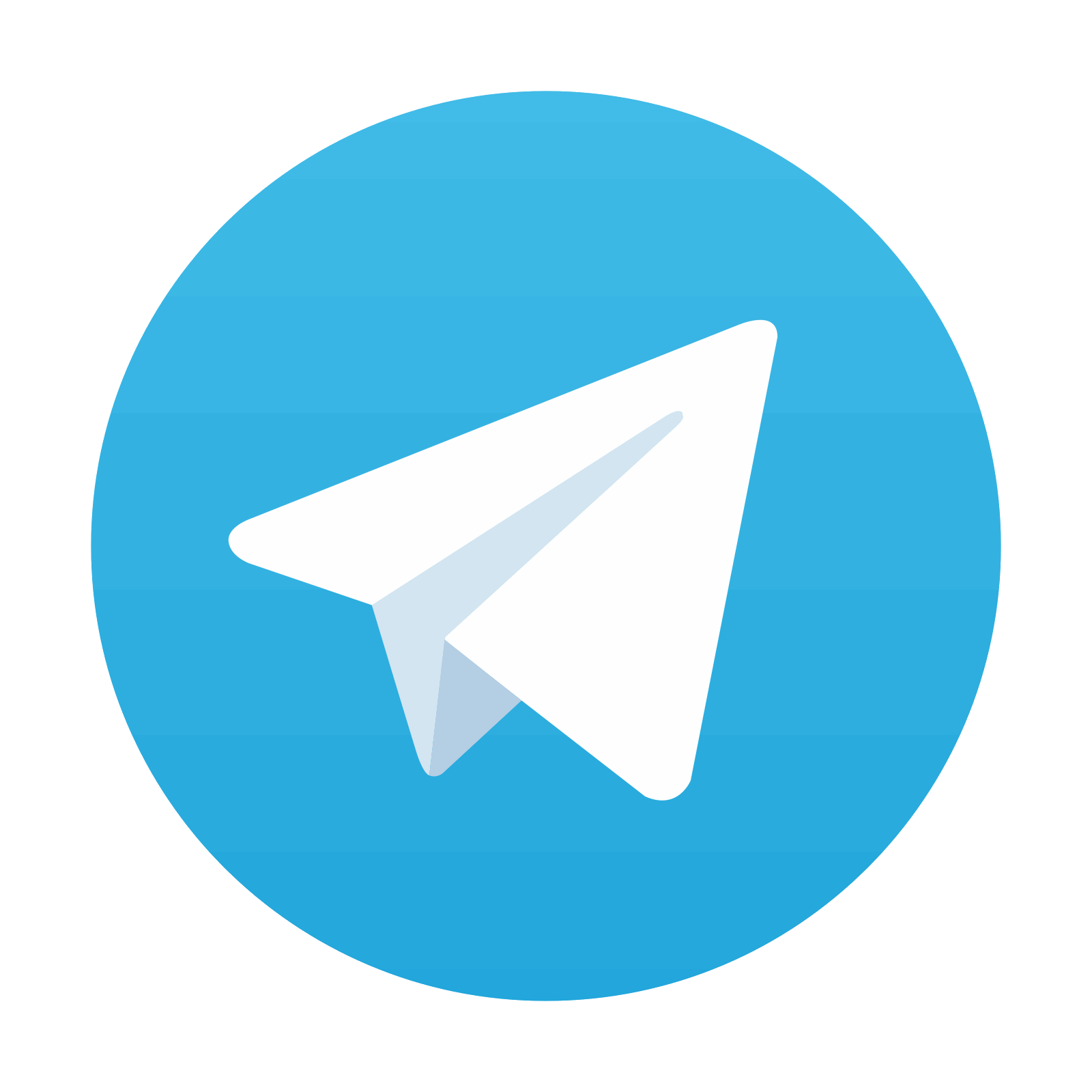
Stay updated, free dental videos. Join our Telegram channel

VIDEdental - Online dental courses
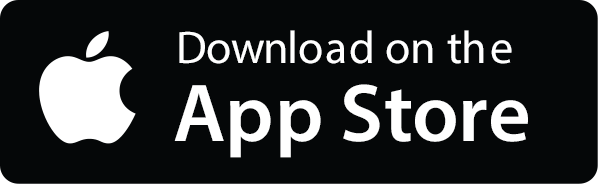
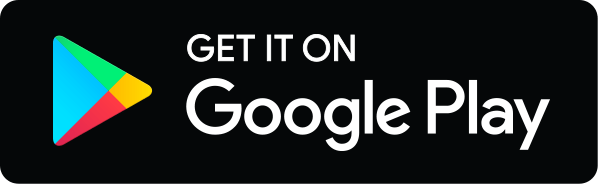