Abstract
The aim of this study was to compare the mechanical stress over hemimandible substrate and hardware after sagittal split ramus osteotomy (SSRO) fixed with five different techniques using three-dimensional (3D) finite element analysis. A 3D finite element model of a hemimandible was created and a 5 mm advancement SSRO was simulated on a computer model. The model was fixed with five different techniques: 3 linear 60° screw arrangement; 3 linear 90° screw arrangement; 3 inverted L screw arrangement; 1 conventional miniplate; and 1 locking miniplate with four monocortical screws. Load was applied until 3 mm displacement was reached and the results were compared with previous mechanical and photoelastic tests, thus analysing the mechanical stresses developed in the proximity of miniplates and screws and within the fixation system itself. The maximum principal stress values demonstrate a lower mechanical stress rate in bone and in the fixation system with the inverted L arrangement, followed by the linear 90° and linear 60° arrangements. The locking miniplate/screw system presented lower maximum principal stress and better stress distribution compared with the conventional system. Under the conditions tested, the reversed L arrangement provided the most favourable stress dissipation behaviour.
The sagittal split ramus osteotomy (SSRO), initially described by Obwegeser and subsequently modified, is now a standard, common and very successful procedure in oral and maxillofacial surgery for the treatment of mandibular discrepancies. Originally patients needed intermaxillary fixation (IMF), as bone stabilization was achieved by means of wire osteosynthesis. Today, stability is achieved by metal plates, screws and combinations of both, in a technique that is referred to as ‘rigid internal fixation’ (RIF).
The introduction of modern devices for internal fixation substantially shortens the duration of IMF or even obviates it. Rigid fixation has become the standard method for controlling the position of the skeletal segments against muscular pull, contraction of soft tissues and gravitational displacement. Miniplates and screws are used to stabilize the proximal and distal segments after osteotomy, to achieve fast bone healing, to avoid postoperative intermaxillary fixation, to initiate early postoperative mandibular function and oral hygiene, and to improve postoperative skeletal stability. Rigid fixation has some disadvantages, such as displacement of the condyle from the fossa and irreversible nerve damage due to the applied compression with miniplates and especially with lag screws.
Relapse after osteotomy is common and an incidence as high as 50% has been reported. Movement at the osteotomy site is the main cause of relapse. According to some authors, the use of miniplates or screws can prevent or at least reduce the incidence of relapse. Different fixation methods including miniplate and lag screws have been compared in numerous studies, some with contradictory results.
Although RIF has been used for more than three decades in orthognathic surgery, since the classic works of Spiessel, there is no international consensus about the ideal rigid fixation technique of choice for SSRO, and many surgeons base their decisions about the fixation methods on their own clinical experience.
Titanium alloy is the most commonly used material in the manufacture of miniplates and screws because of its stiffness, strength and biocompatibility. It is these properties that help to maintain the relative position of the bone segments. RIF procedures have improved the prospects of healthy bone union and lowered the rate of mal-union and non-union.
Many authors have studied the mechanical characteristics and differences between the stabilization techniques for SSRO. Direct experimental measurements, although not impossible, are limited due to a number of factors, such as the amount and type of data points, instrumentation, cost, time, patient availability, and their potentially invasive nature. Most studies used idealized in vitro models. The specific design, model geometry and the type of loading regimens chosen often limit these studies. In most of these studies, it is difficult to understand the subtle mechanical interactions between bone and fixation devices. Generalization of the results to improve the surgical design is difficult.
Several authors have reported the accuracy of finite element analysis (FEA) in describing the biomechanical behaviour of bone specimens. FEA is an analytical system widely applied in engineering and in the aerospace industry, which can be used to solve complex problems in biomechanics. In FEA, a computational model is developed based on the modular principle; it is made from many finite size elements adapted to the real structures. In some given conditions of stress, the displacement and strains of these simple elements can be calculated. Based on the linkage conditions of elements to nodes, the deformation of the overall structure at every node and the variables derived from this as well as the strains can be calculated.
The aim of the present study was to compare the stress values after using five methods of rigid fixation following SSRO advancement surgery. The FEA results are compared and validated with the results of previously published mechanical and photoelastic tests.
Materials and methods
To create the finite element model, it was necessary to build the geometric structures of the hemimandible, the screws and the miniplates. The hemimandible was built from a mandible DICOM file taken from the data bank at CTI (The Renato Archer Center for Information Technology, Campinas, SP, Brazil). The data had been obtained previously from a dry mandible submitted to a helical CT scan with a thickness of 1 mm. The computer models of the titanium miniplates (both conventional and locking) and screws were based on physical specimens from Neoortho (JJGC Ind. e Com. de Mat. Dent. Ltda. – Curitiba, Paraná, Brazil), the same as those used in the work of Sato et al., that was applied to compare the results obtained with FEA.
The SSRO were fixed by means of five RIF techniques that are commonly used in the clinical setting. Technique 1 uses 3 bicortical titanium screws in the inverted L arrangement; technique 2 uses 3 bicortical titanium screws in the 90° linear disposition; technique 3 uses 3 bicortical screws in the 60° linear arrangement; technique 4 uses a conventional 4 hole titanium miniplate with 4 monocortical screws; and technique 5 uses a locking titanium miniplate with 4 monocortical screws ( Fig. 1 ).

Each set of miniplate and screws was determined to be in perfect contact with the bone, with the exception of locking plates, which presented a small misalignment to simulate the clinical application.
The tridimensional geometry of both mandible and miniplate/screws was created by Rhinoceros 4.0 software (McNeel North America, Seattle, WA). At this point, the mandible was separated into two parts to generate a hemimandible due to the symmetry on both sides, bearing just the first molar, to simplify the modelling.
Finite element model development and properties
The geometry was imported to the software Femap v.10.1 (Siemens PLM Software Inc., Plano, TX) for the preprocessing of the finite element (FE) model development. All the materials were considered to be homogenous, isotropic and linear-elastic.
As the results of the FEA would be validated through previous tests, the best choice was to model the bone as one homogenous phase, just like the material used in the mechanical and photoelastic tests (polyurethane and photoelastic resin). Properties from the polyurethane mandibular substratum were obtained by means of a compression test at Instron 4411. Properties for the titanium alloy were provided by the manufacturers, with an isotropic Young’s modulus of 624.42 MPa for the mandibular substratum and 116,000 MPa for the titanium alloy, and Poisson’s ratios of 0.2817 and 0.34, respectively.
A SSRO was simulated according to Epker, with a 5 mm advancement between the proximal and distal segments, as proposed by most orthognathic studies. The two bone fragments were tightly fixed together after the bone was cut, only allowing displacement in the direction of the chewing force.
The mandible was constrained in the temporomandibular joint and posterior ramus area, simulating the mechanical and photoelastic test. Load was applied in the middle of the occlusal face of the first molar and the FEA was done until a 3 mm displacement was reached. The tetrahedral elements and ten nodes of each model are listed in Table 1 .
Model | Elements | Nodes |
---|---|---|
Linear 90° | 91,578 | 138,942 |
Linear 60° | 65,445 | 100,526 |
Inverted L | 129,548 | 194,667 |
Conventional miniplate | 65,550 | 100,446 |
Locking miniplate | 96,410 | 146,673 |
A mechanical structural analysis was carried out using NEi Nastran v.9.2.3 software (NEi Software, Westminister, CA). Stress contours were computed and plotted in the bone tissue and in the fixation appliances. The stability of a three-dimensional (3D) state of stress was evaluated according to the stress hypothesis of ‘maximum principal stress’, the stress scale of which measures the general effective stress in a material. All stress values are given in MPa (N/mm 2 ). A colour scale with 16 stress values served to evaluate the stress distribution quantitatively, varying between −3 to 20 MPa for the hemimandible and −3 to 500 MPa for the miniplates/screws.
The graphic visualization of the results was exported to the FEMAP v.10.1 software. Stress values in the adjacent bone were compared with the fringes from the photoelastic test, taking the pattern and the intensity into consideration. Quantitative analysis was carried out by considering the load performed by the model in the standardized 3 mm displacement.
Results
The results obtained by FEA were compared qualitatively with the photoelastic analysis results from Sato et al. Similar stress patterns between both sample groups were found, which validates the FEA models.
Linear 90° screws arrangement
The highest maximum principal stress of the proximal segment was measured around the superior and inferior screws, with minimum stress distribution in the middle screw. In the upper screw, the stress is higher in the medullar region than in the cortical region, with the stress dissipating to the superior and posterior region, an area with good bone thickness (oblique line of the mandible). Meanwhile, around the lower screw the stress dissipates to the superior and anterior regions. When analysing the distal segment, stress was higher in the lower screw, medium in the middle and lower in the upper screw. When the whole set is considered, the lower screw is the one submitted to the higher stress rates, as seen in the photoelastic test. The stress in the screws showed that the higher stress value was in the middle of the lower screw ( Fig. 2 ).
Linear 60° screws arrangement
The maximum principal stress in the proximal segment demonstrates that the higher stress was in the lower screw, and the other screws barely presented any stress rate. The same stress distribution pattern occurs in the distal segment. Concerning the 60° arrangement, the stress was more concentrated in the lower screw, when compared to the 90° arrangement. Maximum principal stress in the screws was distributed in the middle of the screws, with higher stress when compared with the 90° arrangement, where the lower screw concentrated the highest stress amount ( Fig. 3 ).
Inverted L arrangement
The higher maximum principal stress in the proximal segment was concentrated in the upper and lower screws in the distal segment, whereas in the proximal segment the concentration was much higher in the upper screw, followed by the inferior one, and with minimum stress in the medium screw. The stress seems to be much more homogeneous in this group when compared with other bicortical screw fixation techniques. The stress zones in the proximal segment dissipated to areas with higher bone thickness (oblique line of the mandible), providing the fixation system with more resistance. Very little stress was concentrated in the anterior region of the osteotomy, which could generate a fragility zone; but stress was found in the interior of the bone segments, in the direction of the mandibular canal. The stress in the screws showed that highest stress value was in the middle of the upper screw, followed by the lower one. The middle screw presented virtually minimum stress ( Fig. 4 ).
Conventional miniplate
The highest maximum principal stress in the proximal segment was around the anterior screw, with stress dissipating to the anterior and superior regions, in the direction of the osteotomy. The distal screw of the proximal segment had the lowest stress rates of all groups, with no stress in the bone around the screw. In the distal segment, some stress was found near the screws, but with lesser intensity than the anterior screw of the proximal segment. Stress in the screw near the osteotomy of the distal segment dissipates to the inferior and posterior directions, while the stress of the other screw in this segment was distributed to the superior and anterior course. Concerning the titanium miniplate and screw complex, the stress was concentrated mainly in the lower part of the miniplate from the gap to both screws in the distal segment. Stress was also detectable in the upper part of the miniplate near the first screw of the proximal segment, near the osteotomy, but with less intensity. The screw near the osteotomy in the proximal segment was the one submitted to the highest stress in the system, followed by the screw near the osteotomy of the distal segment ( Fig. 5 ).
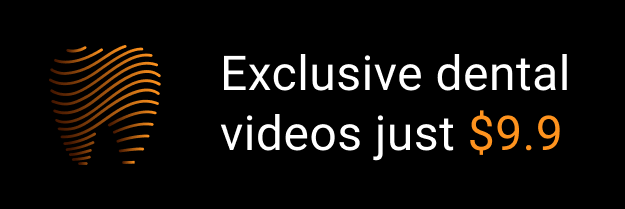